Fig. 44.1
Airway scalars. Airway pressure (Paw), flow, and volume (Vt) are graphically displayed over time
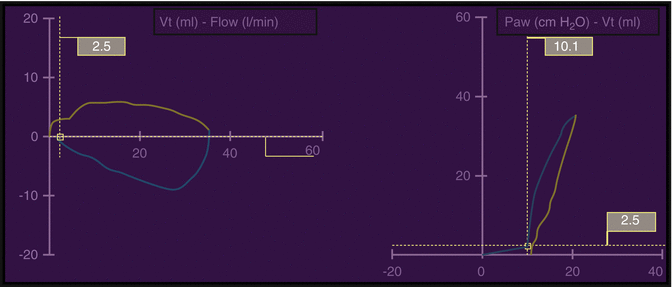
Fig. 44.2
Airway loops. Flow-volume (Vt) and pressure (Paw)-volume (Vt) loops as typically displayed by most ventilators with inspiration being represented by the yellow lines and expiration represented by the blue lines
In contrast to scalars, airway loops depict one variable in relation to another, rather than versus time (Fig. 44.2). The airway loops are particularly helpful in assessing alterations in airway resistance, pulmonary compliance, patient work of breathing, pulmonary overdistension, and “gas trapping” (i.e., premature termination of exhalation). The standard pressure-volume loop graphs pressure on the horizontal axis and volume on the vertical axis. On the pressure-volume curve, the slope of the inspiratory limb represents compliance. Flow-volume loops depict flow on the vertical axis and volume on the horizontal axis. These loops also can be employed to assess a number of important aspects of ventilator management, including large and small airway obstruction as well as expiratory airway resistance.
The overall goal of this section is to provide clinicians with an approach to optimizing mechanical ventilation strategies using a comprehensive, but simple, bedside assessment of patient-ventilator interactions through the analysis of airway graphics.
44.2 Respiratory Alkalosis/Acidosis: Alveolar Ventilation
44.2.1 Increased Ventilatory Requirements
The elimination of the carbon dioxide (CO2) produced from the metabolic processes throughout the organs and tissues of the body is a vital function of the respiratory system. Inadequate alveolar ventilation and CO2 clearance secondary to respiratory muscle failure is one of the most common reasons for inability to wean from mechanical ventilation in the neonatal and pediatric populations.
Respiratory pump failure may be due to decreased ventilatory capacity and/or increased respiratory muscle load; either of which may, in turn, be secondary to a wide range of disturbances including shock, hypoxemia, and hypercapnia (Table 44.1). Diminished effective tidal volume delivery created by impaired respiratory muscle function leads to inadequate alveolar ventilation and, subsequently, respiratory acidosis. Clinically, this respiratory muscle dysfunction is often demonstrated as the development of tachypnea. In this context, the presence of hypercapnia despite a significant increase in respiratory rate should be concerning and generally represents an indication to discontinue weaning from mechanical ventilatory support.
Table 44.1
Typical causes of respiratory pump failure
Decreased ventilatory capacity |
Respiratory muscles |
Hyperinflation |
Malnutrition |
Metabolic derangements |
Decreased oxygen supply |
Disuse atrophy |
Fatigue |
Abdominal wall defects |
Neurologic |
Decreased respiratory center output |
Cervical spinal cord surgery |
Phrenic nerve dysfunction |
Increased respiratory muscle load |
Increased ventilatory requirements |
Increased CO2 production |
Increased dead space ventilation |
Inappropriately elevated ventilatory drive |
Increased work of breathing |
Decreased efficiency of breathing |
Increased chest wall compliance |
Respiratory pattern |
Failure to wean from mechanical ventilation may also result from increased ventilatory requirements, which can be secondary to increased CO2 production. Reasons for increased CO2 production include excessive carbohydrate intake, fever (10 % increase for each °C), and excessive muscle activity (e.g., seizing, shivering, rigors). In this setting, augmenting alveolar ventilation may be necessary to maintain normocarbia. Another reason that increased minute ventilation may be necessary to maintain effective ventilation is to compensate for an increased ratio of physiologic dead space to tidal volume (V D/V T) as occurs with decreasing pulmonary capillary blood flow (which is often caused by a low cardiac output state), significant obstruction of the airways, or excessive distending airway pressures. Finally, excessive respiratory drive from psychological stress, central nervous system abnormalities, or pulmonary receptor stimulation may stimulate inappropriate hyperventilation and a secondary increase in respiratory muscle load.
44.2.2 Respiratory Acidosis
Hypercapnia and associated respiratory acidosis are the consequence of inadequate CO2 elimination in relation to production and may develop during the weaning phase of mechanical ventilation. This respiratory acidosis can have clinically significant effects including alterations on pulmonary vascular resistance (PVR), ventricular performance, systemic hypertension, ventricular ectopy, and catecholamine release. Hypercapnia may be caused by inadequate CO2 elimination, increased production, and/or an airway obstructive process.
