Patient factors
Age
Height and/or length
Weight
Temperature
Anatomic variability (e.g., Martin-Gruber anastamosis)
Environmental factors
Electrical (60 Hz) interference
Drug administration (pyridostigmine, neuromuscular blockade, botox)
Machine/operator factors
Stimulation malposition
Electrode malposition
Cathode position (erroneous reversal of stimulator polarity)
Submaximal stimulation applied
Stimulus artifact
Adjacent nerve co-stimulation
Filter settings
Measurement errors
Sweep speed and sensitivity errors
Patient Factors
Age
Age is one of the most commonly encountered variables encountered by neurologists and neurophysiologists who work with children. Clinicians are well aware of the dramatic changes in neurodevelopmental milestones that occur during the first few years of life. The changes in central and peripheral nerve myelination are equally remarkable, though less commonly recognized, over these years. Interpretation of pediatric nerve conduction studies must always include consideration of a child’s age. Axon diameter and myelination gradually increase from birth until adult values are attained at 4–5 years of age [1]. Not surprisingly, the normal reference ranges for electrodiagnostic tests also change with age, reflecting the progressive myelination that occurs in the first few years of life. Several reports have documented term newborns to show ulnar nerve motor conductions in the range of 20–35 m/s, with adult nerve conduction velocities not consistently observed until 3–5 years of age (Fig. 11.1) [2, 3]. This maturation must be considered when interpreting nerve conduction studies of infants and young children so as to avoid misinterpreting normal physiological differences as a sensorimotor polyneuropathy with demyelinating features. Normal values have been derived from prior published reports and are detailed in Chap. 24.

Fig. 11.1
Normal changes in ulnar motor nerve conduction velocity within the first few years of life, reflecting progressive growth and myelination [2]
Temperature
Temperature is another important and common artifact. Practically, there are several reasons why aberrant skin temperatures may be encountered in the EMG laboratory. Patient anxiety or apprehension is not unusual, particularly in children who are about to undergo nerve conduction testing. Physiologically, this anxiety may be associated with the increased circulation of epinephrine. Blood vessel constriction occurs as part of the “fight or flight” response such that blood is shunted to muscle [4]. Circulation to the skin is also reduced to potentially mitigate blood loss in the event of injury. As a result, the hands and feet of anxious individuals tend to be cooler than usual [5]. Sweating may further cool the hands. Cool ambient air temperatures including air conditioning in a patient wearing summer attire can further augment this problem. Regardless of the exact cause, temperature artifacts are common and can lead to interpretation error and misdiagnosis.
Temperature can affect peripheral nerve function by influencing ion channel function as well as neuromuscular transmission by altering acetylcholinesterase activity. Cooler temperatures will affect both sensory nerve action potentials (SNAPs) and motor compound muscle action potentials (CMAPs) by: (1) reducing conduction velocities; (2) increasing onset or peak latencies; and (3) increasing SNAP or CMAP amplitudes (Fig. 11.2). This pattern of abnormality can mimic compression neuropathies and potentially give rise to erroneous diagnoses; a common example is carpal tunnel syndrome.

Fig. 11.2
Median nerve sensory and motor responses showing faster conduction velocity and shorter onset latencies at warmer (32 °C) temperature compared to cooler limbs
Limb cooling affects both sensory and motor nerves. Action potentials are propagated along a myelinated axon as the voltage-gated sodium channels at each node of Ranvier open and sodium ions move along their electrochemical gradient into the axon. After the initial influx of sodium ions, these channels close. Potassium channels then open, resulting in an efflux of potassium ions, thereby restoring the resting membrane potential. Physiologically, cooling slows the rate at which sodium channels open and, to an even greater extent, the rate at which they close. The slower rate of opening gives rise to the delay in the propagation of action potential along the axons resulting in a slower conduction velocity. This delayed closing results in higher amplitude and longer duration action potentials which are reflected in the higher SNAP and CMAP amplitudes that are observed in patients with cool limbs.
