Bacterial and Fungal Infections
Robert L. Schelonka
Bishara J. Freij
George H. McCracken Jr.
Infections cause significant mortality and long-term morbidity in neonates, especially for premature infants of very low birth weight (1,2,3,4,5,6). Temporal and geographic differences in the relative frequencies of various neonatal pathogens are well recognized (7,8,9,10,11). In North America in the 1930s and 1940s, gram-positive cocci such as group A β-hemolytic streptococci and Staphylococcus aureus were the most common bacterial isolates from neonates with sepsis, with Escherichia coli accounting for most of the remaining cases. S. aureus and E. coli became the major pathogens in the 1950s, but since the late 1960s, group B β-hemolytic streptococci and E. coli have predominated. Coagulase-negative staphylococci emerged in the 1980s and have surpassed S. aureus and gram-negative enteric bacilli as the bacteria most frequently associated with nosocomial infections in many neonatal intensive care units (NICUs), and several Candida species have increased in frequency to become major neonatal pathogens in the 1990s. This has largely been a consequence of the survival of very-low-birth-weight (VLBW) infants who require lengthy hospitalizations and considerable mechanical and nutritional support (12,13). Since the 1990s there has been an emerging problem of multiple-drug-resistant gram-positive and gram-negative enteric bacilli in NICUs (14,15,16,17).
The outcome of neonatal infections may be improved if illness is recognized early and appropriate antimicrobial agents are administered promptly. This chapter presents pertinent epidemiologic and pathogenetic concepts of specific infections, clinical manifestations, and diagnostic evaluations of patients with these diseases, with a rational approach to therapy of neonatal infections.
EPIDEMIOLOGY
The two principal sources of newborn infection are the mother and the nursery environment. Infection is acquired from the mother transplacentally, at the time of delivery, or in the postnatal period. The infant may acquire infection postnatally from environmental sources, such as nursery personnel, respiratory equipment, sinks, contaminated total parenteral nutrition solutions or medication vials, and incubators. Infections manifesting within the first week of life are usually the result of exposure to microorganisms of maternal origin, but infections presenting later can have a maternal or environmental source.
Myriad aerobic and anaerobic bacteria, mycoplasmas, chlamydiae, fungi, viruses, and protozoa can be found in the maternal genital tract. Some of these organisms pose little threat to the newborn infant (e.g., Lactobacillus, a-hemolytic streptococci, Veillonella), and others are infrequent causes of neonatal disease (e.g., Streptococcus pneumoniae, Neisseria meningitidis) (18,19,20). More commonly, organisms such as groups A and B γ-hemolytic streptococci, E. coli, Listeria monocytogenes, Haemophilus influenzae, Neisseria gonorrhoeae, cytomegalovirus, and herpes simplex virus are responsible for serious neonatal infections (3,4).
Within a few days after birth, a-hemolytic streptococci, S. epidermidis, and gram-negative enteric bacilli colonize the throat, nose, umbilicus, and stool (21,22,23). The gastrointestinal (GI) tract of newborns becomes heavily colonized by lactobacilli. Infants in NICUs tend to have delayed colonization, which probably is related to early antimicrobial therapy for possible sepsis, and are more likely to acquire nosocomial strains of gram-negative bacilli such as Klebsiella, Enterobacter, Citrobacter, and E. coli (24,25,26). Colonization of the scalp, axilla, and groin by coagulase-negative staphylococci is universal by 48 hours of age (12). In a prospective study of 18 premature infants admitted to a NICU, S. epidermidis as the only coagulase-negative staphylococcal species isolated from the axilla, ear, nasopharynx, and rectum was found in about 11% of infants during their first postnatal day; this increased to 100% by 4 weeks of age. None of these infants had a
predominant S. epidermidis biotype on the first day compared with 89% by 4 weeks of age. The prevalence of slime production and multi-drug resistance among isolates rose from 68% to 95% and from 32% to 82%, respectively, during the 4-week study period (27).
predominant S. epidermidis biotype on the first day compared with 89% by 4 weeks of age. The prevalence of slime production and multi-drug resistance among isolates rose from 68% to 95% and from 32% to 82%, respectively, during the 4-week study period (27).
Postnatal fungal colonization is more likely to occur in low-birth-weight (LBW) infants. An estimated 10% of term infants have GI Candida colonization within the first 5 days of life; infants weighing less than 1,500 g have colonization rates of about 60% (28). Early colonization (less than 2 weeks of age) is more common, involves the GI and respiratory tracts, and is with Candida albicans or C. tropicalis, unlike late colonization (≥2 weeks of age), which usually involves the skin and is more likely to be with C. parapsilosis (29). C. albicans colonization of infants is usually of maternal origin, whereas C. parapsilosis colonization is acquired from exogenous sources such as the hands of nursery personnel (30). Cutaneous colonization with Malassezia (Pityrosporum) furfur, a lipophilic yeast best known as the cause of tinea versicolor, is common and is found in as many as two-thirds of all critically ill newborns; fewer than 3% of healthy newborns and young infants have skin colonization by this fungus (31,32,33). The use of water-in-oil emollient creams (e.g., Eucerin Creme) as a moisturizer for premature infants does not appear to alter colonization patterns by bacteria or fungi (34), but in a randomized controlled trial of topical emollient cream there was an 5% absolute increased risk (25.8% vs. 20.4%; RR: 1.26; 95% CI: 1.02,1.54) of acquiring nosocomial bacterial sepsis. This increase in infection rates due mostly to an increase in the number of coagulase-negative staphylococci infection (35). The vagina or cervix of asymptomatic, sexually active women is colonized by Ureaplasma urealyticum in 40% to 80% and by Mycoplasma hominis in 21% to 53%. Vertical transmission rates from 45% to 66% for preterm and term neonates have been reported for U. urealyticum (36). By 3 months of age, about 33% to 68% of these infants continue to have detectable pharyngeal, ocular, or vaginal colonization (37). Vertical transmission rates for infants born to women with cervical Chlamydia trachomatis infections have been estimated at 40% to 70%; about 35% of untreated infants continue to be infected at one or more sites (e.g., conjunctiva, nasopharynx, oropharynx, rectum, vagina) at 12 months of age (38,39,40).
In the United States, bacterial sepsis affects up to 32,000 infants annually, and the incidence of neonatal sepsis is from 1 to 8 cases per 1,000 live births (41,42,43). The average national incidence rate for nursery-acquired infections is about 1.4%, but figures reported for NICUs are considerably higher and range from 5% to 30% (3,44,45,46,47). The most important risk factors for acquiring a nosocomial infection are low birth weight and gestational age; others include prolonged hospitalization, invasive procedures, placement of indwelling devices such as central venous catheters or ventriculoperitoneal shunts, bacterial or fungal colonization, and overcrowded nurseries.
PHARMACOLOGIC BASIS OF ANTIMICROBIAL THERAPY
Selection of antimicrobial therapy for neonatal infections must be based on pharmacokinetic properties of antibiotics in newborn infants of different gestational and postnatal ages, antimicrobial susceptibilities of commonly encountered pathogens within each nursery, and the natural history of the infectious disease being treated (48).
Combining two or more antibiotics is the usual clinical practice when initiating therapy for presumed systemic bacterial disease (e.g., ampicillin and an aminoglycoside are combined to treat suspected early onset septicemia or meningitis before identification of the pathogen). After a bacterium has been identified and its susceptibility to various antimicrobial agents is determined, a single appropriate antibiotic usually is satisfactory for treating most infections.
Although antibiotics are used commonly to prevent infection, they are effective prophylactically only if directed against a single pathogen. For example, a single dose of penicillin G given intramuscularly at birth reduces the colonization rate and incidence of early- onset group B streptococcal (GBS) disease, except in infants who acquire the infection in utero (49). However, if antibiotics are used as broad-spectrum coverage against many potential pathogens, they rarely are effective. This umbrella method of chemoprophylaxis encourages the emergence of resistant strains among previously susceptible bacteria and alters the normal flora of the GI and respiratory tracts with overgrowth of potentially virulent organisms. Broad-coverage prophylaxis may partially suppress a bacterium, masking the development of clinical disease and causing neglect of important surgical measures or serious delay in administering more effective therapy.
