Definition
Autism spectrum disorder (ASD), as defined by the American Psychiatric Association’s Diagnostic and Statistical Manual of Mental Disorders, fifth edition, (DSM-V) is a complex heterogeneous neurodevelopmental disorder with two main distinguishing features.
Impaired social communication and social interaction
Narrow or restricted and repetitive behaviors and interests
In contrast to the previous edition, in DSM-V ASD can be comorbid with other developmental disorders such as attention deficit hyperactivity disorder (ADHD), developmental coordination disorder (DCD), specific language impairment (SLI), and intellectual disability (ID).
ASD typically emerges by 3 years but rarely before 10 months of age. Some information provided below applies to follow-up care but is included here as a general introduction.
Incidence/prevalence
Reported prevalence of ASD has increased over the past 30 years from 1:1000 to ~1:88.
ASD prevalence associated with obstetric and neonatal factors that result in NICU admission is estimated to be between 1:32 and 1:77.
Severity of CNS injury and most clinical risk factors are individually associated with ASD risk, but are highly intercorrelated and likely reflected in the twofold increased risk of ASD if born preterm.
Since NICU admissions account for about 1/8 of live births with preterm birth a major cause, from 10% to 25% of all ASD cases are likely to be NICU graduates.
Pathophysiology
In summary, studies of the pathophysiology of ASD point to developmental disruption experienced early, most likely in the first trimester when CNS organization undergoes large developmental changes. First trimester abnormalities have cascading consequences for second trimester development, with long-term negative consequences expressed years later as ASD. These early adverse effects are not necessarily apparent as hemorrhage or other detectable injury on MRI, CT, or CUS after delivery, but may be visible only on autopsy, or possibly as brain volumetric deficiencies or neural track abnormalities.
The pathophysiology of ASD is assumed to be related to abnormal brain development.
Brain imaging links numerous brain centers and their interconnections with ASD, including regions in the cortex, cerebellum, limbic system, thalamus, and brainstem.
MRI studies show different developmental trajectories for both subcortical and cortical brain volume growth overall and in specific areas associated with ASD at least through 7 years and in some areas into adolescence.
A difference in growth trajectory, with increased head size associated with ASD, may indicate abnormalities in apoptosis and neuronal pruning that affect developing neural pathways.
Volumetric MRI of the brainstem, by contrast, indicates smaller volumes at birth, only reaching normal levels by adolescence.
Postmortem studies show abnormalities in cell development, migration, and connectivity in multiple brain regions linked to the serotonin system, oxidative stress, and associated factors.
Prenatal (first and second trimester) abnormalities
Placental abnormalities
ASD-associated placental abnormalities primarily involve lack of synchrony and poor arborization of the arterial system branching from central to peripheral areas of the placenta, suggesting poor fetal oxygenation and/or perfusion.
These first trimester disorganizing effects have multiple causes, including early infection, diabetes, and hypertension, and are coincident with problems that produce low gestational age (GA) and birthweight (BW) and intrauterine growth restriction (IUGR), and frequently occur in multiple gestation pregnancies.
Disruption in neural development
During the first trimester, brainstem, cerebellum, and basal ganglion subcortical systems begin to emerge and form neural networks.
Disruption can occur as a result of first trimester risk conditions likely due to complex deficits at microcellular levels (in pre- or mature oligodendrocytes, astroglia, and microglia) that control gene expression, protein synthesis, and neural connectivity.
Neurofunctional interactions occur within and between brain levels and with the environment, with higher-level subcortical and cortical systems beginning to emerge in the late second trimester.
Studies of genetic etiologies emphasize the complex epigenetic nature of ASD, in which interactions among multiple gene clusters involve specific gene sites that control developmental organization and plasticity in multiple brain regions throughout development.
Such processes also can be modulated by environmental factors/influences/events such as exposure to neurotoxins or antineuroleptic drugs.