Studies have shown predictable neurophysiological changes with cooling. Ulnar sensory and motor nerve conduction velocities decrease by 1.6–2.1 m/s for every 1 °C of cooling and distal latencies increase by 0.2 ms for every 1 °C of cooling [6]. Other studies have confirmed a conduction velocity decrease of 1.5–2 m/s for each degree of cooling [7, 8]. As such, limb temperatures should be measured and, if necessary, re-warmed to attain temperatures in the 33–34 °C range [9]. Other sources recommended a range of 34–36 °C for the upper limbs and 31–34 °C for the lower limbs [10]. Caution must be taken when hands are warmed since the superficial tissue will warm more quickly than deeper tissue, which can cause the limbs to cool off more quickly than expected after the study has been restarted [11].
Weight
Adipose tissue will insulate peripheral nerves such that stimulating electrodes may have greater difficulty delivering supramaximal stimulation. This is more problematic at proximal stimulation sites and can be observed in healthy infants as well as children and adults who have limb edema and/or obesity. Practically this is reflected in an amplitude drop at the proximal stimulation site compared to the more distal stimulation site(s) which can mimic axonal injury and/or conduction block. Caution must be taken not to overcall conduction block in such individuals, particularly when studying the tibial nerve motor response. Subcutaneous adipose tissue distributions often makes it difficult to elicit sural sensory responses in infants.
Height
Patients’ heights or limb lengths are known to influence late responses (e.g., F-response minimum latency) when completing neurophysiological testing in adults. Correction factors, namely the F-estimate, can be used to calculate the minimum F-responses in patients who are tall and consequently have longer limb lengths [12]. However, several studies have also demonstrated an inverse relationship between sensory nerve conduction velocity and adult patients’ height [13–15]. Cadaver studies have established that sensory nerve conduction velocities are slowed by 3.9 m/s for every 10 m of axonal length.
Normal pediatric values exist for pre-pubertal children of various ages. The precise contribution of height versus age-related myelination changes is less well known for this population. As such, greater emphasis is placed upon age rather than height per se when evaluating pediatric studies. Exception may be taken for very tall adolescents who should follow adult rules for correction factors as they apply to late responses.
Anatomic Variability
Children can demonstrate the same patterns of anomalous innervation seen in adults, especially as these anatomical variants are generally congenital. The classic Martin-Gruber anastomosis (MGA) should be considered in any child who demonstrates a higher CMAP amplitude when stimulating the ulnar nerve at its distal stimulation site (wrist) relative to a proximal site (below or above the elbow). MGA has been reported in up to 15–20% of adult patients [16], and presumably a similar proportion of children are affected. MGA results from the cross-over of median-to-ulnar nerve motor fibers supplying the hypothenar muscles. It has been referred to as a “pseudo-conduction block” as it has the potential to mislead inexperienced electromyographers into thinking that it represents evidence for a demyelinating disorder such as chronic inflammatory demyelinating polyradiculoneuropathy (CIDP) or multifocal motor neuropathy. The presence or absence of MGA can be confirmed by stimulating the median nerve proximally (elbow) while leaving the recording electrodes over the hypothenar muscles. The sum of the CMAP amplitudes recorded over the hypothenar muscles while stimulating the proximal median and ulnar sites should equal that seen with stimulation at the distal ulnar site in this condition. Other anomalous innervations include the Marinacci anastomosis (forearm ulnar-to-median communication), Riche-Cannieu anastomosis (palmar median-to-ulnar communication) and Berrettini anastomosis (palmar ulnar-to-median communication).
