Antifungal Agents
Michael Cohen-Wolkowiez
William J. Steinbach
Daniel K. Benjamin Jr.*
*Dr. Benjamin receives support from the United States Government for his work in pediatric and neonatal clinical pharmacology (1R01HD057956-02, 1R01FD003519-01, 1U10-HD45962-06, 1K24HD 058735-01, and Government Contract HHSN267200700051C), the non profit organization Thrasher Research Foundation for his work in neonatal candidiasis (http://www.thrasherresearch.org), and from industry for neonatal and pediatric drug development (http://www.dcri.duke.edu/research/coi.jsp)
Because of advances in aggressive chemotherapeutic agents and frequent use of organ transplantation, fungal pathogens are an expanding complication in immunocompromised patients (1). Fortunately, the therapeutic armamentarium for invasive fungal infections has markedly increased in the last decade and more importantly both regulatory agencies and industry have worked cohesively to evaluate the use of these agents in the pediatric population. We will review the major systemic antifungal agents in clinical use or advanced testing (Table 35.1), excluding those antifungal agents that presently remain in the early stages of development (i.e., sordarins, pradimicins, or benanomycins).
Polyenes
Amphotericin B
Mechanism of Action
The oldest antifungal class is the polyene macrolides, amphotericin B and nystatin. Since its initial approval for use in 1958, amphotericin B remains the “gold standard” for invasive fungal infection treatment as well as the standard of comparison for all newer antifungal agents. However, the fact that amphotericin B remained at such a post was not solely by virtue of its effectiveness but rather due to the lack of alternatives until recent studies (2). Amphotericins A and B are natural fermentation products of a soil actinomycete collected in Venezuela in 1953, but although each has antifungal properties, amphotericin A was not developed (3). Amphotericin B is so named because it is amphoteric, forming soluble salts in both acidic and basic environments (4). However, because of its insolubility in water, amphotericin B for clinical use is actually amphotericin B mixed with the detergent deoxycholate in a 3:7 mixture (4,5).
The polyenes bind to ergosterol, the major sterol found in fungal cytoplasmic membranes. The lipophilic amphotericin B acts by preferential binding to fungal membrane ergosterols, creating transmembrane channels, which result in an increased permeability to monovalent cations. The fungicidal activity is believed to be due to a damaged barrier and subsequent cell death through leakage of essential nutrients from the fungal cell. Amphotericin B also has oxidant activity that disrupts cellular metabolism (6), inhibits proton adenosine triphosphatase (ATPase) pumps, depletes cellular energy reserves, and promotes lipid peroxidation to result in an increase in membrane fragility and ionized calcium leakage (5,7,8).
Pharmacology
Amphotericin B is released from its carrier and distributes very efficiently (>90%) with lipoproteins, taken up preferentially by organs of the reticuloendothelial system, and follows a three-compartment distribution model. There is an initial 24- to 48-hour distributional half-life reflecting uptake by host lipids, very slow release and excretion into urine and bile, and a subsequent terminal elimination half-life of up to 15 days (9). In a small series (n = 13) evaluating the pharmacokinetics of amphotericin B among premature infants (27.4 ± 5 weeks), 9 subjects showed elimination of amphotericin B at steady state with an estimated elimination half-life of 14.8 hours (5 to 82). The rest of the infants, however, showed minimal drug elimination during the dosing interval suggesting substantial drug accumulation and interindividual variability (10).
Experimental in vitro and in vivo studies support concentration-dependent killing with a prolonged postantifungal effect, suggesting that large daily doses will be most effective and achieving optimal peak concentrations is important (11). Peak levels are achieved 1 hour after a 4-hour infusion and reach a plateau at the third consecutive day of a constant dose (4). There is a relationship between total dose administered and tissue concentrations, suggesting a progressive accumulation with continued drug administration (12). However, there is no evidence of a clinical dose effect (13) to support higher doses (>1 mg per kg per day) of amphotericin B (14). In adults, cerebrospinal fluid (CSF) values are only 2% to 4% of serum
concentrations and sometimes difficult to detect (15). In a small series of premature infants born at 27.4 (±5) weeks’ gestational age (n = 5), however, CSF amphotericin B concentrations were 40% to 90% of serum concentrations obtained simultaneously (10).
concentrations and sometimes difficult to detect (15). In a small series of premature infants born at 27.4 (±5) weeks’ gestational age (n = 5), however, CSF amphotericin B concentrations were 40% to 90% of serum concentrations obtained simultaneously (10).
Table 35.1 Formulations of Selected Systemic Antifungal Agents | ||||||||||||||||||||||||||||||||||||
---|---|---|---|---|---|---|---|---|---|---|---|---|---|---|---|---|---|---|---|---|---|---|---|---|---|---|---|---|---|---|---|---|---|---|---|---|
|
In addition to conventional amphotericin B deoxycholate, three fundamentally different lipid-associated formulations have been developed that offer the advantage of an increased daily dose of the parent drug, better delivery to the primary reticuloendothelial organs (lungs, liver, spleen) (16,17), and reduced toxicity. The US Food and Drug Administration (FDA) approved amphotericin B lipid complex (ABLC) in December 1995, amphotericin B colloidal dispersion (ABCD) in December 1996, and liposomal amphotericin B (L-amphotericin B) in August 1997 (18).
