- •
The settings during conventional ventilation and oxygen supplementation require frequent adjustments to adapt to the changing needs of the preterm infant.
- •
Because of the premature infant’s respiratory instability and resource limitations, a balance between adequate respiratory support and the risk of adverse side effects is not always achieved.
- •
Automated modalities that adjust the respiratory support and oxygen supplementation to the infant’s needs have been developed to address these limitations.
- •
Studies evaluating these modalities show promising short-term results, but further research is needed to determine whether these systems can improve longer-term respiratory, ophthalmic, and neurodevelopmental outcomes.
A high proportion of premature infants have respiratory failure and require mechanical respiratory support and supplemental oxygen. These therapies are associated with increased risk for lung and eye injury, particularly when they are used for prolonged periods. Excessive oxygen supplementation is also associated with greater risk for oxidative damage to the central nervous system and other organs.
During neonatal intensive care, caregivers need to make frequent adjustments in respiratory support based on monitored parameters of ventilation and oxygenation to minimize the risk of adverse effects. Despite best efforts, the respiratory support often exceeds what is actually needed by the infant or at times may be insufficient. This is due to the intrinsic respiratory instability of the preterm infant, to the intermittent nature of monitoring, and to staff limitations that do not allow minute-to-minute adjustment of the respiratory support to meet the infant’s needs.
To address these limitations, newer forms of neonatal respiratory support have been developed to automatically adapt respiratory support to the changing needs of the infant. The most promising of these approaches will be described in this chapter with emphasis on the available evidence and possible limitations.
Automation in Mechanical Ventilation
Despite the increasing use of noninvasive respiratory support, a considerable proportion of very preterm infants require intubation and mechanical ventilation after birth. As the initial respiratory failure improves, two divergent patterns are commonly observed. In some infants, the weaning process proceeds rapidly, but in others weaning is difficult and they continue mechanical respiratory support for long periods.
In addition to the severity of underlying lung disease, factors that prolong the need for respiratory support in preterm infants include immaturity of the respiratory control system, instability of lung volume, and unfavorable lung and chest wall mechanics. These conditions increase the respiratory instability, which combined with inconsistent weaning strategies and staff limitations, can lead to prolonged ventilation and increased risk for lung injury.
Standard modes of neonatal ventilation, such as intermittent mandatory ventilation (IMV), synchronized IMV (SIMV), and assist/control (A/C) ventilation, use inflation pressure as the primary control variable and are referred to as pressure-controlled (PC) modes. They provide a constant level of ventilatory support whereby the peak inflation pressure (PIP), ventilator cycling frequency, or both are constant. These modes require manual adjustments to adapt to the changing needs of the infants, but many times these are not frequent enough to maintain stable gas exchange. During the weaning process, the ventilator frequency and/or PIP are reduced and spontaneous inspiratory effort plays a greater role in maintaining ventilation. However, ventilation can be affected by an inconsistent respiratory drive and acute changes in lung mechanics. To overcome the limitations of intermittent manual adjustment of ventilator settings, automated modes have been proposed as means to continuously titrate respiratory support to the infant’s changing needs.
Volume-Targeted Ventilation
Volume-targeted ventilation (VTV) includes modes of PC ventilation in which the peak inflation pressure or inflation time is automatically adjusted from one cycle to the next or within the cycle to maintain the tidal volume ( V T ) close to a target level. Available modalities of VTV differ in whether V T adjustment is based on the volume delivered to the patient during inspiration, the exhaled volume, or the volume delivered by the ventilator to the circuit rather than the patient.
In volume guarantee (VG) ventilation PIP is adjusted from one cycle to the next to maintain a target V T . PIP of the subsequent cycle is adjusted based on the difference between the exhaled V T of the previous cycle and the target V T . VG targets the exhaled V T to circumvent the overestimation of delivered V T resulting from gas leaks around the endotracheal tube (ETT) during the inspiratory phase. PIP is automatically adjusted within a range set by the clinician. VG ventilation can be used in combination with A/C ventilation, PSV, SIMV, and IMV.