SEPSIS NEONATORUM
The term sepsis neonatorum describes a disease of infants who are younger than 1 month of age, are clinically ill, and have positive blood cultures. The presence of clinical manifestations differentiates this condition from the transient bacteremia observed in some healthy neonates.
The bacteria responsible for neonatal sepsis vary geographically. GBS and E. coli predominate in the United States, whereas S. aureus and gram-negative bacilli are much more common in developing countries (8). The bacterial etiology of sepsis also varies by the postnatal age of the infant. In a study of a cohort of 6,956 VLBW infants (401 to 1,500 g) admitted to 15 medical centers in the United States during a 32-month period between 1998 and 2000, the incidence of early- onset sepsis (occurring during the first 72 hours of life) was 1.5% and that of late-onset sepsis (occurring after 3 days of age) was 25%. The nature of the pathogens associated with the early-onset and late-onset infections differed markedly, with E. coli predominating (44% of cases) in the former and coagulase-negative staphylococci (48% of cases) in the latter (Table 47-1) (3,4).
TABLE 47-1 DISTRIBUTION OF PATHOGENS IN A COHORT OF 5,447 (EARLY-ONSET) AND 6,956 (LATE-ONSET) VERY-LOW-BIRTH-WEIGHT INFANTS (401-1,500 G) IN THE UNITED STATES FROM SEPTEMBER 1,1998 TO AUGUST 1, 2000. | |||||||||||||||||||||||||||||||||||||||||||||||||||||||||||||||
---|---|---|---|---|---|---|---|---|---|---|---|---|---|---|---|---|---|---|---|---|---|---|---|---|---|---|---|---|---|---|---|---|---|---|---|---|---|---|---|---|---|---|---|---|---|---|---|---|---|---|---|---|---|---|---|---|---|---|---|---|---|---|---|
|
Pathogenesis
Maternal, environmental, and host factors determine which infants exposed to a potentially pathogenic organism will develop sepsis, meningitis, or other serious invasive infections.
Many prepartum and intrapartum obstetric complications have been associated with increased risk of infection in the newborn, the most significant of which are premature onset of labor, prolonged rupture of fetal membranes, chorioamnionitis, and maternal fever. In one study of 963 pregnancies complicated by premature rupture of membranes, the incidence of clinical sepsis increased from 2% among infants born within 23 hours of membrane rupture to 7% and 11% among those delivered 24 to 47 hours and 48 to 71 hours after rupture, respectively. The risk was highest for the premature, LBW infants (50). The incidence of infection has been estimated at 8.7% for infants born to mothers with prolonged rupture of membranes (≥24 hours) and clinical chorioamnionitis (51). Intraamniotic infection is associated with a higher incidence of sepsis among infants weighing less than 2,500 g at birth compared with those weighing 2,500 g or more (16% and 4%, respectively). Death from sepsis is greater in the LBW group (11% and 0%, respectively) (52). Isolation of bacteria from the chorioamnion has been associated with an increased risk of neonatal death among preterm infants (53). Maternal urinary tract infections, low parity, and the use of internal monitoring devices are risk factors for chorioamnionitis (54). Concurrent maternal and neonatal bacteremia has been documented for many microorganisms (55,56,57).
With improved supportive care of the sick neonate has come increased opportunity for microorganisms of relatively low virulence to cause systemic disease. The use of arterial and venous umbilical catheters, central venous catheters, indwelling urinary catheters, and endotracheal tubes provides access to the debilitated infant for organisms in the respiratory, GI, or genitourinary tract, on the skin, or in respiratory support equipment.
Bacterial colonization of the skin and mucosal surfaces precedes invasive disease in most infants with sepsis. Type III strains of GBS, the ones most commonly associated with early-onset septicemia and with meningitis at any age in early infancy, adhere better to vaginal and neonatal buccal epithelial cells in vitro than do other GBS strains (58,59). Bloodstream invasion generally follows local multiplication of the organism at sites of colonization. Aspiration of infected amniotic fluid is another proposed route for fetal infection among infants born to mothers with chorioamnionitis.
Animals have been used to define the host-bacteria interactions that determine pathogenesis of disease. Bloodstream infection in infant rats or mice caused by E. coli K1 or any of the GBS serotypes can be prevented by pretreatment with type-specific capsular polysaccharide antibody (60,61). The orogastric route for E. coli K1 in the infant rat and the intratracheal installation of GBS in the
rhesus monkey or rat produce illnesses that closely parallel the human syndromes (62,63,64).
rhesus monkey or rat produce illnesses that closely parallel the human syndromes (62,63,64).
Several investigations of the host-parasite association of humans with GBS have focused on measurement of specific antibody in the serum of infected and colonized persons. Protective concentrations of antibody to GBS serotype III were found in 73% of women whose newborn infants were well but in only 17% of women whose neonates developed sepsis or meningitis caused by this organism (65). The amount of antibody to GBS serotype III was considerably lower in ill infants than in healthy neonates born to mothers with vaginal colonization (65). Levels of GBS serotype III antibodies correlate with in vitro opsonic activity and in vivo protection of animals experimentally infected with these strains (66,67). The administration of standard intravenous immunoglobulin preparations with activities against GBS, of human GBS monoclonal antibodies, or of GBS hyperimmune polyclonal antibodies provides significant protection to animals experimentally infected with this pathogen (68,69). Asymptomatic colonization also is associated with antibody formation (70,71). Although less extensively studied, similar observations have been made for other GBS serotypes (69,72,73,74,75).
Evidence suggests that antibodies to the K1 E. coli antigen are protective against infection by this organism, but this is not firmly established (76). Neonatal serum has been shown to be inefficient in killing E. coli because of a deficiency of nonimmunoglobulin G serum components, such as complement factor 9 (77,78).
Physiologic deficiencies of the classic and alternative pathways of complement activation in neonates contribute to inefficient bacterial opsonization (79,80). Organisms such as GBS and E. coli that have high capsular sialic acid content tend to be poor activators of the alternative complement pathway (81).
Fibronectin is a multifunctional glycoprotein found in the plasma and on the surface of certain epithelial cells, basement membranes, and connective tissues. In plasma, fibronectin acts as a nonspecific opsonin that enhances clearance of invading bacteria (82). Fibronectin is deficient in neonatal plasma, and its concentration varies inversely with gestational age (82,83). Septic infants have been shown to have significantly lower plasma concentrations of this glycoprotein than healthy, age-matched controls (84). The soluble form of fibronectin binds poorly to GBS (85). Fibronectin enhances phagocyte function in vitro and in vivo (82).
Quantitative and qualitative deficiencies in neonatal neutrophils contribute to the immaturity of the immune system of newborns. The abnormalities become most pronounced at times of stress or during infections and include impaired chemotaxis, decreased deformability, reduced C3bi receptor expression, depressed bacterial killing by phagocytes, and oxidative metabolic abnormalities. The neutrophil storage pool of neonates is markedly depleted compared with that of adults (86). Stem cell proliferative rates are at near maximal capacity and cannot increase appreciably in response to infection (87).
Clinical Manifestations
Most infants with septicemia present with nonspecific signs that are usually observed first by the nurse or mother rather than by the physician. The most common of these vague signs are temperature instability, lethargy, apnea, and poor feeding (88). Although hypothermia is more common, a temperature elevation above 37.8°°C is significant in the neonate and frequently associated with bacterial infections, especially with temperatures higher than 39°C (89). The clinical signs in some infants may suggest respiratory or GI disease (e.g., tachypnea and cyanosis or vomiting, diarrhea, and abdominal distention). Septicemia must always be included in the differential diagnosis when evaluating an infant with these findings (88).