ABLC (Abelcet, Enzon Corporation, Bridgewater, NJ) is a tightly-packed ribbon-like structure of a bilayered membrane formed by combining dimyristoyl phosphatidylcholine, dimyrisotyl phosphatidylglycerol and amphotericin B in a ratio of 7:3:3. ABCD (Amphocil, AstraZeneca, London; or Amphotec, Intermune Pharmaceuticals, Brisbane, CA) is composed of disk-like structures of cholesteryl sulfate complexed with amphotericin B in an equimolar ratio. L-amphotericin B (AmBisome, Fujisawa Healthcare, Inc., Deerfield, IL), the only “true liposomal” product, consists of small uniformly sized unilamellar vesicles of a lipid bilayer of hydrogenated soy phosphatidylcholine-distcaryl phosphatidylglycerol-cholesterol-amphotericin B in the ratio 2:0.8:1:0.4 (19,20).
Table 35.2 Spectrum of Activity of Selected Antifungal Agents | ||||||||||||||||||||
---|---|---|---|---|---|---|---|---|---|---|---|---|---|---|---|---|---|---|---|---|
|
Lipid formulations of amphotericin B generally have a slower onset of action and are less active than amphotericin B in time-kill studies, presumably due to the required disassociation of free amphotericin B from the lipid vehicle (21). It is postulated that activated monocytes/macrophages take up drug-laden lipid formulations and transport them to the site of infection where phospholipases release the free drug (18,22). The different pharmacokinetics and toxicities of the lipid formulations are reflected in the dosing recommendations: ABLC is recommended at 5 mg per kg per day, ABCD at 3 to 5 mg per kg per day, and L-amphotericin B at 1 to 5 mg per kg per day. However, most clinical data have been obtained with the use of these preparations at 5 mg per kg per day. Animal studies clearly indicate that on a similar dosing schedule the lipid products are almost always not as potent as amphotericin B, but that the ability to safely administer higher daily doses of the parent drug improves their efficacy (15) and they compare favorably with the amphotericin B deoxycholate preparation with less toxicity. A multicenter maximum tolerated dose study of L-amphotericin B using doses from 7.5 to 15 mg per kg per day
found a nonlinear plasma pharmacokinetic profile with a maximal concentration at 10 mg per kg per day and no demonstrable dose-limiting nephrotoxicity or infusion-related toxicity (23).
found a nonlinear plasma pharmacokinetic profile with a maximal concentration at 10 mg per kg per day and no demonstrable dose-limiting nephrotoxicity or infusion-related toxicity (23).
Lipid formulations have the added benefit of increased tissue concentration compared with conventional amphotericin B, specifically the liver, lungs, and spleen. However, it is not entirely clear whether these higher concentrations in tissue are truly available to the microfoci of infection. L-amphotericin B has a comparatively higher peak plasma level and prolonged circulation in plasma (19), whereas ABCD has a lower plasma level than amphotericin B after infusion but a longer half-life and larger volume of distribution (24).
Several reviews have focused on amphotericin B pharmacokinetics in children. In one study of five premature infants and five older children the volume of distribution was smaller and the elimination clearance more rapid than previously reported in adults. Serum levels were approximately half of those in adults with comparable doses, and interpatient variability was marked in the premature infants (25). Other studies have confirmed interpatient variability in pediaic patients including lower serum levels in smaller children with higher total clearance, indicating perhaps that they received too low of a dose. In addition, older, heavier children with a relatively higher exposure to amphotericin B may be overdosed and at greater risk for toxicity (26,27). One study examined ABLC in six children with hepatosplenic candidiasis and showed that steady state was reached in 7 days with continued resolution of lesions even after drug discontinuation (28). A population PK analysis of 39 pediatric patients with cancer aged an average of 6.5 years (0.17 to 17) weighing an average of 21.1 kg (6.1 to 84.1) receiving 0.8 to 5.9 mg per kg of body weight per day of L-amphotericin B found that body weight exhibits a significant influence in its pharmacokinetic parameters. The estimated clearance and volume of distribution in the central compartment in this population was 0.44 L per hour and 3.12 L, respectively. Through simulations of doses ranging from 1 to 12.5 mg per kg per day it was found that infants weighing less than 20 kg may require higher doses than heavier children (29). Weight was also the most influential predictor of population pharmacokinetic parameters among neonates [median weight 1.06 kg (0.48 to 4.9); median gestational age, 27 weeks (24 to 41)] with invasive candidiasis treated with ABLC at 2.5 to 5 mg per kg of body weight for a median of 21 days (4 to 47 days). In this population, postnatal and gestational age did not influence clearance or volume of distribution of ABLC (30).
Toxicities, Side Effects, and Drug Interactions
Tolerance to amphotericin B deoxycholate is limited by its acute and chronic toxicities. In addition to fungal ergosterol the drug also interacts with cholesterol in human cell membranes, which likely accounts for its toxicity (31). Up to 80% of patients receiving amphotericin B develop either infusion-related toxicity or nephrotoxicity (4), especially with concomitant therapy with nephrotoxic drugs such as aminoglycosides, vancomycin, cyclosporine, or tacrolimus (32,33). Amphotericin B also has a constrictive effect on the afferent and efferent renal arterioles, leading to a reduction in the glomerular filtration rate (34).