In pressure-regulated–volume-control (PRVC) ventilation an initial diagnostic cycle determines the respiratory system compliance and PIP is subsequently adjusted from cycle to cycle based on the difference between the inspiratory V T and the target. The V T is measured at the ventilator end of the patient circuit, resulting in overestimation of the V T delivered to the patient. Use of the compliance compensation feature to estimate the infant’s inspiratory V T after compensation for the volume compressed in the circuit is available but may be unreliable in the presence of ETT leaks. The measured V T during inspiration may be overestimated in the presence of leaks around the ETT. The newest version of PRVC now measures the V T at the airway opening, which should provide more accurate estimation of delivered V T . PRVC is exclusively an A/C mode.
In volume controlled (VC) ventilation the set volume is delivered into the ventilator circuit and the inspiratory phase ends when this volume is delivered. Pressure rises and reaches its peak just before exhalation. In newborn infants, the volume delivered to the circuit is much larger than V T delivered to the infant because of compression of gas within the circuit and humidifier and possible loss of gas owing to ETT leak. VC can be used in combination with A/C, SIMV, or IMV.
The automatic adjustment of PIP in VTV maintains a more stable V T , prevents excessive lung inflation, and leads to a more stable gas exchange in the face of changing lung mechanics and spontaneous respiratory effort. Fig. 19.1 shows typical adjustments in peak pressure to changes in spontaneous respiratory effort during VTV.

Studies in premature infants have shown reduced variability in V T , fewer ventilator cycles delivering too large or small V T , and less hypocapnia with VG compared with PC ventilation.
Another documented advantage of VTV is automatic weaning of PIP in real time, rather than intermittently in response to blood gas measurements. The effectiveness of this approach, however, appears to be dependent on the target V T . Targeting a very low V T resulted in rapid weaning of PIP, but this led to a compensatory rise in spontaneous breathing effort or resulted in higher transcutaneous partial pressure of carbon dioxide (P co 2 ) levels in those infants unable to compensate consistently.
Similarly, in a recent study a reduction in the target V T from 5 to 4 mL/kg resulted in a greater than 50% increase in work of breathing. Thus weaning should be done with caution because a target V T that is too low could lead to a large reduction in PIP and result in periods when the infant receives minimal or no ventilatory support ( Fig. 19.2 ). This and other studies underscore the importance of choosing an appropriate V T for each patient and clinical situation. Patient size, postnatal age, the nature of the lung disease, and the choice of synchronized mode all affect the optimal V T setting. It is also important to appreciate that it is the patient’s pH, not just the arterial partial pressure of carbon dioxide (Pa co 2 ), that is the primary regulator of respiratory drive. Thus patients with a significant base deficit will attempt to normalize their pH by achieving mild respiratory alkalosis. The low Pa co 2 often prompts weaning of target V T , which in turn results in automatic lowering of PIP and inadequate support when the patient generates a spontaneous V T above the set value.

In infants with spontaneous hypoxemia episodes triggered by acute reductions in V T and ventilation, VG reduced the duration of the episodes compared with PC ventilation. This was particularly evident with a higher target V T . Although the automatic increase in PIP during VG did not prevent the occurrence of the hypoxemia episodes, it reduced the need for increased fraction of inspired oxygen F io 2 to resolve hypoxemia.
In infants with respiratory distress syndrome (RDS), use of VG during the first week resulted in lower levels of inflammatory cytokines in bronchoalveolar fluid compared with PC ventilation. This has been attributed to reduced exposure to breaths with excessive V T . However, inflammatory markers were shown to be higher when these infants underwent ventilated with VG at a low target V T and low positive end-expiratory pressure (PEEP). These findings indicate that avoidance of hypoventilation and atelectasis may be as relevant as avoiding overinflation.