Airway obstruction results in an inadequate CO2 elimination when inspired gas is unable to reach the alveoli or, more commonly, when alveolar gas is unable to be exhaled. Obstructive airways disease may be inspiratory and/or expiratory and can be caused by pathology of the alveoli, small airways, and/or larger airways. Irrespective of the location of the obstruction, the end result of inadequate effective alveolar ventilation is manifested by a decrease in effective V T, leading to tachypnea and possibly hypercapnea.
Hypercapnia, in and of itself, does not necessitate intervention and in many circumstances is not harmful (Peltekova et al. 2010; Laffey et al. 2000; Ni Chonghaile et al. 2005; Kavanagh et al. 2006). There is evidence to support permissive hypercapnia as a ventilatory strategy in which the partial pressure of arterial carbon dioxide (PCO2) is allowed to increase as long as an “acceptable” pH is maintained. Of course, “acceptable” values for pH and PaCO2 vary between individual patients, within an individual patient over time, and among clinicians.
However, the respiratory acidosis associated with significant hypercapnia can have detrimental effects on ventricular function and the pulmonary vasculature. When respiratory acidosis develops, an appropriate increase in the delivered tidal volume may be required to increase minute ventilation and correct the associated hypercapnia. While there are various noninvasive techniques (i.e., capnography and transcutaneous monitoring) to assess CO2 elimination, arterial blood gas analysis is most commonly utilized. As PaCO2 is inversely related to alveolar ventilation, a reduction in minute ventilation by 50 % results in a doubling in the PaCO2. As ventilator interventions are implemented to improve acidosis and reduce PaCO2, airway pressure and delivered tidal volume should be monitored to ensure that they remain within the nontoxic range as ventilatory support is increased.
In the assessment of unexplained hypercapnea and respiratory acidosis, patient-ventilator dys-synchrony is an important consideration. This abnormality in the relationship between the patient and the ventilator may cause an individual to compensate for the abnormal patient-ventilator interactions by increasing spontaneous respiratory effort, which may further exacerbate the patient-ventilator dys-synchrony. Patient-ventilator dys-synchrony most commonly occurs if overall ventilatory support is inadequate, the inspiratory flow does meet patient demand, and/or the trigger is not adequately sensitive. Patient agitation related to dys-synchrony may undermine ventilatory interventions, require ventilator adjustments, generally in the flow and/or trigger settings,and necessitate an increase in sedation and/or neuromuscular blockade to improve gas exchange, as discussed later in this chapter (Epstein 1971; Gibney et al. 1982; Greenough et al. 1983; Stark et al. 1979).
44.2.3 Respiratory Alkalosis
Respiratory alkalosis is generally less common (and less clinically concerning) in the intensive care unit setting than respiratory acidosis. Compensatory respiratory alkalosis develops in most spontaneously breathing patients as a response to significant metabolic acidosis. Treatment of the underlying metabolic process is essential along with (or prior to) ventilator interventions to correct a respiratory alkalosis. It should be noted that in a mechanically ventilated patient with a primary metabolic acidosis, ventilator weaning could have a profound impact on pH, exacerbating the overall acidotic state.
Another cause of respiratory alkalosis is neurologic dysfunction and an inappropriate stimulation of the central nervous system. An abnormal respiratory drive will induce respiratory alkalosis secondary to intrinsic abnormalities of the respiratory centers of the brain. In this situation, the underlying process should be fully assessed and addressed as clinically indicated.
44.3 Patient-Ventilator Dys-synchrony
Patient-ventilator dys-synchrony occurs when a patient’s spontaneous inspiratory effort is out of phase with the positive pressure breaths of the ventilator, resulting in a patient “fighting” the ventilator. When ventilatory support is dys-synchronous with patient effort, oxygen (O2) consumption of the respiratory muscles may be excessively high and creates an imbalance between O2 delivery and consumption. This additional respiratory effort and dys-synchrony may lead to a reduction in the effective tidal volume delivery and a subsequent respiratory acidosis. Patient-ventilator dys-synchrony is diagnosed by careful observation of the patient and a comprehensive assessment of the ventilator’s airway graphical display. In the differential, ventilation/perfusion mismatch, mucous plugging, pneumothorax, and reactive airways disease must be considered. Once these causes are addressed, altering ventilatory mode, increasing overall ventilator support, adjusting inspiratory flow, improving trigger sensitivity, and/or administering pharmacologic sedation (preferably as a last intervention) will generally improve synchrony between the patient and the ventilator.