Renal function usually returns to normal after cessation of amphotericin B, although permanent renal impairment is common after larger doses (2). Amphotericin B nephrotoxicity is generally less severe in infants and children than in adults, likely due to the more rapid clearance of the drug in children. Lipid formulations appear to stabilize amphotericin B in a self-associated state so that it is not available to interact with the cholesterol of human cellular membranes (19,35). Another theory for the decreased nephrotoxicity of lipid formulations is the preferential binding of its amphotericin B to serum high-density lipoproteins compared with amphotericin B’s binding to low-density lipoproteins (36). The high-density lipoprotein-bound amphotericin B appears to be released to the kidney more slowly, or to a lesser degree. For infusion-related toxicity there is a general agreement that L-amphotericin B has less toxicity than ABLC whereas ABCD appears closer in toxicity to conventional amphotericin B (37,38).
Clinical Studies
The recommended dose of amphotericin B is 1.0 to 1.5 mg per kg per day and optimal duration of therapy is unknown but largely dependent on underlying disease, extent of the patient’s fungal infection, resolution of any neutropenia, lessening immunosuppression, and the return of graft function following bone marrow or organ transplantation (39,40). There is no total dose of amphotericin B recommended, and the key to success has been suggested to give high doses in the initial phase of therapy and reduce the dose if toxicity develops (41). According to the FDA, all three lipid formulations are currently indicated for patients with systemic mycoses, primarily invasive aspergillosis, who are intolerant of or refractory to conventional amphotericin B. In addition, L-amphotericin B is approved as empiric therapy for the neutropenic patient with persistent fever, despite broad-spectrum antibiotic therapy (17). Many experts also suggest possible initial therapy with lipid amphotericin B formulations in patients with marginal renal function or those receiving nephrotoxic drugs.
There are no data or consensus opinions among authorities indicating improved efficacy of any new amphotericin B lipid formulation over conventional amphotericin B (17,18,34,38,42). One exception is that L-amphotericin B has shown less frequent infusion-related adverse events than the other lipid formulations and conventional amphotericin B (18). This fact leaves the clearest indication for a lipid formulation over amphotericin B to be reducing nephrotoxicity, which is an important clinical feature in complex patients with invasive mycoses.
Pediatric Clinical Studies
Because amphotericin B is the oldest commonly used systemic antifungal, there are the most pediatric data for this agent. The first review of pediatric antifungal therapy was in 1969 (43) and covered the nephrotoxicity of amphotericin B in 39 pediatric patients. An open-label study of 111 treatment episodes with ABLC in pediatric patients
revealed a well-tolerated drug with generally stable renal function over 6 weeks of therapy. There was a complete or partial therapeutic response in 70% of patients, including 56% for aspergillosis and 81% for candidiasis (44). A retrospective study of 46 pediatric patients who received ABLC confirmed the minimal decline in renal function and reported an overall response rate of 83%, including 78% against aspergillosis and 89% against candidiasis (45).
revealed a well-tolerated drug with generally stable renal function over 6 weeks of therapy. There was a complete or partial therapeutic response in 70% of patients, including 56% for aspergillosis and 81% for candidiasis (44). A retrospective study of 46 pediatric patients who received ABLC confirmed the minimal decline in renal function and reported an overall response rate of 83%, including 78% against aspergillosis and 89% against candidiasis (45).
In a prospective, randomized controlled trial of 49 children who received ABCD versus amphotericin B there was significantly less renal toxicity in the ABCD group (12%) versus the amphotericin B group (52.4%). Although not statistically significant, a greater proportion of children who received ABCD (69%) versus amphotericin B (41%) also had a successful outcome (46). A retrospective analysis of 30 children found that a short course of 7 to 14 days of amphotericin B after the last positive blood culture for Candida species was adequate for treatment in children (47). Prophylactic liposomal amphotericin B administered for the first 100 days post–allogeneic stem cell transplantation in children reduced the incidence of invasive mold infections in a retrospective single center study (48). From 1996 to 2000 the safety and efficacy of ABLC injection was assessed in 548 children with cancer (0 to 20 years of age) who were enrolled in the Collaborative Exchange of Antifungal Research registry. Response data for 89% (255/285) of patients with documented pathogens showed that a complete or partial response was achieved in 54.9% of patients. Among patients with proven Aspergillus infection, the response rates were 37.5% to 40.5%. In this report, elevations in serum creatinine of more than 1.5 and more than 2.5 times baseline values were seen in 24.8% and 8.8% of all patients, respectively. The use of ABLC in pediatric patients with cancer intolerant of or refractory to conventional antifungal therapy is recommended (49).
There is very limited published experience in neonates despite extensive use, and one review of L-amphotericin B in neonates showed that the agent is well tolerated, and renal function previously diminished while on conventional amphotericin B improved while on the liposomal formulation (50).