Thus, deviation from expected normal trajectories begins early in fetal development affecting cell production, proliferation, and migration, thereby interfering with myelin growth and integrity, and synaptic connectivity in multiple neural pathways.
Brainstem and cerebellum
Subcortical development occurs in brainstem and cerebellar regions via neural proliferation from the subventricular plate as early as 7 to 20 weeks’ gestation. Early abnormalities in these regions are involved in the etiology of complex developmental dysfunction in ASD, eg, linkage between abnormalities in olivofloccular circuitry in ASD to eye-gaze problems.
Besides cerebellar damage, 90% to 100% of cases with encephalopathy of prematurity (EP) indicate early damage to the brainstem olivary complex and to the pontine region in the form of smaller and less numerous cells and problems in connectivity to the cerebellum.
The involvement of the brainstem is especially important, since it can be assessed routinely in NICU infants using auditory brainstem responses (ABRs).
Neonatal brain development
Disorganization of brain function continues to occur through NICU discharge, resulting from cascading consequences of atypical connectivity.
Effects on newly forming neural networks connecting brainstem and higher level structures include multiple subcortical systems with brainstem structures involved with autonomic systems, arousal, visual and auditory systems, and linked to cerebellum, striatum, and amygdala.
ASD-linked brain disorganization is further affected by CNS damage during the convalescent care period related to preterm birth, hypoxia, and other adverse conditions.
Risk factors
Multiple obstetric, prenatal, perinatal, and neonatal medical factors are associated with ASD risk. Most are comorbid with very low BW and GA, IUGR, and hypoxia. No biomarkers reported to date are adequately sensitive and specific for a definitive early diagnosis. However, converging pathways of risk may cause early deleterious effects on brain development, especially in brainstem, thalamic, and limbic regions, which might be part of a developmental trajectory to ASD, and are reflected to varying degrees by NICU admission.
Familial and genetic susceptibility
ASD affects 4.7 times more males (1:54) than females (1:252).
ASD runs in families. An estimated 10% to 20% of children with older ASD siblings, 20% to 30% of fraternal twins, and 60% to 70% of monozygotic twins will have ASD.
The increased risk of younger siblings of children with ASD has enabled prospective studies into signs of ASD from early infancy, providing important data about early ASD behavior and informing early interventions.
Many genes potentially linked to ASD involve common pathways affecting neural development. Most candidate genes have peak expression midway through fetal development.
Substantial comorbidity exists behaviorally and genetically between ASD and other developmental disabilities, genetic syndromes such as fragile X syndrome, Down syndrome, neurofibromatosis, and tuberous sclerosis and personality disorders; overlapping combinations of genes link ASD to these disorders, supporting shared genetic, epigenetic, and/or intrauterine environmental causes during fetal development as the underlying etiology.
Epigenetic effects and environmental stress
In ASD, biological and environmental pathways are related through changes in DNA or its expression by transporter RNA.
Potential factors include parental and even grandparental age (with a stronger paternal than maternal effect), placenta growth and integrity, hormonal states, oxytocin levels, maternal diabetes, depression, seizures, antiepileptic and other classes of drugs, and neurotoxins or pesticides (eg, cytokines, PCBs, autoemission organophytes).
Major environmental stressors interacting with arousal regulation during pregnancy may also play a role, because ASD is increased by maternal stress (especially in the second trimester). But this may occur only in mothers with certain allelic polymorphisms in the serotonin transporter gene, reinforcing the epigenetic nature of ASD and a potential association with maternal depression.