Clinical manifestations of sepsis in very-low-birth weight infants (501-1,500 g) include increasing apnea (55%), feeding intolerance, abdominal distension, or heme-positive stools (43%), increased respiratory support (29%), and lethargy and hypotonia (23%). Abnormal white blood cell count, unexplained metabolic acidosis, and hyperglycemia are noted in 46%, 11%, and 10%, respectively (47).
Although it is tempting to recommend an evaluation for septicemia in all infants with nonspecific clinical manifestations, this is impractical and unnecessary in many cases. A complete history and physical examination, coupled with clinical experience, are the best guides to determine the extent of the evaluation. If septicemia cannot be reasonably excluded on clinical grounds, a blood culture should be obtained and empiric antibiotics administered. Hepatosplenomegaly, jaundice, and petechiae are classic signs of neonatal infection but represent late manifestations.
Streptococcal Disease
In the 1970s, Group B γ-hemolytic streptococci infections emerged a leading infectious cause of morbidity and mortality in the United States (90,91). By the 1980s, GBS were the most common gram-positive bacteria isolated from blood of infants with septicemia in North America (92). Although control measures such as intrapartum antibiotic therapy which were in widespread practice in the 1990s have reduced the incidence of early onset Group B strepto-coccal (GBS) disease, GBS remains an important cause of neonatal sepsis. GBS accounts for 10% to 40% of sepsis cases occurring in the first week of life (3,93,94).
In the mid 1980s, clinical trials demonstrated that administering intrapartum intravenous antibiotics could prevent early onset neonatal GBS sepsis (95). In response to these findings, the American Academy of Pediatrics in 1992 provided guidelines recommending intrapartum antibiotic prophylaxis (IAP) of selected GBS carriers (96). In 1996, with additional data and the collaborative efforts of clinicians, researchers, professional organizations and the public health community, the Centers for Disease Control and Prevention (CDC) issued consensus guidelines recommending IAP to prevent GBS maternal-infant transmission
(97,98). The 1996 national guidelines recommended that women colonized with GBS at 35 weeks or more of gestation or women with intrapartum risk factors (delivery <37 weeks gestation, intrapartum temperature ≥100.4°°F or rupture of membranes ≥18 h) should be offered intrapartum chemoprophylaxis. Additionally, women having a previous infant with invasive GBS disease or women with GBS bacteriuria during pregnancy should also be offered intrapartum chemoprophylaxis. Because of the routine implementation of IAP, the incidence of early onset neonatal infections decreased by 65 percent, from 1.7 per 1000 live births in 1993 to 0.6 per 1000 in 1998 (99). With the decline in early onset GBS disease, there has been a concomitant rise in E. coli and ampicillin-resistant E. coli sepsis in very low birth weight and preterm infants (4). The CDC and the American College of Obstetricians and Gynecologists have recommended universal screening at 35 or more weeks of gestation and IAP for GBS-colonized women (100,101).
(97,98). The 1996 national guidelines recommended that women colonized with GBS at 35 weeks or more of gestation or women with intrapartum risk factors (delivery <37 weeks gestation, intrapartum temperature ≥100.4°°F or rupture of membranes ≥18 h) should be offered intrapartum chemoprophylaxis. Additionally, women having a previous infant with invasive GBS disease or women with GBS bacteriuria during pregnancy should also be offered intrapartum chemoprophylaxis. Because of the routine implementation of IAP, the incidence of early onset neonatal infections decreased by 65 percent, from 1.7 per 1000 live births in 1993 to 0.6 per 1000 in 1998 (99). With the decline in early onset GBS disease, there has been a concomitant rise in E. coli and ampicillin-resistant E. coli sepsis in very low birth weight and preterm infants (4). The CDC and the American College of Obstetricians and Gynecologists have recommended universal screening at 35 or more weeks of gestation and IAP for GBS-colonized women (100,101).
The epidemiology, pathogenesis, and clinical features of GBS disease have been defined (102). The organism is a common inhabitant of the female genital tract and can be isolated from vaginal and anorectal cultures of as many as 35% of asymptomatic pregnant women (102,103,104,105). Risk factors for maternal GBS colonization include lower parity, higher frequency of intercourse, multiple sexual partners, and concurrent colonization with Candida spp (104). Peripartum GB S colonization of the lower urogenital tract has been associated with several maternal complications including preterm labor, premature rupture of membranes, endometritis, chorioamnionitis, urinary tract infection, intrapartum or postpartum fever, late abortions, and invasive infections, such as bacteremia or meningitis (57,106). The identical serotype can be isolated frequently from urethral cultures of the sexual partners of these culture-positive women (107). Although most infected pregnant women have normal, healthy infants, 1% to 2% of pregnancies involving maternal infection result in stillbirths or infants with neonatal disease. Vertical transmission of group B Streptococci occurs in approximately 50% to 70% of mother-infant pairs, resulting in neonatal colonization rates of from 8% to 25%. Transmission is most likely to occur among infants born to heavily colonized mothers (108). The early-onset GBS syndrome occurs within the first 72 hours of life (mean age of onset 20 hours), and 65% of reported cases involve premature infants. There is often a history of other maternal obstetric complications (Table 47-2) (106). Onset is sudden and follows a fulminant course, with the primary focus of inflammation in the lungs, although meningitis can develop. Respiratory distress is the most common initial sign among infants with early onset meningitis (109). Apnea, hypotension, and disseminated intravascular coagulation cause rapid deterioration and often lead to the patient’s demise within 24 hours. It is difficult to identify the infant with respiratory distress caused by GBS infection, because in 60% of infected patients, the chest radiograph shows a reticulogranular pattern with air bronchograms indistinguishable from that seen with uncomplicated hyaline membrane disease. The mortality rate is approximately 6% and is inversely correlated with birth weight (110,111). All five GBS serotypes have been incriminated in early-onset disease in roughly similar proportions. A similar syndrome has been associated with groups D and G streptococci (112,113).
TABLE 47-2 Risk factors for early-onset group B streptococcal disease | ||
---|---|---|
|
A late-onset syndrome caused by GBS or L. monocytogenes occurs most frequently at 2 to 4 weeks of age, but it may be seen as late as 16 weeks. The onset is insidious; poor feeding and fever are the most frequent presenting signs. A fulminant illness with rapid onset and progressive deterioration occasionally is encountered (114). Meningitis is seen in approximately 60% of infants with the late-onset syndrome, and GBS serotype III accounts for about 95% of these cases (102). Rarely, infants with late-onset meningitis caused by GBS present with hydrocephalus. These infants may appear to have uncomplicated hydrocephalus with normal lumbar cerebrospinal fluid (CSF). Examination of ventricular fluid reveals pleocytosis, and the organism is recovered on culture. Spinal fluid cultures of infants with meningitis caused by gram-positive organisms usually are sterile within 24 to 36 hours of therapy, and the mortality rate is 10% to 15%.
Approximately 20% of neonatal infections caused by GBS do not fit into the early- or late-onset syndromes and extend over a broad clinical spectrum involving many different organ systems. Several manifestations have been observed (102,115,116):
Cellulitis
Scalp abscess
Impetigo
Fasciitis
Breast abscess
Adenitis
Supraglottitis
Conjunctivitis
Orbital cellulitis
Ethmoiditis
Otitis media
Pneumonia complicated by empyema
Myocarditis
Endocarditis
Hepatitis
Septic arthritis
Osteomyelitis
Bursitis
Urinary tract infection
Omphalitis
Peritonitis
Asymptomatic transient bacteremia.
The transient bacteremia is remarkable because these infants appear clinically well and are cultured because of a history of maternal obstetric complications. A repeat blood culture before the institution of antibiotic therapy frequently is sterile.
Both relapses and reinfections can occur after invasive GBS infections (102). Reasons for relapse may include an inadequate penicillin dose, a short duration of therapy of the initial episode, or unrecognized foci of infection (e.g., endocarditis, brain abscess). Reinfection can occur because of maternal GBS mastitis (117). Rifampin (20 mg/kg/d for 4-7 days) has been used to eradicate GBS carriage in infants with recurrent disease and is given after completion of systemic penicillin therapy (117).