Pyrimidine Analogues
5-Fluorocytosine
Mechanism of Action
5-Fluorocytosine (5-FC; Ancoban, ICN Pharmaceuticals, Costa Mesa, CA) is a fluorinated analogue of cytosine first synthesized in 1957 as a potential antitumor agent (51), first used to treat human disease in 1968 (52), and initially approved for use in 1972 (17). Unfortunately, 5-FC has little inherent anti-Aspergillus activity (53) and most reports detail clinical failure with monotherapy for yeast infections (54). Its antimycotic activity results from the rapid conversion of 5-FC into 5-fluorouracil (5-FU) within susceptible fungal cells (55,56). The two mechanisms of action of 5-FU are incorporation into fungal RNA in place of uridylic acid to inhibit fungal protein synthesis and inhibition of thymidylate synthetase to inhibit fungal DNA synthesis (55). The latter appears to be the dominant mechanism. Clinical and microbiologic antifungal resistance appears to develop quickly to 5-FC monotherapy, so clinicians have reserved it for combination approaches to augment other more potent antifungals.
Pharmacology
Fungistatic 5-FC is thought to enhance the antifungal activity of amphotericin B, especially in anatomical sites where amphotericin B penetration is often suboptimal such as CSF, heart valves, and the vitreal body (13). 5-FC penetrates well into most body sites because it is small, highly water-soluble, and not bound by serum proteins to any great extent (55). One explanation for the synergism detected with amphotericin B + 5-FC is that the membrane-permeabilizing effects of low concentrations of amphotericin B facilitate penetration of 5-FC to the cell interior (3). Using a Candida albicans model, Beggs and Sarosi suggested that synergism actually results from sequential and not combined action, with amphotericin B acting alone until its gradual oxidation results in its depletion, at which point 5-FC acts on surviving fungal cells’ RNA and DNA synthesis (3,57). 5-FC is available only as an oral formulation in the United States, and the correct dose is 150 mg per kg per day in four divided doses.
Toxicities, Side Effects, and Drug Interactions
The toxicity of 5-FC is hypothesized to be due to its conversion to 5-FU, with reports of patients receiving 5-FC for antifungal treatment having serum 5-FU levels in the range found after chemotherapeutic doses (58). However, a pilot study of six patients found undetectable levels of 5-FU in patients receiving 5-FC therapy, suggesting it unlikely that 5-FC toxicity is indeed caused by 5-FU exposure, as originally hypothesized. However, the patients in this study received 5-FC intravenously, and thereby bypassed the conversion of oral 5-FC to 5-FU by the human intestinal microflora (59).
5-FC may exacerbate myelosuppression in patients with neutropenia and toxic levels may develop when in combination with amphotericin B due to nephrotoxicity of the amphotericin B and the decreased renal clearance of 5-FC (39). Routine serum 5-FC level monitoring is warranted in high-risk patients, since peak serum concentrations of 100 μg per mL or greater (2 hours postdose) are associated with bone marrow aplasia. In a review of a multicenter trial of 194 patients who received amphotericin B + 5-FC for cryptococcal meningitis, one or more toxic drug reactions (including azotemia, renal tubular acidosis, leukopenia, thrombocytopenia) developed in 103 patients (60). Toxicity appeared in the first 2 weeks of therapy in 56% of patients and in the first 4 weeks of therapy in 87% of patients. In a study involving 33 neonates in the United Kingdom treated with intravenous or oral 5-FC who underwent therapeutic drug monitoring, drug concentrations were low (trough, <20 mg per L or peak, <50 mg per L) in 40.5%; undetectable in 5.1%; high (trough level >40 mg per L or peak >80 mg per L) in 38.9%; and potentially toxic (>100 mg per L) in 9.9% (61). Given the narrow therapeutic range, the need for therapeutic drug
monitoring and oral administration in the United States, this drug is seldom used in neonates.
monitoring and oral administration in the United States, this drug is seldom used in neonates.
Clinical Studies
Nearly all clinical studies involving 5-FC are combination antifungal protocols for cryptococcal meningitis, due to the inherently rather weak antifungal activity of 5-FC monotherapy. The first comparative clinical cryptococcal meningitis study with amphotericin B + 5-FC was in 1979 (62) and found a benefit to the combination over amphotericin B alone. One multicenter study of 194 patients with cryptococcal meningitis concluded that 4 weeks of amphotericin B + 5-FC should be reserved for patients without neurologic complications or immunosuppressive therapy, but in a more high-risk population 6 weeks of combination therapy resulted in less relapses (63). Further studies included a multicenter trial that found similar results with consolidation therapy using either itraconazole or fluconazole for 8 weeks after 2 weeks of initial amphotericin B + 5-FC therapy (64).
While other studies have evaluated different combinations for cryptococcal meningitis (65,66,67), amphotericin B + 5-FC is currently recommended as initial therapy for cryptococcal meningitis after substantial study, and it is also suggested for use in candidal meningitis (68). 5-FC fills an important role in conjunction with amphotericin B, as 5-FC will penetrate into the CSF much better compared with amphotericin B’s poor penetration, and 5-FC treated patients had less relapses compared with those receiving only amphotericin B.