Comorbid effects of ASD and NICU admission and sociological factors
Biological
Besides male gender and preterm birth, multiple developmental risks associated with NICU admission also increase the incidence of ASD. These include
Assisted reproduction
Multiple-gestation pregnancies
Older age of one or both parents
Neurotoxic intrauterine conditions (such as transient hypoxia and maternal infection)
Frequent NICU medical procedures aimed at decreasing morbidity and mortality
Fragile, compromised system exposed to increased environmental conditions/stressors
Sociological
Recent factors potentially contributing to increases in ASD prevalence include
Better recognition of symptoms and behavioral features in infants and young children
Availability of early intervention and preschool programs, which may require a diagnosis of ASD
Awareness of importance of CNS dysregulation in disrupting fetal and neonatal maturation and development
Refinement of early diagnostic and intervention tools
A shift in conceptualization of etiology from one implicating inappropriate learning, poor parenting, and abnormal temperament or personality development to discrete categorical abnormality models and, most currently, to a quantitative dimensional representation of a brain disorder.
Discredited factors
Two former widely cited factors, (1) being reared by a “cold” mother, and (2) presence of mercury in early vaccinations, have been discredited.
For professionals, the myth of a “cold” parent altered therapeutic interventions (if any were recommended); for parents, it caused huge guilt; for children, it caused despair of improvement and even institutionalization.
For pediatricians, the vaccination myth changed practices; for parents, it caused decision-making dilemmas; but, worst of all, for many children it resulted not only in exclusion from school but in disease and even death. Preventable outbreaks and their consequences (eg, of measles) in communities where parents refuse immunization still continue and need to be addressed in a rational but strong manner by the medical community.
Decreased female risk
Epidemiological study of NICU admission by gender indicates sex-linked effects that increase male prevalence both in the NICU (54% versus 46%) and in preterm birth, despite an approximate 100-g advantage in BW.
Males apparently are more medically fragile, perhaps less mature, and either independently or as a consequence more susceptible to later disability.
Whether the effects result from more neonatal conditions linked to ASD in males or are secondarily linked to ASD for other reasons is not known, although interesting hypotheses concern hormonal factors that influence early subcortical neural brain development.
Hormonal factors
The 4.7:1 male/female ratio in ASD may be due to an interaction between testosterone production and sensitivity of androgen receptors, beginning in the first and peaking in the second trimester. This interaction results in increases in arousal in the brainstem and cerebellum that can disrupt neural organization in the thalamus and other subcortical areas later involved in arousal and attention.
Estrogen production and receptors in females do not generate this type of potentiated hormonal influence on arousal, resulting in much lower levels of androgens, and higher levels of oxytocin that downregulate androgens.
Thus, expression of gender-specific hormones in interaction with developing hormonal receptors and neural systems influences brain organization underlying arousal responsivity, thereby affecting behavioral responses to environmental stimuli such that males have a greater vulnerability with increased probability of ASD.
Serotonin
A gene modifier effect exists between ASD severity and an X-linked MAOA genotype (MAOA-uVNTR) in affected boys and their mothers that is similar to the effect of recurrent maternal depression on severity of ASD in offspring.
Since the primary substrate for MAOA is serotonin, prenatal maternal serotonin levels may influence outcome.
These genetic factors may contribute to resiliency or vulnerability, thereby influencing aberrant outcome in NICU boys, secondary to prenatal influences on CNS development.
Summary of risk
With multiple obstetric, prenatal, perinatal, and neonatal problems related to later ASD, there is a shift toward understanding ASD in terms of complex interactions of environmental factors and early brain organization rather than any single cause. ASD then becomes a chronic rather than an acute CNS problem that affects the presence and severity of later symptoms. Exposure to specific drugs during pregnancy (eg, antiepileptics, antidepressants), toxic chemicals (eg, pesticides, autoemissions), and other neurotoxic events (eg, placental insufficiency, hypoxia, maternal infection) also appear to produce chronic more than acute effects. These effects are strongest in the first and second trimesters, producing long-term adverse consequences in multiple structural and functional domains. They directly and/or indirectly influence brain development, particularly in interaction with fetal and neonatal growth. They are epigenetic, affecting gene expression and translation, and modulating neural proliferation and connectivity. Thus preterm birth, low BW, male gender, and numerous medical problems render NICU infants at greater risk for ASD, but ongoing medical problems occur more in babies who later develop ASD than with medically equivalent NICU infants. Whether this is a reflection of their higher ASD risk or their continued medical problems increases their ASD risk is not known.