In randomized clinical trials in preterm infants with RDS, VTV resulted in faster weaning from mechanical ventilation compared conventional PC ventilation and a reduction in the composite of BPD or death. These studies did not focus on the subpopulation of premature infants who at present are more likely to require mechanical ventilation after birth—that is, infants of less than 28 weeks’ gestational age. Further investigation is necessary to confirm these findings in this population. Also, there are important differences between modalities of VTV that have been implemented and their relative advantages or limitations that have not been evaluated. Nonetheless, two meta-analyses that included a variety of modes of VTV demonstrated a number of advantages of VTV compared with PC ventilation, including decreased incidence of bronchopulmonary dysplasia, lower rate of severe intraventricular hemorrhage, lower incidence of periventricular leukomalacia, lower rate of pneumothorax, less hypocapnia, and shorter duration of mechanical ventilation. Although these meta-analyses have some limitations, they suggest that automated, real-time adjustment of inflation pressure is superior to intermittent manual adjustment. As of this writing, all of the ventilators in common use in neonatal intensive care units offer some form of VTV, making VTV the first reasonably well-established and widely available modality of automated respiratory support.
Targeted Minute Ventilation
Targeted minute ventilation (TMV) is a modality of respiratory support in which the cycling frequency of the ventilator is automatically adjusted to maintain minute ventilation at a target level. TMV is intended to reduce the respiratory support by providing fewer ventilator breaths when the infant is able to increase his or her contribution to minute ventilation. If minute ventilation exceeds the set target, the ventilator rate is automatically reduced to a lower limit or vice versa. In preterm infants recovering from RDS, TMV reduced the ventilator rate by 50% compared with SIMV while total minute ventilation remained within the same range. Transient increases in ventilator rate were observed during TMV to prevent hypoventilation during periods of central apnea or reduced V T . Typical adjustments of the ventilator rate during TMV are shown in Fig. 19.3 .

TMV was combined with automatic adjustments to PIP to simultaneously maintain targets of minute ventilation and V T , respectively. This modality was evaluated in an animal model that replicated the mechanisms leading to episodes of hypoxemia in preterm infants. Automatic increases in ventilator rate or PIP were effective in attenuating the severity of hypoxemia while the combined adjustments further improved ventilation stability and attenuated hypoxemia. This strategy has yet to be evaluated in infants.
Mandatory minute ventilation (MMV) is a modality in which the measured minute ventilation is compared with a set target level. Ventilator mandatory breaths stop when this level is exceeded by unassisted spontaneous breaths or by spontaneous breaths assisted with pressure support. When minute ventilation declines below the target, the ventilator delivers volume-targeted cycles at a constant rate to keep minute ventilation at the target. In near-term infants without lung disease, MMV reduced the ventilator rate compared with SIMV. As of this writing, this mode has not been evaluated in premature infants with lung disease.
Adaptive mechanical backup ventilation is a modality in which the ventilator monitors spontaneous breathing and arterial oxygen saturation (Sp o 2 ). In the occurrence of apnea or hypoxemia, the ventilator provides a backup rate until the episode is resolved. In preterm infants recovering from RDS, adaptive backup ventilation attenuated the frequency and duration of episodes of hypoxemia compared with conventional backup ventilation set to respond to apnea alone. These findings suggest a role for hybrid ventilation modes to improve oxygenation stability.
The effects of different minute ventilation targets in premature infants have not been assessed. A target level below the infant’s required total minute ventilation is likely to provide a background level of minimal respiratory support. On the other hand, a target level that is near or exceeds the infant’s total minute ventilation could result in the ventilator taking over and inhibition of the infant’s respiratory drive.
Proportional Assist Ventilation
The respiratory pump of the ventilated preterm infant must overcome increased resistive or elastic loads owing to the underlying lung disease to generate an adequate V T . Proportional assist ventilation (PAV) is a modality whereby the ventilator pressure is automatically adjusted in proportion to the volume, flow, or both, generated by the respiratory pump. This enhances the infant’s volume- or flow-generating ability and results in a reduction in the obstructive or restrictive loads that impede breathing. In PAV, the user determines the degree of mechanical unloading by setting the elastic (volume-proportional) and resistive (flow-proportional) gains in airway pressure.
The volume- and flow-proportional gains must be suited to each infant and require estimation of the respiratory compliance and/or airway resistance. Higher gains result in larger increases in airway pressure per unit of volume or flow generated by the infant. An excessively high elastic (volume) gain that exceeds the elastic recoil of the lungs can lead to a runaway increase in pressure, whereas a resistive (flow) gain that compensates beyond the airway resistance can lead to pressure oscillations.