Pediatric Clinical Studies
The use of 5-FC in premature neonates is discouraged. A study evaluating risk factors and mortality of neonatal candidiasis among extremely premature infants showed that infants with Candida meningitis who received amphotericin B in combination with 5-FC had a prolonged time to sterilize the CSF compared with those receiving amphotericin B monotherapy (median of 17.5 vs. 6 days, respectively) (69).
Azoles
The azoles are subdivided into imidazoles and triazoles on the basis of the number of nitrogens in the azole ring (7), with the structural differences resulting in different binding affinities for the cytochrome P450 (CYP) enzyme system. With the exception of ketoconazole, the imidazoles have been limited to treatment of superficial mycoses and none, including ketoconazole, have activity against the mold Aspergillus. Of the older first-generation triazoles, fluconazole is also ineffective against Aspergillus, but itraconazole does possess activity against Aspergillus. Newer second-generation triazoles (voriconazole, posaconazole, and ravuconazole) are modifications of prior triazoles with an expanded antifungal spectrum of activity and generally lower minimum inhibitory concentration values compared to the older compounds (70).
Mechanism of Action
The azole antifungals are heterocyclic synthetic compounds that inhibit the fungal cytochrome P45014DM (also known as lanosterol 14α-demethylase), which catalyzes a late step in ergosterol biosynthesis. The drugs bind to the heme group in the target protein and block demethylation of the C-14 of lanosterol, leading to substitution of methylated sterols in the membrane and depletion of ergosterol. The result is an accumulation of precursors with abnormalities in fungal membrane permeability, membrane-bound enzyme activity, and lack of coordination of chitin synthesis (71,72).
Fluconazole
Pharmacology
Fluconazole (Diflucan, Pfizer Inc., New York, NY) is a bis-triazole that was discovered in 1982 and approved by the FDA for use in treating cryptococcosis and Candida infections in 1990. An in vitro time–kill study showed that the rate of fluconazole fungistatic activity was not influenced by concentration once the maximal fungistatic concentration was surpassed (concentration-independent), which is in contrast to the concentration-dependent fungicidal activity of amphotericin B (71) or caspofungin (73). Fluconazole is well absorbed from the gastrointestinal tract and is cleared predominantly by the renal route as unchanged drug, whereas metabolism accounts for only a minor proportion of fluconazole clearance (74). Binding to plasma proteins is low (12%) (75). Gastric absorption of oral fluconazole is virtually unaffected by pH or the presence of food in the stomach.
Fluconazole is available as either an oral or an intravenous form, and oral fluconazole has a high bioavailability of approximately 90% relative to its intravenous administration. Fluconazole passes into tissues and fluids very rapidly, probably due to its relatively low lipophilicity and limited degree of binding to plasma proteins. Concentrations of fluconazole are 10- to 20-fold higher in the urine than in blood, and drug concentrations in the CSF and vitreous humor of the eye are approximately 80% of those found simultaneously in blood (75). The concentrations of fluconazole in body fluids such as vaginal secretions, breast milk, saliva, and sputum are also similar to those in blood, and the fluid:blood ratio remains stable after multiple doses. There is a linear plasma concentration–dose relationship.
It is clear that simple conversion of the corresponding adult dosage of fluconazole on a weight basis is inappropriate for pediatric patients. A review of five separate fluconazole pharmacokinetic studies in 113 pediatric patients, including 12 premature neonates (74) showed that with the exception of neonates, fluconazole clearance is generally more rapid in children than in adults, with a mean plasma half-life of approximately 20 hours compared with approximately 30 hours in adult patients. Therefore, to achieve comparable exposure in pediatric patients, the daily fluconazole dose needs to be essentially doubled for children older than 3 months. Correct pediatric fluconazole doses should be proportionately
higher than adult doses, generally 10 to 12 mg per kg per day.
higher than adult doses, generally 10 to 12 mg per kg per day.
In neonates the volume of distribution is significantly greater and more variable than in infants and children, and doubling the dose for neonatal patients is necessary to achieve comparable plasma concentrations. The increased volume of distribution is thought to be due to the larger amount of body water found in the total body volume of neonates. However, there is also a slow elimination of fluconazole, with a mean half-life of 88.6 hours at birth, decreasing to approximately 55 hours at 2 weeks of age. This is due to the reduced activity of hepatic enzymes for biotransformation as well as reduced glomerular filtration for the first month of life. As renal function continues to develop, the fluconazole plasma half-life decreases (74); however, a recent population pharmacokinetic study in premature infants suggests that maintenance fluconazole doses of 12 mg per kg per day are necessary to achieve exposures similar to older children and adults (76). In addition, a loading dose of 25 mg per kg would achieve steady-state concentrations sooner than the traditional dosing scheme (77). This strategy is currently being evaluated in a phase I clinical trial.
Children with human immunodeficiency virus (HIV) infection who receive oral fluconazole achieve similar serum concentrations to intravenous dosing and this indicates a nearly complete degree of absorption (78). In fact, there have also been comparable concentrations after either oral or intravenous administration in children younger than 3 months (79). The bioavailability of enteral fluconazole is adequate in even critically ill surgical patients (79). The peritoneal bioavailability of fluconazole is also excellent for treating dialysis patients with peritonitis. A prospective study of 17 children on dialysis found that fluconazole was excreted almost solely through the dialysate and the terminal half-life was significantly longer in the children requiring peritoneal dialysis (80). Finally, there is a theoretical advantage to the oral suspension fluconazole over capsules for oral thrush, as in one study of health volunteers the peak saliva levels of fluconazole were higher in those flushing their mouth for 2 minutes with the oral suspension than in those who swallowed the capsule (81).