Resiliency
Despite increasing rates of ASD, it still remains relatively uncommon. Neurofunctional development is resilient.
In the event of an abnormal preterm or adverse delivery, the likelihood of a major abnormal outcome such as ASD, even after one or more risks, is low.
Ascertainment of risk in NICU ASD cases
Most studies attempting to predict ASD (eg, studies of younger siblings of ASD children) exclude risk conditions such as preterm birth, NICU assignment, and multiple gestations because of potential confounds.
Even if the symptomatology in a “healthy” term nursery infant with ASD (eg, irritability, GI problems) is different from the NICU infant with or without ASD who frequently has these symptoms, the pathophysiology may be similar.
The increased risk of ASD in the NICU infant needs to be addressed. Just as parents of high-medical-risk infants need to be informed of potential outcomes such as cerebral palsy, ASD should be cited as a potential adverse outcome for certain NICU graduates (eg, VLBW).
Clinical presentation
Signs and symptoms
Early
No signs or symptoms during the intensive care phase specifically relate to ASD.
Slow recovery and high recurrence of problems (not a typical “feeder and grower”) are sensitive but not specific indicators of later ASD.
These include atypical immaturity, persistent bradycardia, slow maturation of lungs with prolonged ventilator/CPAP support, feeding issues, poor weight gain, general instability, poor state regulation, and lack of smooth progress with abnormal growth and developmental trajectories.
Convalescence
Signs of neurofunctional disorganization potentially related to ASD are more apparent when the infant is stable enough to be assessed for extent and degree of recovery, typically at NICU discharge.
Continued indications of brainstem dysfunction result in longer hospital stays that are exacerbated by prolonged respiratory and feeding problems. Such indications may reflect failure to thrive, slow development and rate of recovery, and continued autonomic dysregulation.
This transition out of the neonatal period represents development from brainstem and subcortically mediated to higher-level limbic and cortical functioning. It forms the initial anchoring for subsequent developmental trajectory transition over function and age points. Although statistically ASD-risk and high-medical-risk predate later disability and may follow separate trajectories in their eventual outcomes, their joint effects typically interact adversely and lead to increased ASD severity.
Assessment of brain structure and function
Assessment of neurofunction is used to evaluate current status, characteristics and speed of recovery, and trajectories across age. Since CNS injury and atypical neurofunction are related to ASD, a variety of techniques are useful, especially when combined with structural imaging to help define the extent and character of early brain insult. Further examination of potential early precursors to ASD indicates that ASD is best predicted by the conjoint association of an initial abnormal ABR (that resolves) with atypical visual preferences at 4 months.
Auditory brainstem responses
Although typically used to assess hearing, ABRs have general utility for assessing neural integrity noninvasively, especially when myelin dysfunction is suspected.
Transmission from one brainstem region to the next can be inferred, allowing precise measurement of auditory tract transmission speeds. Electrical potentials are embedded in scalp recordings of ongoing brain electrical activity emanating from brainstem regions, as long as the potentials are synchronized by some source, in this case an auditory stimulus.
ABRs, besides correlating to concurrent subcortical structural insult in NICU infants (eg, IVH), are useful for identifying infants with worse ASD prognosis.
ASD is associated with first- to second-trimester mechanisms that control the integrity of myelin sheaths that, in turn, directly affect transmission in brainstem regions likely affected in ASD. ABRs may be especially useful in tracking ASD because the superior olivary nuclei involved in processing auditory information, are also implicated in ASD through connections to cerebellum.
Brainstem involvement is indicated by initial abnormal delayed ABR latencies that typically resolve before NICU discharge. They are most informative when performed within 2 to 14 days after birth but not before 32 weeks’ PCA (postconceptional age).