44.3.1 Patient-Ventilator Interactions
Graphical waveforms are useful in the assessment of the characteristics of ventilator function and provide a graphical display of the impact of mechanical ventilatory strategies. The timing sequence of various ventilatory events relative to a patient’s respiratory effort can be assessed by airway scalars (Fig. 44.1). Ventilator dys-synchrony becomes evident when the timing and magnitude of pressure, volume, and especially flow are disproportionate or delayed. Using airway graphics analysis, clinicians can titrate ventilator breaths to improve patient-ventilator synchrony, reduce patient work of breathing, and calculate a variety of physiologic parameters related to respiratory mechanics (Tobin et al. 2001).
Airway graphic analysis can be used to continually assess respiratory pathophysiology by evaluating inspiratory flow, tidal volume, airway pressures, compliance, airways resistance, and pressure-volume and flow-volume relationships. Airway graphic analysis can assist with determining the effectiveness of respiratory interventions and changes in a patient’s respiratory status over time. In addition to patient-ventilator dys-synchrony (including excessive patient work of breathing), abnormalities frequently diagnosed by airway graphic analysis include pulmonary overdistension, excessive air leak, premature termination of exhalation/dynamic hyperexpansion (“gas trapping”)/intrinsic positive end-expiratory pressure (PEEPi), inspiratory and expiratory airway obstruction, and increased airways resistance.
44.3.2 Trigger Dys-synchrony
Trigger insensitivity/dys-synchrony is the inaccurate sensing of a patient’s inspiratory effort, which can result in either the failure of a ventilator to respond to a patient’s effort or the auto-triggering of a ventilator without any patient effort. An intubated patient’s attempt to inspire results in a decrease in the airway flow and/or pressure from baseline in the ventilator circuit, which should trigger a ventilator-assisted or ventilator-supported breath. However, when trigger sensitivity is inadequate, the ventilator may be unable to determine that patient effort has actually occurred, as seen in by the first three breaths in Fig. 44.3. Equally problematic, as seen in breath 4 in Fig. 44.3, the ventilator may deliver a full mechanical breath without regard to patient effort. When a patient’s effort is inadequately sensed, this trigger dys-synchrony can result in tachypnea, increased work of breathing, and patient discomfort (“fighting the ventilator”).
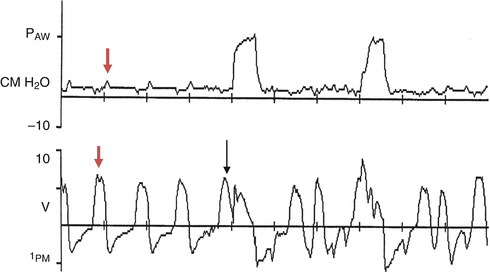
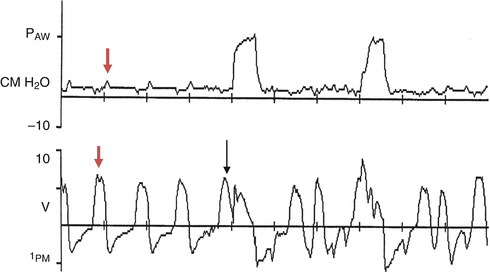
Fig. 44.3
Trigger dys-synchrony. Deflection in the flow tracing without an appropriate deflection in the pressure tracing reflects a breath not sensed by the ventilator (red arrows). Although the patient can move a flow of gas by breathing the bias flow available in the circuit, the patient effort is inadequate to generate a full mechanical or pressure support breath. The black arrow denotes a ventilator breath being initiated during patient exhalation secondary to inadequate trigger sensitivity
Adjustments in the sensitivity of the trigger can substantially improve patient-ventilator synchrony when patient effort is not appropriately being sensed by the ventilator. Flow triggering is generally more sensitive than pressure triggering as a change in flow requires less inspiratory effort than a pressure change. Thus, flow triggering is used most commonly in neonatal and pediatric critical care as a mechanism to improve patient-ventilator synchrony and minimize issues with trigger sensitivity.
Dys-synchrony may also occur when an excessive air leak initiates unwarranted ventilator triggering. In this situation, the ventilator may not be able to distinguish the difference between a decrease in airway flow/pressure due to the excessive air leak and inspiratory effort by the patient. Thus, a large air leak may be misinterpreted by a ventilator as patient effort, resulting in “autocycling” of an inappropriately triggered ventilator breath (i.e., without patient effort). In such situations, the trigger sensitivity setting should be adjusted and/or the air leak addressed.
44.3.3 Flow Dys-synchrony
In addition to trigger insensitivity, inadequate inspiratory flow can lead to patient-ventilator dys-synchrony. Figure 44.4 reveals a patient effort which results in a decrease in airway pressure (arrows); however, as inspiratory flow is limited in this square-wave, constant flow mode of ventilation, the ventilator is unable to deliver the inspiratory flow the patient desires. In this situation, patient effort creates a transient reduction of airway pressure during inspiration, and flow dys-synchrony is demonstrated by a decrease in the airway pressure tracing on the pressure–time scalar. The clinical findings of this “double breath” are typically tachypnea and increased work of breathing, along with overall patient discomfort and agitation. This dys-synchrony may also be similarly seen on the flow-volume loop as indicated by the arrow (Fig. 44.5).