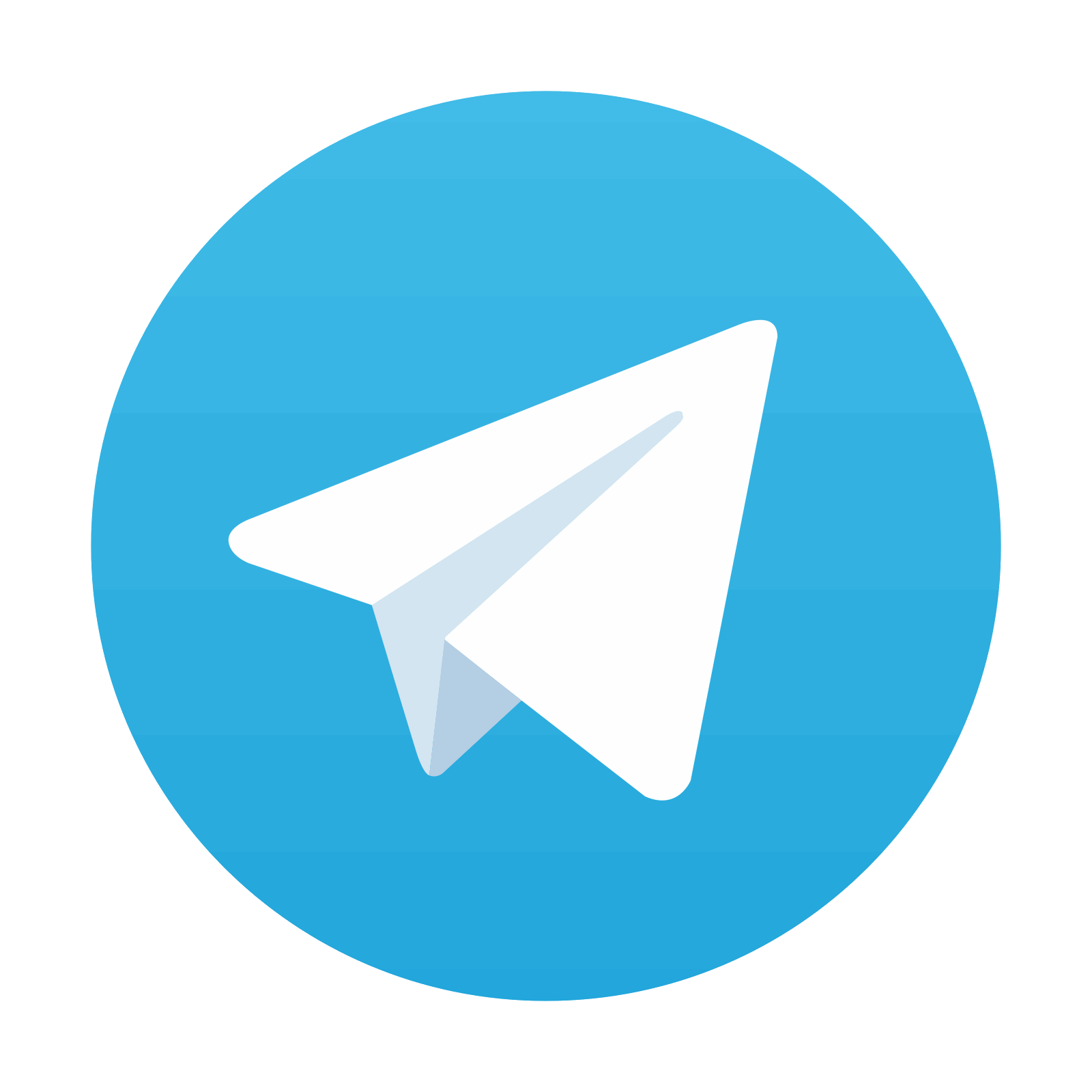
Stay updated, free articles. Join our Telegram channel

Full access? Get Clinical Tree
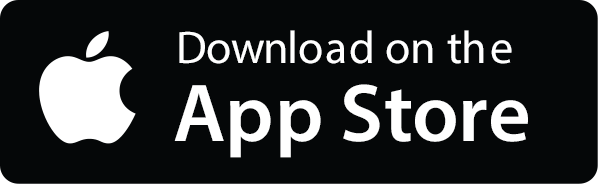
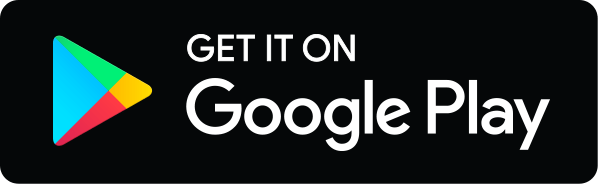