Electrical potentials are embedded in scalp recordings of ongoing brain electrical activity emanating from brainstem regions, as long as the potentials are synchronized by some source, in this case an auditory stimulus. Transmission from one brainstem region to the next can be inferred, allowing precise measurement of auditory tract transmission speeds. Transmission speeds are detected for the first 10 to 12 ms from the cochlea through brainstem nuclei to projections to auditory cortex. Slower transmission typically indicates immature or abnormal myelin support. Immaturity versus abnormality is differentiated by reference to PCA-dependent norms and to rate of change over PCA with repeated tests.
When CNS pathology such as IVH is detected, ABR abnormality is also present 85% to 90% of the time. ASD infants are more likely than other NICU infants to have abnormal ABRs, with delays in transmission resembling those of infants with significant structural CNS insults even when little or no evidence of structural CNS insult is identified.
Neonatal neurobehavior (NB)
Various procedures are used to track NB integrity, observe quality of performance, and determine degree of recovery from abnormality; they typically are performed at hospital discharge and repeated at 4 to 6 weeks’ PTA.
Most assessments of neurobehavior are (1) dependent on CNS maturity and integrity, (2) affected by CNS injury or dysregulation, and (3) modified by experience and practice.
Performance is judged on a range of behaviors differentially sensitive to CNS injury. These include elicited behaviors such as visual/auditory attention and symmetry, head/trunk control, movements/tone of extremities, state regulation (alertness, irritability), feeding, and the quality of spontaneous movements.
By the time of hospital discharge, differential performance depends on CNS integrity. NICU neonates without indication of CNS or ABR abnormality perform well in all areas. Neonates with significant CNS injury have the highest incidence of abnormalities, especially in motor systems, and more in lower than upper extremities (usually higher tone).
There is a constant rate of recovery in all abnormal groups by 1 month corrected age that is not shown by ASD infants. Visual (asymmetric tracking) and upper body problems (usually lower tone) persist in ASD infants even when compared to matched NICU controls with similar CNS insults. These eye movement and upper extremity control issues at 1 month may be linked to odd lateral gaze, head lag, and high-guard arm position observed at older ages.
Arousal regulation of attention
The ability to modify attention to adjust to varying levels of arousal-producing events is characteristic of normal neonates and reflects brainstem-mediated autonomic system development related to later regulatory processes, temperament, executive function, and cognitive performance.
Normal neonates are excellent modulators. They look to more stimulating events when less aroused (after feeding) and less stimulating events when more aroused (before feeding or with additional stimulus exposure).
In contrast, neonates with an initial abnormal ABR (that resolves) with no or slight CNS insult, or with significant CNS insult, have regulatory problems; they prefer less stimulating events even when less aroused. Neonates with indication of lower dopamine uptake (eg, with intrauterine cocaine exposure) have opposite regulatory problems, seeking stimulation even when more aroused. By 4 months corrected age, when responses are mediated by higher subcortical and sensory-specific cortical levels, the neonatal pattern of arousal modulated attention is superseded by a much-abated preference for more stimulation regardless of arousal condition or CNS injury.
However, infants later diagnosed with ASD do not show an abated response and continue to prefer more stimulation when less aroused, indicating an insufficient shift in attention from that seen during the neonatal period.
Condition variability
There is a wide range of variability in risk and in the number and severity of signs in clinical presentation, likely involving environmental factors as well as genetic and epigenetic interactions.
Diagnosis
Although risk factors and signs related to ASD may be present, no ASD diagnosis is made this early in development.
Differential diagnosis of ASD versus other comorbid developmental disabilities by risk factors and signs is not possible. Associations may be sensitive but not specific.
Despite this, a trajectory toward ASD and other developmental disorders likely begin to coalesce by the end of this period.
Management
Medical management is based on the infant’s needs and system analyses according to best practices and is not different from other high-medical-risk neonates receiving intensive care, although awareness to the neonatal risks and the link to familial history of ASD should be noted.