Operator adjustments of the volume- or flow-proportional gains during PAV to maintain adequate ventilation parallel to some extent the adjustments in PIP during PSV or A/C ventilation to maintain an adequate V T . During PAV the airway pressure is automatically adjusted by the ventilator but the clinician is responsible for setting appropriate limits for peak pressure, delivered volume, inspiratory time, and the backup mandatory breaths for apnea. PAV resulted in reduced breathing effort and improved ventilation and gas exchange with lower peak pressures compared with IMV, A/C ventilation, and SIMV in preterm infants during the weaning phase of RDS and in the evolving phases of chronic lung disease.
It should be noted that the PAV approach assumes mature respiratory control, because it is a positive feedback mechanism that unloads the elastic and resistive forces to allow the subject with insufficient strength to generate the desired V T . The inconsistent and highly variable respiratory drive of the preterm infant may not be optimally suited for this form of assistance. Additionally, because the system by necessity responds to inspiratory flow and volume, a large leak around the uncuffed ETT would be interpreted as a large inspiration and given correspondingly high level of inflation pressure, potentially leading to dangerously large V T . Although PAV has been available for many years, it has not gained widespread acceptance, possibly because of its complexity and lack of documented advantage over other modalities.
Neurally Adjusted Ventilatory Assist
Neurally adjusted ventilatory assist (NAVA) is a modality where the ventilator pressure is automatically adjusted in proportion to the patient’s respiratory effort as measured the electrical activity of the diaphragm (EA DIA ). The proportionality factor or NAVA gain determines the increase in pressure per microvolt of EA DIA . Because the ventilator pressure increases or declines with the infant’s inspiratory effort, NAVA is proposed as a way to enhance the inspiratory effort and optimize synchrony. Fig. 19.4 shows adjustments in ventilator pressure during NAVA. Like PAV, this is a positive feedback system, raising concern about amplifying the variability of the immature infant’s respiratory effort.

NAVA has been shown to be effective in maintaining ventilation and gas exchange in premature infants comparably or better than modalities of conventional PC ventilation. This has been attributed to the better synchrony between the infant’s inspiration and the ventilator. However, no randomized trials with important clinical outcomes, such as bronchopulmonary dysplasia or intraventricular hemorrhage, have been conducted.
In NAVA, increasing the proportionality gain can produce an increase in V T , a reduction in EA DIA , or both. EA DIA is monitored continuously and is used to assess the patient’s inspiratory drive and adjust the NAVA gain as needed. However, data on the relation between the EA DIA and inspiratory effort or volume generation and the variability between or within patients are scant for preterm infants. The EA DIA , seen as an indicator of inspiratory effort, was reduced during NAVA compared with PC ventilation, but it was higher compared with VTV.
The reported findings on the use of NAVA are promising, but further research is needed to assess its physiologic and clinical effects in premature infants. In NAVA the level of support depends largely on the NAVA gain setting, but little is known about the effects of different NAVA gains or the methods to determine the most appropriate gain. Appropriate limits for PIP should be set to avoid excessive V T resulting from the positive feedback when the infant becomes agitated, and apnea ventilation is necessary when respiratory effort is lacking. The extent to which EA DIA can be compared between infants or within the same infant over time as an indicator of inspiratory drive is unknown. Thus further research is needed to determine the interaction between NAVA and the extremely premature infant’s immature respiratory control center. Use of noninvasive NAVA as a means of synchronizing noninvasive positive-pressure ventilation is gaining popularity because it is currently the only available method of synchronization of nasal ventilation available in the United States. Noninvasive NAVA is likely to be safer in immature infants, because excessive V T is unlikely to be achieved with an open system.
Automated Adjustment of Supplemental Oxygen
Most premature infants with respiratory insufficiency require supplemental oxygen to maintain adequate oxygenation. However, the fraction of inspired oxygen (F io 2 ) can be excessive at times and induce hyperoxemia, which increases the risk for eye and lung injury and oxidative stress in other organs, including the central nervous system. To avoid this problem, arterial oxygen saturation is continuously monitored by pulse oximetry (Sp o 2 ) and F io 2 is adjusted to keep Sp o 2 within a prescribed range. However, multicenter data revealed that preterm infants receiving supplemental oxygen spent only 48% of the time with Sp o 2 within the target range. They spent 36% of the time with Sp o 2 above (range 5%–90%) and 16% of the time below the target range (range 0%–47%). These data showed that maintenance of Sp o 2 within the target range worsens over time and is slightly improved by tight Sp o 2 alarms. Maintenance of oxygenation within the intended range has been shown to be largely influenced by the nurse/patient ratio.