Toxicities, Side Effects, and Drug Interactions
Side effects of fluconazole are uncommon. In one study of 24 immunocompromised children, elevated transaminases were observed in only two cases (82). A large review of 78 reports that used fluconazole in a total of 726 children younger than the age of 1 year showed that it was generally well tolerated and reaffirmed the guidelines to increase the interval between doses due to the prolonged elimination during the first month of life (83). Another review of 562 children from 12 clinical studies confirmed that pediatric results mirror the excellent safety profile seen in adults. The most common side effects were gastrointestinal upset (7.7%) (vomiting, diarrhea, nausea) and a skin rash (1.2%) (84). Fluconazole affects the metabolism of cyclosporine, leading to its increased concentration when used together (76).
Clinical Studies
A review of two randomized clinical trials showed that patients who received fluconazole versus placebo for prophylaxis against candidiasis while undergoing allogeneic bone marrow transplantation had lower rates of candidal infection and gut graft-versus-host disease (85). Additional studies revealed that either fluconazole (200 mg per day) versus low-dose amphotericin B (0.2 mg per kg per day) or high dose (400 mg per day) versus low-dose (200 mg per day) fluconazole prophylaxis showed any difference in incidence of fungal infections or survival (86,87).
For treatment of invasive candidiasis, a multicenter trial of 236 patients found that those treated with fluconazole + amphotercin B versus fluconazole alone trended toward better success and more rapid resolution of Candida fungemia with the combination (88). In another randomized trial for treatment of fungal infections using medical and surgical intensive care unit patients, there was a significant decrease in Candida species infections in the group that received fluconazole versus placebo (89).
Unlike itraconazole or ketoconazole, fluconazole is particularly appropriate for urinary tract infections due to its concentrating effect in the bladder. Fluconazole is also effective for superficial skin infections, since the stratum corneum: serum ratio is 37 (90).
Pediatric Clinical Studies
An open, prospective, randomized pilot study in 50 children undergoing remission induction or consolidation chemotherapy showed that those patients who received either fluconazole or nystatin prophylaxis for Candida infections had similar rates of success (91). A study of 40 young infants (aged 2 days to 3 months) with either nonresponse or contraindication to standard antifungal therapy treated with fluconazole showed a 97% clinical and mycological response rate (92). In a prospective, randomized double-blind trial over a 30-month period of 100 infants with birth weights less than 1,000 g, those infants who received fluconazole for 6 weeks had a decrease in fungal colonization (22% vs. 60%) as well as a decrease in the development of invasive fungal infection compared with placebo (0% vs. 20%) (93). A larger prospective, randomized double-blind, controlled trial conducted in nine neonatal intensive care units in Italy among 322 infants with birth weight less than 1500 g showed that a fluconazole prophylaxis regimen of 3 to 6 mg per kg several times per week for 4 to 6 weeks reduced the incidence of Candida colonization [9.8% in the 6 mg group, 7.7% in the 3 mg group, and 29.2% in the placebo group (p < .001)] and invasive fungal infections [2.7% in the 6 mg group (p = .005), 3.8% in the 3 mg group (p = .02), and 13.2% in the placebo group] (94). A retrospective study evaluating the incidence of invasive candidiasis and Candida-related mortality among infants with birth weights less than 1,000 g who received fluconazole prophylaxis (3 mg per kg several times per week) for 6 weeks showed that the incidence of invasive candidiasis and Candida-associated mortality decreased. In the group receiving fluconazole, no increase in fluconazole resistant Candida strains was observed (95). Similarly, another report demonstrated that
the use of fluconazole prophylaxis for 4 to 6 weeks in infants with birth weights less than 1500 g did not increase the incidence of fungal colonization and infections caused by natively fluconazole-resistant Candida species (96). Results of fluconazole prophylaxis studies in premature infants are encouraging; however, the universal implementation of such strategy across nurseries is discouraged because (a) the rate of Candida infections varies greatly among centers (97) and (b) there is insufficient neurodevelopmental follow-up data in these infants to justify prophylaxis (98). A multicenter international trial is underway to answer questions regarding the most appropriate prophylaxis regimen and the need of prophylaxis based on individual nursery systemic Candida infection rates.
the use of fluconazole prophylaxis for 4 to 6 weeks in infants with birth weights less than 1500 g did not increase the incidence of fungal colonization and infections caused by natively fluconazole-resistant Candida species (96). Results of fluconazole prophylaxis studies in premature infants are encouraging; however, the universal implementation of such strategy across nurseries is discouraged because (a) the rate of Candida infections varies greatly among centers (97) and (b) there is insufficient neurodevelopmental follow-up data in these infants to justify prophylaxis (98). A multicenter international trial is underway to answer questions regarding the most appropriate prophylaxis regimen and the need of prophylaxis based on individual nursery systemic Candida infection rates.