Coagulase-Positive Staphylococcal Disease
The phage group I S. aureus, which was common in the late 1950s, still exists in some nurseries and occasionally causes serious systemic neonatal disease. The pathogenicity of this organism is based on its ability to invade the skin and musculoskeletal system, producing furuncles, breast abscesses, adenitis, and osteomyelitis. Septicemia usually is secondary to local invasion. After S. aureus is recovered from blood cultures of neonates, a careful search should be made for a primary focus. Some group I S. aureus strains produce toxic shock syndrome toxin-1, formerly known as enterotoxin F or pyrogenic toxin C, and have caused toxic shock syndrome in older neonates (118,119). Clinical characteristics of this disease include the sudden onset of fever, diarrhea, shock, mucous membrane hyperemia, and a diffuse erythematous macular rash with subsequent desquamation of the hands and feet, commencing on about the fifth or sixth day of illness.
In the early 1970s, phage group II coagulase-positive staphylococci emerged as a common cause of neonatal infection. Although this organism may be invasive, pathogenicity depends principally on production of exotoxins (e.g., exfolatiative or epidermolytic toxins A and B). Common areas of primary infection include the umbilical stump, conjunctiva, and throat; infection of a surgical wound has been described (120). The exfoliative toxins act on the zona granulosa of the epidermis and cause epidermal splitting through activity of the toxins on the desmosomes (121). Clinical disease may take one of several forms, including bullous impetigo, toxic epidermal necrolysis (Ritter disease), and nonstreptococcal scarlatina. Collectively, these diseases have been referred to as the expanded scalded skin syndrome (Fig. 47-1) (122).
The initial finding in Ritter disease is generalized erythema associated with edema and tenderness on palpation, usually noticed between days 3 and 16 of life (123). After several days, a distinctive desquamation of large sheets of epidermis occurs, which is different from the fine desquamation observed in the second and third weeks of streptococcal scarlet fever. Large flaccid bullae commonly observed in Ritter disease will, on rupture, leave a tender, weeping erythematous base. Some infants may appear quite toxic with the generalized form of disease. A rare, congenital form of staphylococcal scalded skin syndrome has been described (124). Spread within a nursery can occur, and its recognition warrants the prompt institution of infection control measures to limit the spread of the toxigenic strain of S. aureus (125).
Umbilical venous or arterial catheters and central venous lines are well-recognized risk factors for staphylococcal bacteremia. S. aureus is second only to coagulase-negative staphylococci as a cause of catheter-related infections (126).
The mortality rate for neonates with S. aureus bacteremia is about 20%. LBW infants are at highest risk of death from this infection (127).
Coagulase-Negative Staphylococcal Disease
The isolation of coagulase-negative staphylococci from blood, CSF, or urine of newborns with clinical signs of sepsis
can be significant, and these bacteria should not be dismissed as contaminants. Of the at least 30 recognized coagulase-negative staphylococcal species, S. epidermidis is clinically the most significant for neonates (128,129,130). Experience indicates that these bacteria are responsible for about 10% to 27% of all cases of sepsis in NICUs, but can account for as many as 48% of late-onset sepsis cases in VLBW infants (3,92) Coagulase-negative staphylococcal infections are nosocomial in origin and result in substantially longer hospitalizations for affected infants (131,132). Risk of infection with these organisms increases with decreasing gestational age and birth weight (133,134,135,136).
can be significant, and these bacteria should not be dismissed as contaminants. Of the at least 30 recognized coagulase-negative staphylococcal species, S. epidermidis is clinically the most significant for neonates (128,129,130). Experience indicates that these bacteria are responsible for about 10% to 27% of all cases of sepsis in NICUs, but can account for as many as 48% of late-onset sepsis cases in VLBW infants (3,92) Coagulase-negative staphylococcal infections are nosocomial in origin and result in substantially longer hospitalizations for affected infants (131,132). Risk of infection with these organisms increases with decreasing gestational age and birth weight (133,134,135,136).
Clinical manifestations of coagulase-negative staphylococcal infections are similar to those caused by other pathogens and include apnea, bradycardia, temperature instability (e.g., hypothermia, hyperthermia), respiratory distress (e.g., tachypnea, retractions, cyanosis), GI manifestations (e.g., poor feeding, abdominal distention, bloody stools), lethargy, and metabolic acidosis (12,129). Clinical illnesses include septicemia, meningitis with or without CSF abnormalities, necrotizing enterocolitis, pneumonia, omphalitis, soft tissue abscesses associated with persistent bacteremia, endocarditis, and scalp abscesses and osteomyelitis at insertion sites of fetal monitoring electrodes (133,134,135,136,137,138,139,140,141,142,143,144).
Risk factors for coagulase-negative staphylococcal infections include the presence of foreign bodies, such as central venous lines, ventriculoperitoneal shunts, or peritoneal dialysis catheters, prior antibiotic therapy, and intravenous infusion of lipid emulsions for nutritional support (129, 145,146). The organisms travel along catheter tracks from colonization sites. They eventually adhere to, and proliferate on, certain biosynthetic materials and later cause local or systemic reactions. The mechanism by which adherence occurs appears initially to involve hydrophobic and electrostatic interactions between the bacteria and the biopolymers (129). Once attached, a viscous exopolysaccharide referred to as slime is formed. Slime covers the bacteria to form a surface biofilm that protects them from such environmental factors as antibiotics and host defenses although allowing continued access to nutrition (129). The density of the biofilm may be increased if the organisms are exposed to subinhibitory concentrations of antibiotics to which they are susceptible (e.g., vancomycin) (147). Slime appears to inhibit neutrophil chemotaxis and phagocytosis and the lymphoproliferative responses of mononuclear cells to mitogens (148,149). Slime-producing strains account for most coagulase-negative staphylococci isolated from infants with invasive infections (135). Ineffective opsonophagocytosis as a result of intrinsic deficiencies in neonatal host defenses contributes to the increased susceptibility of LBW infants to infection with these organisms (150,151).
Coagulase-negative staphylococci produce a variety of toxins that may serve as virulence factors, including hemolysins, proteases, urease, and fibrinolysin (12). Most fecal isolates produce a hemolysin that functionally and immunologically is identical to the delta toxin produced by S. aureus (152). This toxin causes severe mucosal necrosis and hemorrhage when injected into ligated infant rat bowel loops and may play a role in the pathogenesis of neonatal necrotizing enterocolitis (153,154).
Listeria monocytogenes Disease
The incidence of perinatal listeriosis decreased by about 51% between 1989 and 1993, from 17.4 to 8.6 per 100,000 persons younger than 1 year of age (155,156). This reduction was related temporally with industry, regulatory, and educational efforts to aggressively enforce food monitoring policies.
The pathogenesis of clinical diseases caused by L. monocytogenes is similar to those caused by GBS. A fulminant, disseminated disease (granulomatosis infantiseptica) may occur during the first several days of life. The pathogen is acquired transplacentally or by aspiration at the time of vaginal delivery, and multiple organ systems (e.g., liver, spleen, kidneys, lungs, brain, and skin) are involved; 35% to 55% of infants die of their infection (157,158). The infant frequently presents with hypothermia, lethargy, and poor feeding. Early passage of meconium in a premature infant suggests Listeria infection (159). A characteristic rash consisting of small, salmon-colored papules scattered primarily on the trunk can be observed in some infants. The chest roentgenogram shows parenchymal infiltrates suggestive of aspiration pneumonitis in most infants. A miliary-type of bronchopneumonia can be seen in some cases. Listeria serotypes Ia, Ib, and IVb produce the early onset disease, whereas serotype IVb is the predominant type in late-onset meningitic disease (160).