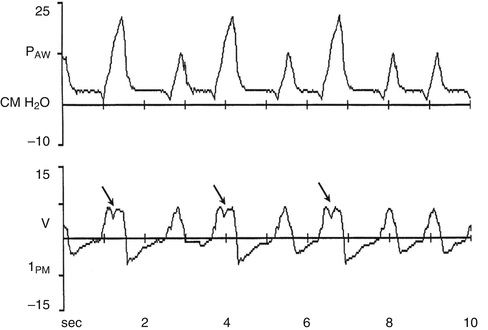
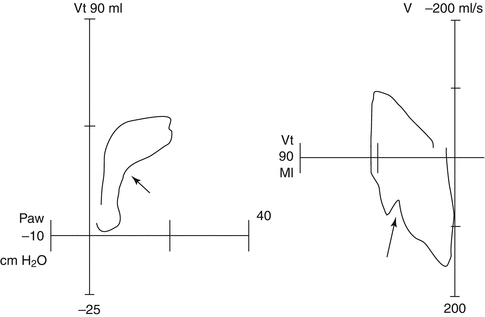
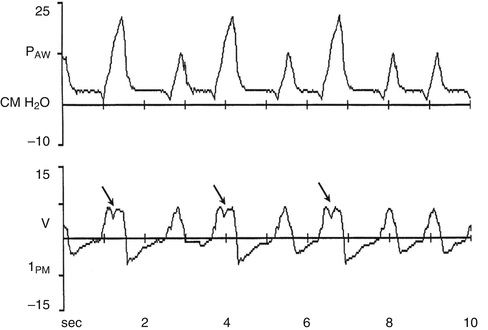
Fig. 44.4
Inspiratory flow dys-synchrony as demonstrated by the flow-time airway scalar. Arrows represent patient effort in the setting of inadequate flow
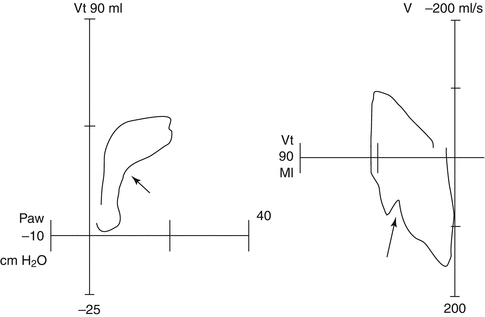
Fig. 44.5
Inspiratory flow dys-synchrony as demonstrated by a flow-volume loop. Arrows represent patient effort secondary to inadequate flow
Increasing the set inspiratory flow rate during square-wave, constant flow ventilation and/or increasing the overall ventilatory support may eliminate flow dys-synchrony; however, such an approach may lead to an unacceptable increase in the peak inspiratory pressure (PIP). In such a situation, changing to an alternate mode of ventilation with variable inspiratory flow may be helpful in improving synchrony. A variable, decelerating inspiratory flow mode will generally meet the inspiratory demand better than a mode with constant inspiratory flow (e.g., volume-limited ventilation).
Although flow dys-synchrony is most commonly seen in constant, square-wave inspiratory flow ventilation (e.g., volume-limited ventilation) as previously described, flow dys-synchrony can occur with decelerating, variable inspiratory flow ventilation when the patient is at the upper limits of the settings chosen (i.e., neonatal or pediatric settings of the ventilator). For example, a large infant with a strong inspiratory demand may demonstrate flow dys-synchrony when the infant ventilator settings are utilized. In this situation, the flow dys-synchrony can be resolved by changing to a pediatric circuit and adapting ventilator settings to increase maximal inspiratory flow.
44.4 Optimizing PEEP
44.4.1 Acute Lung Injury
Acute lung injury (ALI) results in the loss of alveolar stability, alveolar collapse, and decreased pulmonary compliance (Kavanagh et al. 2006; Caironi et al. 2010; Gattinoni et al. 2006; Martin et al. 1992) as seen by a decrease in the slope of the pressure-volume loop (Fig. 44.6). Alveolar collapse may reduce functional residual capacity (FRC) during expiration, if the airway pressures are not adequate to maintain patency of the alveoli at end expiration. In Fig. 44.6, PEEP is inadequate to maintain alveolar patency at end expiration and results in significant atelectasis. During the initial portion of inspiration, re-expansion of collapsed alveoli must occur, and due to the decreased pulmonary compliance, airway pressure must be substantially increased before gas delivery to the alveoli occurs.