Itraconazole
First publicly described in 1983 (99,100) and available for clinical use in 1990, itraconazole (Sporanox, Janssen Pharmaceuticals, Titusville, NJ) adopted a triazole nucleus with higher specificity for the fungal CYP enzyme system than the older imidazoles (101). Itraconazole’s fungicidal activity is not as efficient as that of amphotericin B because inhibition of sterol synthesis takes longer than directly creating channels within the cell membrane (102). Historically there had been several constraints with itraconazole: no parenteral formulation, erratic oral absorption in high-risk patients, and significant drug interactions. These pharmacokinetic concerns have been addressed with both an intravenous formulation and a better absorbed oral preparation.
Pharmacology
Itraconazole has a high volume of distribution and accumulates in tissues, and the tissue-bound levels are probably more clinically relevant to infection treatment than serum levels (72). Itraconazole is poorly water-soluble, not reliably absorbed from the gastrointestinal tract in its capsule formulation, and has high protein binding (11). It has a relatively long half-life of 25 to 50 hours, which allows for once-daily dosing (103).
Dissolution and absorption of itraconazole are affected by gastric pH. Patients with achlorhydria or H2-receptor antagonist use may demonstrate impaired absorption, whereas coadministration of the capsule with acidic beverages such as colas or cranberry juice may enhance its absorption (104). Administering a dose with food significantly increases the absorption of the capsule formulation, but the new oral suspension with a cyclodextrin base is better absorbed on an empty stomach (15). Elimination of itraconazole is primarily hepatic, so there is no need for dosage adjustment in renal failure (72).
To overcome problems with variable absorption, itraconazole has now been solubilized in cyclodextrin, with its substantial improvement in absorption of drug as an oral solution (72,105). This allows itraconazole to be available as an oral capsule, oral solution, and intravenous formulation. Cyclodextrins are naturally occurring doughnut-shaped glucose oligomers produced by enzymatic degradation of starch that have been chemically modified for medical use. The external face of the molecule is hydrophilic to facilitate solubilization of the complex in water and shield a lipophilic guest molecule (106). Once itraconazole is released from the host cyclodextrin it follows its standard pharmacokinetics, with steady-state concentration reached in the second week of daily dosing.
A new intravenous formulation of itraconazole was approved by the FDA for pulmonary and extrapulmonary aspergillosis in patients who are intolerant of or refractory to amphotericin B (107). The intravenous formulation can rapidly achieve high steady-state plasma concentrations (108,109) as opposed to the 7- to 10-day period needed for the capsule or oral formulation (110). In children, itraconazole oral solution produces a maximum concentration lower than in adults which could potentially justify higher dosing in younger children, whereas other pharmacokinetic properties such as half-life are similar to values in adults (111). The intravenous preparation is not recommended for patients with reduced renal clearance because the impact or toxicity of the cyclodextrin is not known. In a single dose (2.5 mg per kg) pharmacokinetic study of itraconazole in children aged 7 months to 17 years (n = 33), pharmacokinetic parameters of itraconazole were highly variable across age groups and, therefore, no age dependence was observed in drug disposition. Overall, the estimated Cmax was 1,015 (±692) ng per mL, systemic clearance was 702.8 (±499.4) mL per hour per kg, and half-life was 20.2 (±12.8) hours (112). Pediatric dosing for itraconazole is not exact, but generally felt to be higher than adult doses and approximately 3 to 10 mg per kg per day.
Toxicities, Side Effects, and Drug Interactions
Side effects are relatively few and include nausea and vomiting (10%), elevated transaminases (5%), (113) and peripheral edema. Although it has not been shown in children, there have been reports of development of cardiomyopathy while receiving this azole. As a potent inhibitor of the fungal CYP3A4 enzyme, itraconazole also has affinity for the human enzyme, and this produces important drug interactions. Prior or concurrent use of rifampin, phenytoin, carbamazepine, and phenobarbital should be avoided. Any drug handled by this cytochrome pathway with normally low bioavailability, extensive first-pass metabolism, or a narrow therapeutic window may be especially vulnerable (114).
Patients receiving cyclosporine who are treated with a triazole should have an immediate dose reduction of cyclosporine followed by frequent monitoring of serum levels. In one study there was an increase in tacrolimus or cyclosporine levels 48 hours after completing an intravenous itraconazole loading dose, so a 50% tacrolimus or cyclosporine dose reduction is recommended after an intravenous itraconazole loading dose (115). Furthermore, the azoles may also increase serum levels and intracellular levels of cytotoxic drugs such as vincristine and anthracyclines (31) or protease inhibitors (116). Azole–drug interactions may also lead to decreased plasma concentration of the azole, related to either decreased absorption or increased metabolism (17).
Although the theoretical concern of polyene–azole antagonism due to ergosterol mechanisms of action exists,
combination with amphotericin B does appear safe in a recent survey of clinical practice of 93 invasive-aspergillosis patients treated with this sequential therapy (117).
combination with amphotericin B does appear safe in a recent survey of clinical practice of 93 invasive-aspergillosis patients treated with this sequential therapy (117).