A delayed form of neonatal listeriosis occurs during the second through fifth weeks of life and primarily involves the meninges (160,161). The infected infant usually is the full-term product of an uncomplicated labor and delivery. Onset of symptoms and signs is relatively insidious and indistinguishable from those observed with meningitis caused by other pathogens. The source of the organism in late-onset disease is unclear. Although acquisition of Listeria may occur during passage through an infected birth canal, it appears that most cases result from postpartum horizontal spread. In favor of the latter route of infection are several reported clusters of neonatal listeriosis and the demonstration of cross-infections between newborns using enzyme electrophoretic typing and DNA fingerprinting (157,162,163,164).
The peripheral leukocyte count usually shows a brisk leukocytosis, with a predominance of polymorphonuclear leukocytes in the differential count (165). A significant elevation in the number of monocytes, to 7% to 21% of the total leukocyte count, has been documented on admission laboratory evaluation of infected infants. A monocytosis of this magnitude can be demonstrated in most remaining infants on repetitive testing of the peripheral leukocyte count, but monocytes typically are not found in the spinal fluid of infants infected with L. monocytogenes. Polymorphonuclear leukocytes predominate in about 75% of cases, with a relative lymphocytosis in the
remaining 25%. In contrast, adults with Listeria meningoencephalitis may have CSF monocytosis of 80% to 90% (158). As with other pyogenic meningitides, hypoglycorrhachia and elevated protein concentrations are frequent findings. Examination of the stained smear of spinal fluid has not been rewarding in more than 50% of cases. This is a reflection of the relatively low concentrations of organisms in the fluid. The bacteriology laboratory should be forewarned of the clinical suspicion of Listeria meningitis, because these microorganisms occasionally are discarded as contaminants because of their tinctorial and morphologic similarities with diphtheroids. Overnight refrigeration of spinal fluid specimens frequently enhances growth of this organism.
remaining 25%. In contrast, adults with Listeria meningoencephalitis may have CSF monocytosis of 80% to 90% (158). As with other pyogenic meningitides, hypoglycorrhachia and elevated protein concentrations are frequent findings. Examination of the stained smear of spinal fluid has not been rewarding in more than 50% of cases. This is a reflection of the relatively low concentrations of organisms in the fluid. The bacteriology laboratory should be forewarned of the clinical suspicion of Listeria meningitis, because these microorganisms occasionally are discarded as contaminants because of their tinctorial and morphologic similarities with diphtheroids. Overnight refrigeration of spinal fluid specimens frequently enhances growth of this organism.
Enterococcal Infection
Group D streptococci were formerly divided into enterococcal and nonenterococcal types. The enterococci are now included in the new genus Enterococcus; most neonatal infections are caused by Enterococcus faecalis and, to a lesser extent, by E. faecium (166,167,168).
Early onset enterococcal disease (less than 7 days of age) is relatively mild and presents clinically with respiratory distress or diarrhea. No associations with underlying conditions, invasive procedures, or maternal obstetric complications have been observed (169). Late-onset disease (≥7 days of age) most commonly afflicts low-birth weight infants with complicated clinical problems that often require surgical procedures (e.g., bowel resection), central venous catheters, or prior treatment with antimicrobials. Clinical manifestations of late-onset disease include apnea, bradycardia, circulatory failure, meningitis, pneumonia, scalp abscesses, catheter-related bacteremia, and necrotizing enterocolitis-associated septicemia (169). About 20% of enterococcal blood isolates represent skin contamination; clinical correlation is needed to differentiate contamination from true infection (167).
Enterococci can spread rapidly within a nursery (170). Nosocomial outbreaks of enterococcal septicemia in NICUs are well documented (168,171). The mortality rate is estimated at 6% to 11%, but it can be as high as 17% in infants with necrotizing enterocolitis-associated septicemia (167,169).
The importance of identifying Enterococcus as the etiologic agent in septicemia primarily relates to selection of proper antimicrobial agents. The enterococci are moderately resistant to penicillin alone, as a result of certain properties of their penicillin-binding proteins (167,172). Some enterococcal strains have acquired new mechanisms of antibiotic resistance that include γ-lactamase production and high-level aminoglycoside resistance (minimal inhibitory concentrations [MIC] ≥2,000 μg/mL) (170,173,174). Strains resistant to the glycopeptides vancomycin and teicoplanin (glycopeptide-resistant enterococci) were first described in 1987 and since have emerged as major nosocomial pathogens (175,176,177,178,179). Nonenterococcal group D streptococci continue to be susceptible to penicillin (167).
Gram-Negative Bacterial Infection
In North America, E. coli is the most common gram-negative organism causing septicemia during the neonatal period. Klebsiella and Enterobacter strains are second (3,92). In contradistinction to illness caused by GBS and L. monocytogenes, E. coli infections do not fit into distinct clinical syndromes of early- and late-onset disease. Approximately 40% of E. coli strains causing septicemia possess K1 capsular antigen, and strains identical with those isolated from blood cultures usually can be identified in the patient’s nasopharynx or rectal cultures. The clinical features of E. coli sepsis generally are similar to those observed in infants with disease caused by other pathogens. Respiratory distress is noted in about 73% with E. coli sepsis occurring during the first week of life (180). Localized E. coli infections have included breast abscess, cellulitis, pneumonia, lung abscess, empyema, osteomyelitis, septic arthritis, urinary tract infection, ascending cholangitis, and otitis media.
An increase in the proportion of neonatal E. coli sepsis cases caused by ampicillin-resistant strains has been noted by several investigators (3,180,181) This shift occurred as maternal intrapartum prophylaxis for the prevention of early onset GBS sepsis was being implemented more widely by obstetricians at these medical centers. Most deaths were seen in neonates infected with ampicillin-resistant E. coli strains.
Pseudomonas septicemia may present with a characteristic violaceous papular lesion or lesions that, after several days, develop central necrosis. Although these skin lesions most commonly are seen in Pseudomonas infection, theymay be associated with other organisms (182). Pseudomonas typically is encountered in late-onset sepsis, although occasional newborns with an early-onset form of this infection have been reported.
Neonatal infections caused by H. influenzae biotype IV have increased in frequency in the last 20 years and currently account for about 8% of early-onset sepsis in very LBW infants (401-1,500 g) (3,183). These strains are nontypeable, have a distinct multilocus enzyme genotype revealed by clonal analysis, express peritrichous fimbriae and a variant P6 outer membrane protein, and have a characteristic outer membrane electrophoretic profile. Genetic analysis suggests that these strains represent a cryptic genospecies only distantly related to H. influenzae or Haemophilus haemolyticus (183,184).
Anaerobic Infections
Anaerobes account for up to 25% of all bacteria isolated from blood cultures of neonates with suspected septicemia in various studies (185,186,187,188). A recent retrospective review of neonatal bacterial infections suggests that the incidence of anaerobic isolates is about 1% (189). Bacteroides spp, primarily Bacteroides fragilis, and clostridia are most commonly recovered (187). Peptostreptococcus spp, Veillonella spp, Propionibacteriurn acnes, Eubacteriurn spp, and Fusobacterium spp occur relatively infrequently. Anaerobes are found
mixed in cultures with aerobic bacteria in about one-third of cases. Clinical illnesses include transient bacteremia, fulminant septicemia, postoperative infections, and intrauterine death associated with septic abortion (185). Localized diseases such as omphalitis, cellulitis, and necrotizing fasciitis are seen commonly with clostridia (190). Conditions predisposing to anaerobic septicemia include prolonged rupture of membranes, chorioamnionitis, prematurity, and GI disease.
mixed in cultures with aerobic bacteria in about one-third of cases. Clinical illnesses include transient bacteremia, fulminant septicemia, postoperative infections, and intrauterine death associated with septic abortion (185). Localized diseases such as omphalitis, cellulitis, and necrotizing fasciitis are seen commonly with clostridia (190). Conditions predisposing to anaerobic septicemia include prolonged rupture of membranes, chorioamnionitis, prematurity, and GI disease.