NICU infants need to be followed closely by experienced clinicians who can integrate across disciplines, since signs may be subtle and even fall within expected ranges. As with all high-medical-risk infants, it is important to
Understand and follow both neurostructural and neurofunctional development.
Track resolution of indicators of CNS dysfunction, including the ABR if still not normalized by discharge.
Assess NB functioning.
Obtain therapeutic intervention as needed.
Involve parents and staff in appropriate therapeutic interventions, eg, for eye-motor or other motor control problems.
Start discharge plan for support services and continuity of medical and behavioral care.
Any familial history of ASD should trigger more intense scrutiny during convalescence and follow-up.
Developmental/therapeutic interventions
With more bradycardia, respiratory problems, inadequate weight gain, and longer stay in hospital, the key is to maintain a supportive environment, encouraging homeostasis, autonomic regulation, and sensory and motor development through external intervention as required.
Interventions for NICU infants at higher risk for ASD, as for other high-medical-risk neonates, are specific to the individual infant’s needs. Therapeutic intervention should
Be specific to any identified abnormality
Facilitate active more than passive participation by the infant
Be as frequent and intense as can be tolerated, with the aim of increasing tolerance
Be appropriate to neurodevelopmental level (age adjusted for preterm birth by using PCA)
Use a qualitative rather than a quantitative delay model to specify abnormalities
Evaluate regulatory functions/signs of dysregulation
Involve NICU staff, caregivers, and family members for administration at more frequent and best times
Prognosis
Early predictors
Familial ASD history, exposure to toxins, delayed recovery, extended time on ventilator, feeding intolerance, failure to thrive, poor autonomic stability, and estimates of brainstem dysfunction serve as predictors and may help determine eventual outcome.
Information needed for later differential diagnosis can be obtained from a variety of sources that evaluate CNS structural and functional integrity. In all periods, from acute to follow-up, the need to document transient abnormality, especially involving subcortical systems, should be emphasized, since rate of recovery helps determine prognosis.
Outcome
Not necessarily different from other high-medical-risk infants while under intensive care.
Generally, ASD likelihood increases as the positive valence of predictors decreases (the greater the number/severity of the symptoms, the worse the outcome).
Decreased likelihood or severity of ASD is predicted with speedier recovery, fewer days to discharge, normal initial ABR, and fewer abnormalities on NB assessment, especially in the visual system and control of the upper body and extremities. Thus, outcome depends on the number and severity of risk factors and symptoms evaluated over infancy until diagnosis can be made.
Discharge
Prior to NICU discharge, it is important to plan a smooth transition to home, and continued intervention as needed. More parental support may be necessary as parents confront an overwhelming task as symptoms later cascade toward ASD, especially with family and work-related responsibilities, and family history, other medical issues, and comorbidity with other disabilities.
Teaching
As with other outcome risks, parents should be made aware of the increased risk for ASD, as it relates to their infant’s premature birth and neonatal course.
Monitoring
Infants at greater risk for developing ASD should be monitored closely in a high-risk infant follow-up clinic until the early toddler years.
Safety
No condition-specific safety concerns at the time of NICU discharge.