Clinical Studies
Itraconazole has roles in the therapy of numerous fungal infections. A multicenter open-label study was performed in 31 patients with pulmonary invasive aspergillosis who received 14 days of intravenous itraconazole followed by 12 weeks of capsules. The intravenous form was well tolerated and target therapeutic concentrations were obtained within 2 days in 91% of patients and in all patients within 1 week of intravenous treatment. These levels were also maintained after switching to oral therapy, and a complete or partial response was seen in 48% (15/31) of patients (108).
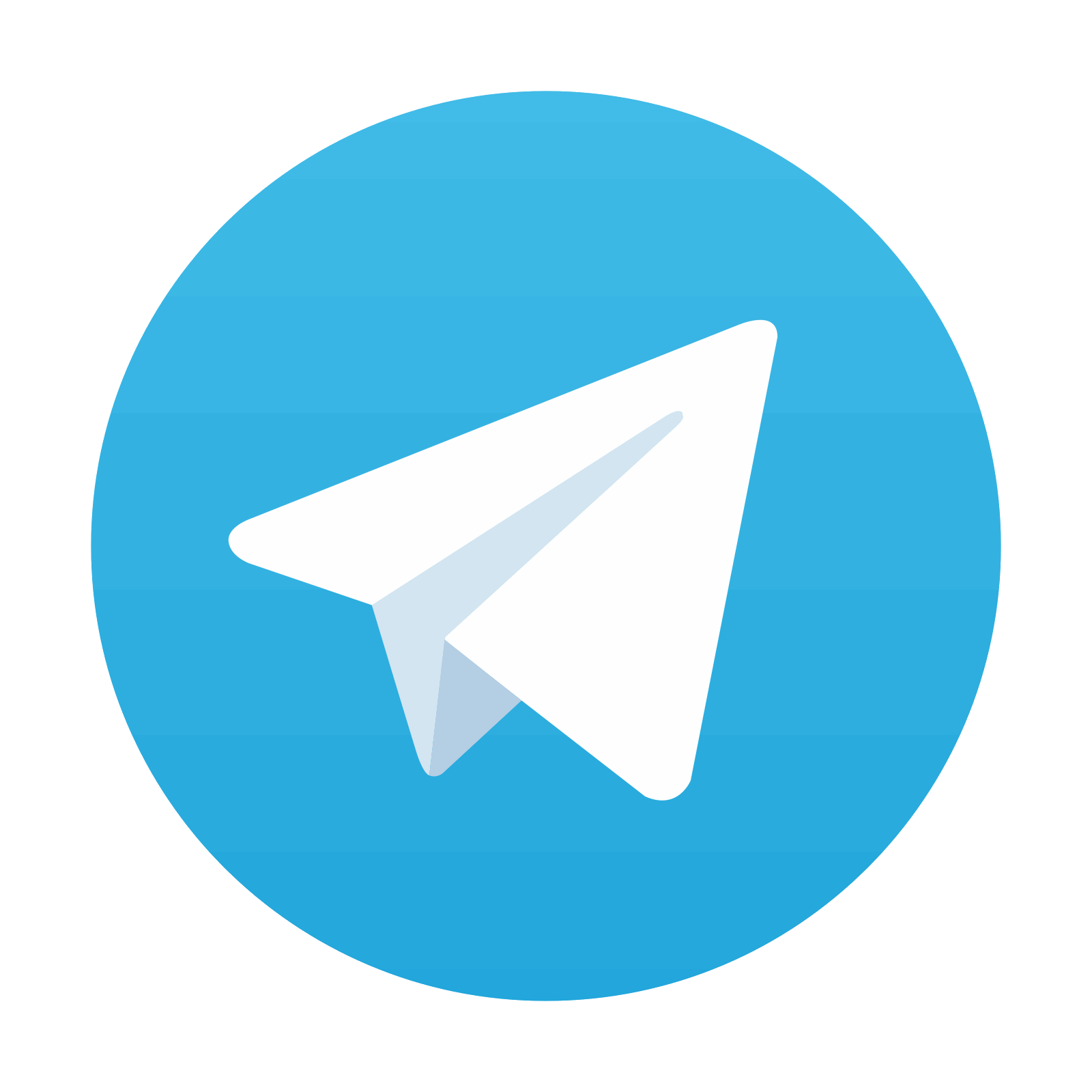
Stay updated, free articles. Join our Telegram channel

Full access? Get Clinical Tree
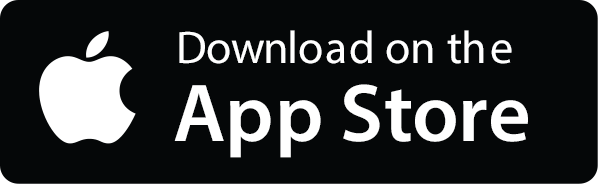
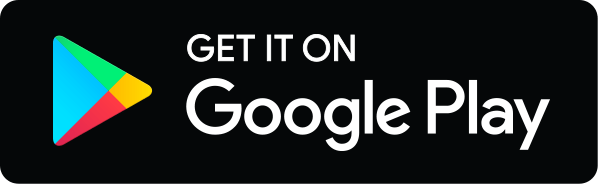