Anaerobes isolated from blood within the first 2 days of life are usually gram-positive and penicillin G-susceptible, but those isolated from older newborns tend to be gram-negative and penicillin G-resistant (186). Gram-positive anaerobes are more likely to be recovered from blood of infants with sepsis associated with chorioamnionitis, whereas gram-negative anaerobes predominate in necrotizing enterocolitis-associated bacteremia (186). The mortality rates for reported cases of anaerobic septicemia are about 35% for illnesses caused by Bacteroides spp and 12% for other anaerobes (187).
Genital Mycoplasmas
M. hominis and U. urealyticum occasionally are isolated from the blood of neonates with sepsis. Cassell and colleagues (36) observed concomitant bacteremia in 40% and 26% of preterm infants with positive endotracheal cultures for M. hominis and U. urealyticum, respectively. Other investigators were unable to recover genital mycoplasmas from the blood or CSF of neonates with suspected sepsis (191- 192). An association between persistent pulmonary hypertension and U. urealyticum sepsis and pneumonia has been observed (193).
Fungal Infections
Fungal sepsis occurs in as many as 12% of LBW infants (3). Most cases are as a result of Candida spp, particularly C. albicans (194,195,196). C. tropicalis, C. parapsilosis, C. lusitaniae, and C. glabrata also cause systemic candidiasis (196). Congenital candidiasis is uncommon and results from ascending infection through intact membranes. It usually manifests with early skin lesions (e.g., maculopapular rash, pustules, vesicles, desquamation, skin abscesses), but disseminated and life-threatening infections can occur (197,198).
The incidence of candidemia in NICUs is increasing steadily. In one such unit in Norfolk, Virginia, the rate of candidemia increased over 11-fold in the 15-year period between 1981 and 1995. Additionally, C. parapsilosis was responsible for 60% of all candidemias during the last 5 years of the study period (199).
Risk factors for late-onset systemic candidiasis include prematurity, low birth weight, use of broad-spectrum antimicrobial agents, H2 blocker therapy, steroid therapy, central vascular catheters, parenteral hyperalimentation, intralipid infusions, prolonged endotracheal intubation, necrotizing enterocolitis, and immunologic immaturity (200,201,202). The most important of these factors appears to be the number of prior antibiotics and the duration of therapy (203,204). LBW infants in whom mucocutaneous candidiasis develops are at considerable risk of subsequent invasive disease (203,204,205). Infants with invasive candidiasis caused by C. albicans are more likely to have antecedent candidal thrush or perineal dermatitis and to die of their infection than neonates with severe C. parapsilosis infections (199,206). Clinical manifestations vary and are indistinguishable from those caused by other pathogens. A fungal cause for sepsis should be considered strongly in an infant weighing less than 1,500 g who has been hospitalized for a prolonged period, is receiving parenteral hyperalimentation through a central vascular catheter, and previously has been treated with multiple antibiotics; a history of GI disease or surgery adds to the clinical suspicion. Diseases caused by Candida spp include, but are not limited to, pneumonia, endocarditis, endophthalmitis, meningitis, cerebral abscesses, pyelonephritis, renal mycetoma (fungal balls), peritonitis, hepatosplenic abscesses, arthritis, and osteomyelitis (194,207,208,209,210,211,212,213,214,215).
Malassezia furfur causes invasive neonatal fungal disease. Most infections involve chronically ill premature infants who are receiving lipid emulsions through a central venous catheter (216). The most commonly reported presenting signs include fever (50%) and respiratory distress (50%). Pathologic analysis reveals mycotic thrombi around catheter tips, endocardial vegetations, and lung lesions that include mycotic emboli with occlusion of pulmonary arteries, septic thrombi, pulmonary vasculitis, and alveolitis (216). Involvement of other organs is uncommon. Malassezia pachydermatis is a recently recognized human pathogen that has been incriminated in NICU outbreaks of bloodstream, urinary tract, and central nervous system infections. In one reported outbreak, the source of M. pachydermatis was the contaminated hands of health care workers who were colonized from their pet dogs at home (217). Systemic infections as a result of other fungi such as Torulopsis glabrata, Hansen ula anomala, Aspergillus spp, Cryptococcus neoformans, Coccidioides immitis, Blastomyces spp, and Trichosporon beigelii are rare (218,219,220,221).
Laboratory Tests and Findings
Since the early 1970s, several screening tests and scoring systems have been described that are purported to aid the physician in making the diagnosis of neonatal infection. Although a few are helpful in identifying the infant at high risk of developing infection, the diagnosis of septicemia can be made only by recovery of the organism from blood cultures or other normally sterile body fluids (221,222). It is imperative that these cultures be obtained by strict aseptic technique. Blood should be obtained from a peripheral vein rather than from the umbilical vessels, the outer several millimeters of which are contaminated frequently with bacteria. Femoral vein aspiration may result in cultures contaminated with coliform organisms from the perineum. Heelstick samples have low sensitivities. The skin
overlying the vein to be punctured should be cleansed with an antiseptic solution, such as an iodophor, and allowed to dry for maximal antiseptic effect. The amount of blood drawn is critical; 1 to 2 mL of blood is required for optimal results (223). The sensitivity of a single blood culture in identifying septicemia is only 80% (222). Obtaining blood cultures from multiple sites may enhance the yield and aid in identifying false-positive results (224). Quantitative blood cultures, if available, are helpful in differentiating true pathogens from culture contaminants (225,226). Routine blood culture methods are sufficient for the detection of Candida septicemia (227); however, if M. furfur is a suspected pathogen, the microbiology laboratory should be alerted so that blood can be inoculated onto special media that provide for the organism’s absolute nutritional requirement for medium-chain fatty acids (196).
overlying the vein to be punctured should be cleansed with an antiseptic solution, such as an iodophor, and allowed to dry for maximal antiseptic effect. The amount of blood drawn is critical; 1 to 2 mL of blood is required for optimal results (223). The sensitivity of a single blood culture in identifying septicemia is only 80% (222). Obtaining blood cultures from multiple sites may enhance the yield and aid in identifying false-positive results (224). Quantitative blood cultures, if available, are helpful in differentiating true pathogens from culture contaminants (225,226). Routine blood culture methods are sufficient for the detection of Candida septicemia (227); however, if M. furfur is a suspected pathogen, the microbiology laboratory should be alerted so that blood can be inoculated onto special media that provide for the organism’s absolute nutritional requirement for medium-chain fatty acids (196).
It frequently is helpful to obtain cultures of other sites before initiating antimicrobial therapy. For example, percutaneous bladder aspiration of urine for culture can be helpful in identifying the urinary tract as the focus of infection. This is particularly true for illness occurring after the third postnatal day. Nasopharyngeal, skin, umbilical cord, gastric, and rectal cultures frequently are positive in the early septicemic form of listeriosis and GBS disease. However, these colonization sites are not predictive of the cause of bloodstream infection and should not be used to guide antimicrobial therapy (228). All clinically stable infants with suspected septicemia should have CSF obtained for examination and culture before therapy. This practice has been challenged for infants younger than 7 days of age because of its low yield; the yield from a lumbar puncture is much higher when performed on infants older than 1 week of age (229,230,231,232,233,234,235). Practitioners opting to forego performing a lumbar puncture for infants with suspected early onset sepsis should anticipate that, on occasion, the diagnosis of meningitis will be delayed or missed because not all newborns with bacterial meningitis have positive blood culture results (234,236).