Maintenance of Sp o 2 within the target range becomes increasingly difficult over time due to frequent fluctuations in Sp o 2 . These fluctuations also increase the demand for staff effort, and frequently the adjustment in F io 2 in response to episodes of hypoxemia is not optimal and infants are exposed to prolonged periods with Sp o 2 above and below the target range. In an attempt to attenuate the frequency of hypoxemia episodes, the clinical staff often tolerates Sp o 2 levels above the target ranges, thus exposing infants to excessive oxygen, as illustrated in Fig. 19.5 .

Systems for automatic adjustment of F io 2 have been developed to improve the maintenance of Sp o 2 within a target range and to reduce exposure to hyperoxemia and hypoxemia as well as supplemental oxygen in premature infants. Fig. 19.6 shows representative automatic adjustments to F io 2 in a preterm infant.

Clinical studies showed that automatic F io 2 control improved the maintenance of Sp o 2 within the target range compared with manual adjustments made by the infant’s caregivers and maintained the range comparably or better than a fully dedicated research nurse ( Table 19.1 ). These studies documented consistent reductions in hyperoxemia with use of automatic control ( Table 19.2 ). These findings confirm the tolerance of excessive Sp o 2 levels in premature infants during routine care. In these studies the exposure to supplemental oxygen was also reduced by automatic control.