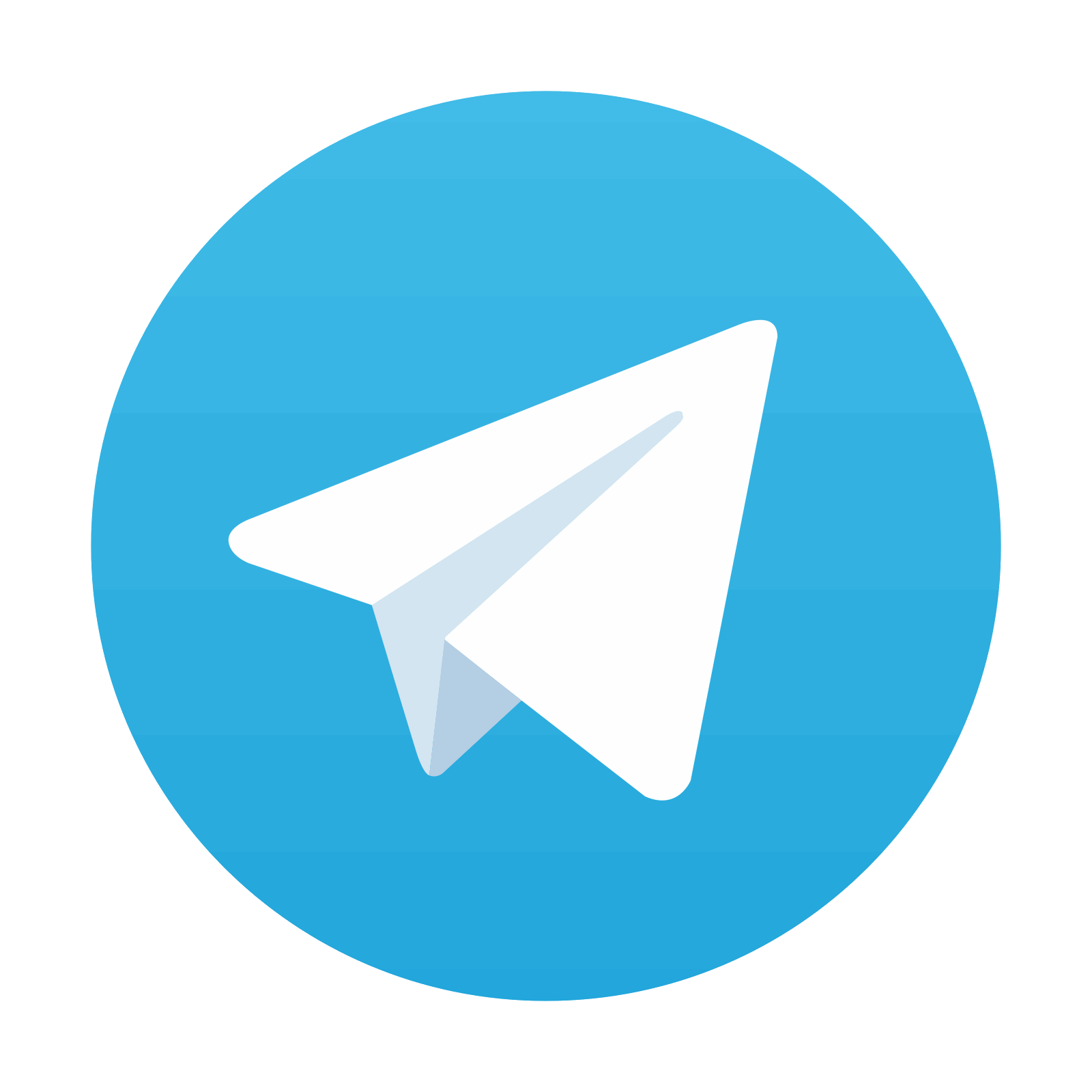
Stay updated, free articles. Join our Telegram channel

Full access? Get Clinical Tree
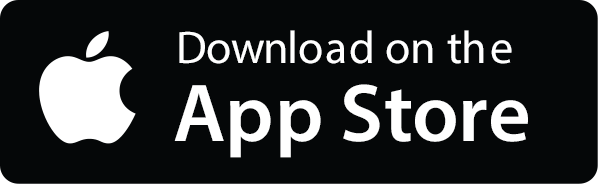
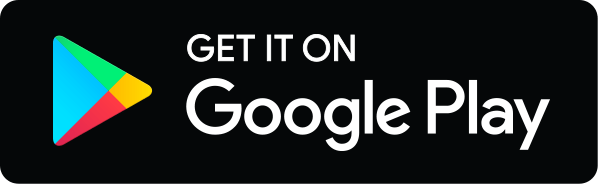