The peripheral leukocyte count is the most frequently used indirect indicator of bacterial infection. After correction for the nucleated erythrocyte count, the total absolute neutrophil count and the ratio of immature to total neutrophilic forms are compared with normal standard values for age. In the absence of maternal hypertension, severe asphyxia, periventricular hemorrhage, maternal fever, or hemolytic disease, absolute total neutropenia and an elevated ratio of immature to total neutrophilic forms suggest bacterial infection (237,238). Infants born in high-altitude areas have higher total and immature neutrophil counts (239). Wide interreader differences in band neutrophil identification have been observed, thereby limiting the utility of the immature to total neutrophil ratio in actual clinical practice (240). Repeating complete blood counts within 24 hours of birth may enhance the value of the test as a screen for sepsis high-risk infants (239). In the absence of clinical signs of sepsis, leukocyte values are unlikely to rule out infection. In a prospective, observational study of 856 near-term or term neonates without clinical signs of sepsis who were exposed to maternal chorioamnionitis, 99% had at least 1 abnormal neutrophil value based on published reference values (237,238,239,240,241,242). Single or serial neutrophil values did not assist in the diagnosis of early onset infection or determination of duration of antibiotic therapy in the at risk but culture-negative infants (243).
Gastric aspirate stains and culture, erythrocyte sedimentation rate, C-reactive protein (CRP), and the nitroblue tetrazolium test have not proved useful as indicators of bacterial infection, although in combination these tests may offer some guidance (244). CRP values appear not to be influenced by perinatal asphyxia, hyperbilirubinemia, periventricular hemorrhage, or respiratory distress syndrome (245). Serial CRP measurements may be helpful in identifying infants not likely to be infected and in whom antibiotics can be safely stopped (246). The detection of interleukin-6 (IL-6) in serum, especially in conjunction with an elevated CRP or procalcitonin measurement, may be useful in the early diagnosis of neonatal infection (243,244,245,246,247,248,249,250,251,252). The levels of granulocyte colony-stimulating factor, neutrophil CD11b expression, circulating intracellular adhesion molecule-1, interleukin-1 receptor antagonist (IL-1ra), serum procalcitonin, and interleukin-8 are all elevated in newborns with sepsis, but how these laboratory findings can best be utilized for the diagnosis of neonatal sepsis is yet to be defined (247,249,250,253,254). In one study, IL-1ra and IL-6 levels were found to be elevated (a 15-fold median maximal increase) 2 days before a diagnosis of sepsis was made (250).
Detection of the soluble antigens of E. coli Kl, GBS, H. influenzae type b, N. meningitidis, and S. pneumoniae by latex particle agglutination (LPA) is useful for identifying the infant infected with these pathogens. The absence of antigen does not rule out infection by these organisms. Substantial sensitivity differences have been found among the commercially available LPA assays for GBS antigen (255). False-positive LPA test results for GBS in urine specimens can result from contamination of bag specimens with these bacteria from perineal and rectal colonization, cross-reacting antigens, or absorption of antigen from the GI tract (256,257). Similar false-positive test results may be obtained for the other bacteria. Rapid diagnosis of invasive Candida infection by detection of its circulating cell wall (e.g., mannan), cytoplasmic (e.g., enolase), or heat-labile antigens is possible, although the reliability of these tests is yet to be proved (258).
Therapy
After septicemia is suspected, suitable cultures should be obtained and therapy with ampicillin and an aminoglycoside started immediately. If meningitis has been excluded, ampicillin is administered intravenously or intramuscularly in individual doses of 50 mg/kg given every 12 hours, or 8 hours for infants weighing <2,000 g or $2,000 g, respectively. The frequency is increased 3 or 4 times daily for infants 1 to 4 weeks of age, depending on their birth weight. The selection of the aminoglycoside
antibiotic should be based on antimicrobial susceptibilities of enteric organisms isolated from infants in each nursery. Gentamicin is the drug of choice for treatment of infections caused by susceptible gram-negative organisms and is administered intravenously or intramuscularly in a dosage of 4 to 5 mg/kg at 24 to 48 hour intervals depending on the infant’s postmenstrual and postnatal age (259).
antibiotic should be based on antimicrobial susceptibilities of enteric organisms isolated from infants in each nursery. Gentamicin is the drug of choice for treatment of infections caused by susceptible gram-negative organisms and is administered intravenously or intramuscularly in a dosage of 4 to 5 mg/kg at 24 to 48 hour intervals depending on the infant’s postmenstrual and postnatal age (259).
Aminoglycoside-resistant E. coli have been encountered in some nurseries in North America. In these nurseries or in an infant from whom an isolate is shown to be resistant to kanamycin or gentamicin, amikacin or cefotaxime should be used. Studies have demonstrated no significant ototoxicity in infants and children who were treated in the neonatal period with kanamycin or gentamicin (48). However, in premature infants and in those receiving these drugs for prolonged periods, brainstem evoked response audiometry should be performed whenever possible. Serum concentrations of the aminoglycosides should be monitored in LBW premature infants because of erratic absorption and elimination of the drugs in these infants. Although cefotaxime should not be used routinely for initial empiric therapy of neonatal sepsis, it is an effective agent when used alone or combined with an aminoglycoside for infections caused by coliform bacilli.
When the type of skin lesions or historic experience suggests the possibility of Pseudomonas infection, ceftazidime, piperacillin or ticarcillin with or without an aminoglycoside is the therapy of choice. Although not approved for use in neonates by the Food and Drug Administration, we have successfully used ticarcillin-clavulanate and piperacillin-tazobactam for treatment of sepsis caused by multiresistant gram-negative enteric bacilli, Pseudomonas, and anaerobic bacteria.
If S. aureus sepsis is suspected but not proved, parenteral methicillin or nafcillin should be substituted for penicillin or ampicillin, because approximately 80% of these staphylococci will be penicillin-resistant. Although gentamicin and kanamycin possess activity against most staphylococci, these agents cannot be recommended because there are no studies of their efficacy in neonatal staphylococcal disease. For disease caused by coagulase negative staphylococci or multiresistant S. aureus strains, vancomycin is the preferred therapy. Peak and trough serum vancomycin concentrations should be monitored because of the drug’s narrow therapeutic index.
After the pathogen is identified and its antimicrobial susceptibilities are known, the most appropriate drug or drugs should be selected. As a general rule, gentamicin alone or in combination with ampicillin, or cefotaxime alone or in combination with an aminoglycoside, should be used for susceptible E. coli, Klebsiella spp, and Enterobacter spp; amikacin alone or in combination with cefotaxime for gentamicin-resistant coliform bacteria; ceftazidime, piperacillin, ticarcillin, pipercillin-tazobactam, or ticarcillin-clavulanate, with or without an aminoglycoside, for Pseudomonas; ampicillin alone or in combination with an aminoglycoside for P. mirabilis, enterococci, and L. monocytogenes; and penicillin for other gram-positive organisms, except for penicillin-resistant S. aureus, for which methicillin or nafcillin is the drug of choice. Vancomycin is used for coagulase-negative staphylococci and MRSA. Rarely, coagulase-negative staphylococcal strains that are resistant to vancomycin can emerge during treatment with this agent (260). S. aureus strains with reduced susceptibility to vancomycin (glycopeptide-intermediate S. aureus [GISA]) have been isolated from a few patients with a variety of infections. The first such clinical isolate was described in a 4-month-old Japanese infant with a nosocomial surgical site infection (261,262). No GISA strains have been identified in newborns to date. Treatment of GISA-related infections generally requires the use of drugs such as linezolid. The MIC and minimal bactericidal concentration (MBC) of penicillin and ampicillin should be determined for GBS because a small percentage of these organisms are tolerant (i.e., have an MBC to MIC ratio greater than 32) to these antibiotics (263). These strains are best treated with a penicillin-aminoglycoside combination. Penicillin resistant and vancomycin-tolerant pneumococci have been reported in newborn infants in France but not yet in North America (264). The therapeutic options for vancomycin-resistant enterococcal infections in neonates are seriously limited. Some strains may be susceptible to a combination of penicillin or ampicillin and an aminoglycoside. Chloram-phenicol or tetracyclines may be effective against some strains, but these drugs have serious toxicities for neonates. Nitrofurantoin is active against many vancomycin-resistant enterococcal strains and has been used to treat enterococcal urinary tract infections in adults. Dalfopristin-quinupristin is approved for use in adults, but there are no data on its use in infants. Several investigational ketolides, oxazolidinones, glycylcyclines, and even newer semisynthetic glycopeptides with in vitro activities against vancomycin-resistant enterococci are being evaluated (265). U urealyticum infections are treated with erythromycin (266).