Authors (Year) | Sp o 2 Target Range (%) | Time Within Target Range (%) | Manual Changes in F io 2 /24 h | Manual Mode | ||
---|---|---|---|---|---|---|
Auto | Manual | Auto | Manual | |||
Bhutani et al. (1992) | 94–96 | 81 | 54 69 | NA | 48–72 a 288–720 a | Routine Dedicated |
Morozoff and Evans (1992) | 90–95 | 50 | 39 | 25 a | 194 a | Routine |
Sun et al. (1997) | Set value ± 3% | 72 | 58 | NA | NA | Routine |
Claure et al. (2001) | 88–96 | 75 | 66 | 0 | 696 a | Dedicated |
Urschitz et al. (2004) | 87–96 | 91 | 82 91 | 7.2 a | 72 a 185 a | Routine Dedicated |
Morozoff and Smyth (2009) | 90–96 | 73 | 57 | 5 a | 89 a | Routine |
Claure et al. (2009) | 88–95 | 58 | 42 | 42 a | 204 a | Routine |
Claure et al. (2011) | 87–93 b | 47 | 39 | 10 | 112 | Routine |
Hallenberger et al. (2014) | All 4 centers: 90–95 80–92 83–93 85–94 | 72 71 69 66 84 | 61 63 64 43 65 | 52 | 77 | Routine |
Zapata et al. (2014) | 85–93 | 58 | 34 | 0 a | 160 a | Routine |
Waitz et al. (2015) | 88–96 | 76 | 69 | NA | NA | Routine |
Lal et al. (2015) | 90–95 | 69 | 60 | NA | NA | Routine |
van Kaam et al. (2015) | 89–93 b 91–95 b | 62 62 | 54 58 | 1 1 | 102 109 | Routine |
Plottier et al. (2017) | 91–95 b | 81 | 56 | 6 a | 55 a | Routine |
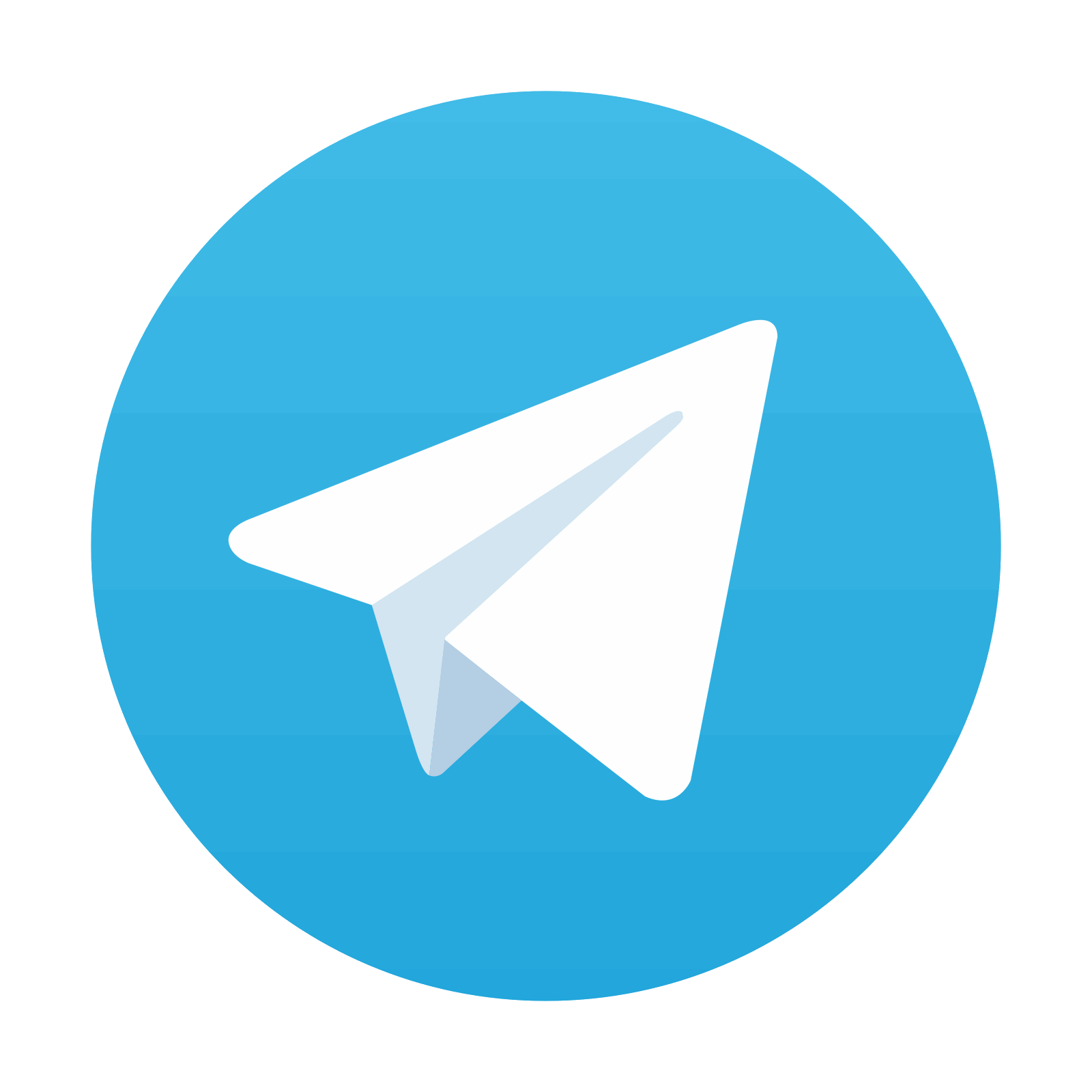
Stay updated, free articles. Join our Telegram channel

Full access? Get Clinical Tree
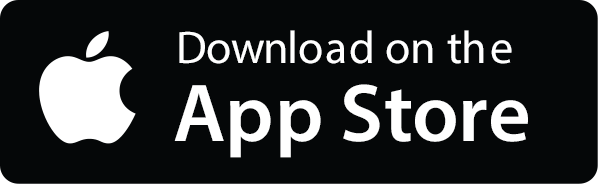
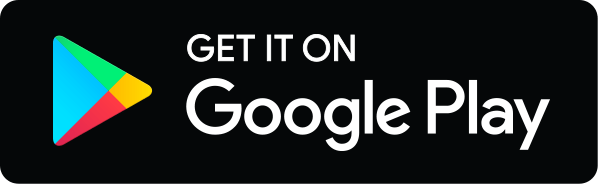