Three classes of antifungals are commonly used in the treatment of systemic fungal infections in neonates: the polyene macrolides (amphotericin B [deoxycholate and lipid preparations]); the azoles (fluconazole); and the fluorinated pyrimidines (flucytosine). The drug of choice for systemic fungal infections is amphotericin B, with or without flucytosine (194,267). The half-life and serum concentrations of amphotericin B are highly variable during the neonatal period (268). The drug appears to be better tolerated by infants than older children and adults, but renal and hepatic functions should be monitored carefully (194,269). The optimal daily dosage of amphotericin B is not universally agreed on. The most commonly used dosage regimen is to begin with 0.5 mg/kg of the drug on the first day and, if tolerated, to increase the daily dosage to 1.0 mg/kg by the second or third day of treatment. The cumulative dosage of amphotericin B needed for the adequate treatment of systemic Candida infection is not well defined, but it is estimated to be 20 to 30 mg/kg (194). Resistance to amphotericin B among Candida species is not a major clinical problem (270). Amphotericin B frequently is combined with flucytosine for the treatment of central nervous system fungal infection because of flucytosine’s
excellent CSF penetration and the in vitro synergy of this drug combination against Candida. GI intolerance, myelosuppression, and hepatotoxicity are common side effects of flucytosine (194).
excellent CSF penetration and the in vitro synergy of this drug combination against Candida. GI intolerance, myelosuppression, and hepatotoxicity are common side effects of flucytosine (194).
Experience with the use of liposomal amphotericin B preparations in newborns is growing (271,272,273,274,275,276). Linder and colleagues (275) compared the effectiveness and tolerability of three antifungal preparations, amphotericin B, liposomal amphotericin B (LamB) and amphotericin B colloidal dispersion (ABCD), for the treatment of 52 neonates with Candida bloodstream infection. Sterilization of the blood was achieved with amphotericin B in 68% of patients, LamB in 83% and ABCD in 57%, when used as monotherapy; with the addition of a second antifungal agent, success rates were 100%, 83.3%, and 92.8%, respectively. There were no differences between the groups in the time to resolution of fungemia. No patients had immediate local or systemic adverse events and none showed deterioration in renal function. Juster-Reicher and colleagues (276) evaluated high-dose (5-7 mg/kg/day) liposomal amphotericin B in 37 very low birth weight infants with systemic candidiasis. Candidiasis was as a result of C. parapsilosis in 17 cases, and C. albicans in 15 cases. Fungal eradication was achieved in (95%) episodes; median duration of therapy until fungal eradication was 8.76 4.5 days. The authors concluded that high-dose liposomal amphotericin B was effective and safe in the treatment of neonatal candidiasis. Additionally, fungal eradication was more rapid in patients treated early with high doses and in patients who received high-dose liposomal amphotericin B as first-line therapy. At present, lipid formulations of amphotericin B do not appear to be more efficacious than conventional amphotericin B deoxycholate, and their use should be limited to patients who are either refractory to, or intolerant of, the regular amphotericin B preparation (277).
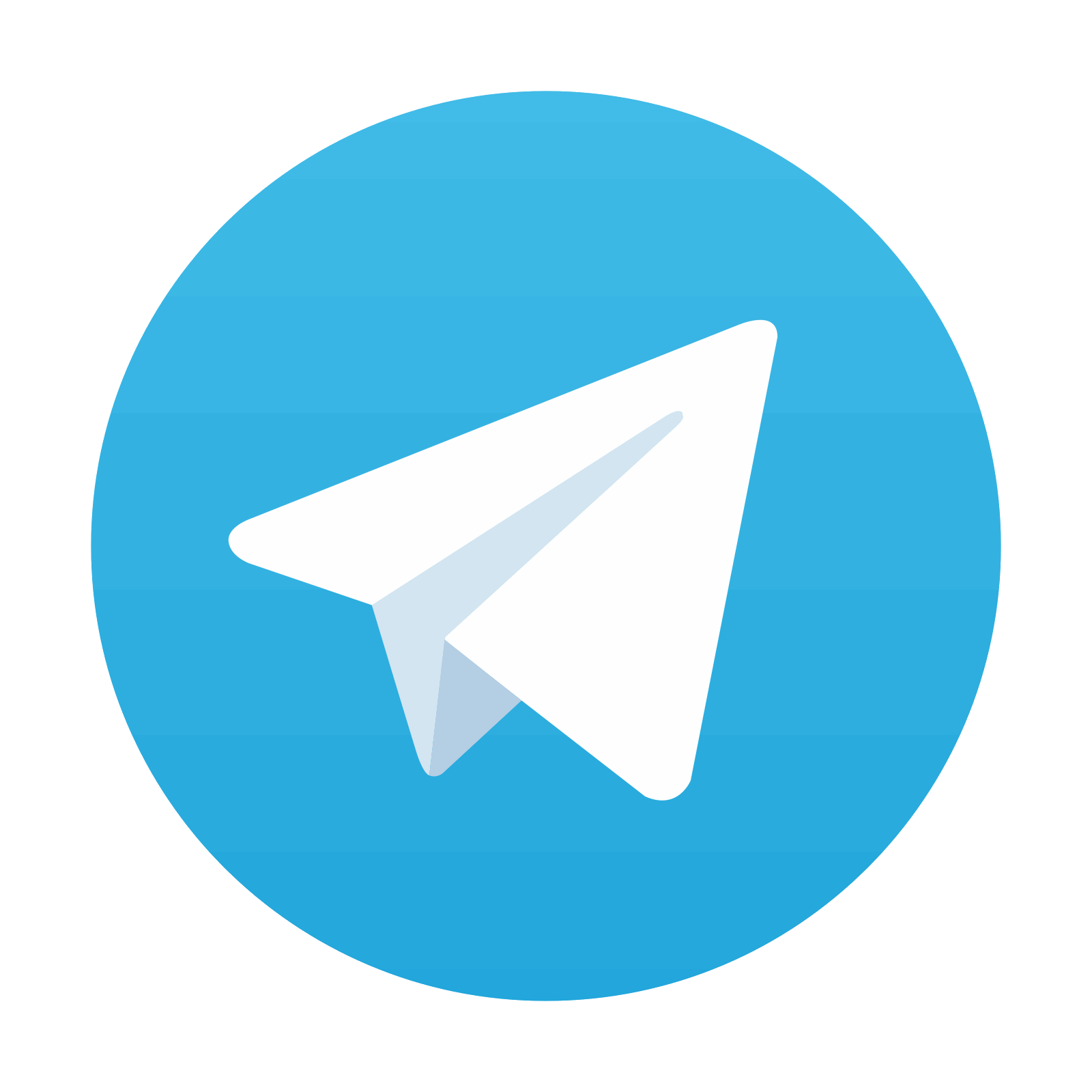
Stay updated, free articles. Join our Telegram channel

Full access? Get Clinical Tree
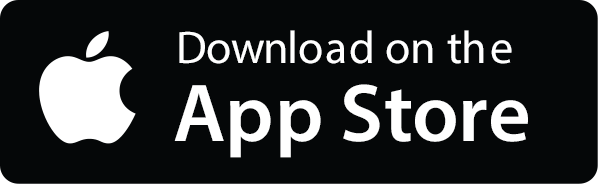
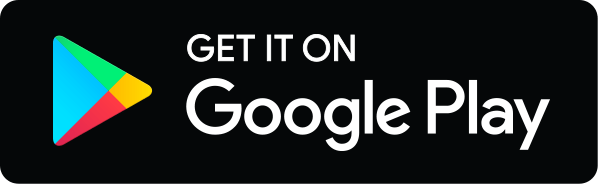