Antiasthmatics
Hengameh H. Raissy
H. William Kelly
Stanley J. Szefler
Introduction
Asthma is the most common chronic disease among children in the United States affecting about 9 million children younger than 18 years (1). In 2005, 8.95% of children had a current diagnosis of asthma, and an asthma attack was reported by about 4 million children in the previous year (1). Twenty-five percent of emergency room visits are for asthma (2) and children will account for 44% of the asthma hospitalizations (3). The prevalence rate of asthma is highest in children 5 to 17 years old at 9.6% (4) and resulted in 12.8 million missed schooldays by this age group in 2003 (5). Although the rate of asthma is greater in boys than in girls younger than 11 years old, it is about equal during puberty (4). The prevalence of asthma is higher in African Americans, 20%, compared with whites in 2006 (6). In addition, the rate of hospitalization in African Americans is three times higher and they are 2.5 times more likely to die because of asthma (4).
The rate of asthma in children younger than 5 years has increased more than 160% in years 1980 to 1994 (7), and it has been estimated that the number of patients with asthma will increase to more than 100 million by 2025 (8). In spite of increased prevalence of asthma in the United States, hospital admission and mortality of asthma have slightly decreased in the last few years (4). However, 80% to 90% of the asthma deaths can be prevented (9).
Asthma is a multifactorial and complex disease. The National Asthma Education and Prevention Program’s Expert Panel Report 3 (EPR3): Guidelines for the Diagnosis and Management of Asthma defines asthma as “a common chronic disorder of the airways that is complex and characterized by variable and recurring symptoms, airflow obstruction, bronchial hyperresponsiveness, and an underlying inflammation.” The airways inflammation has been demonstrated in the central and peripheral airways, and it involves activation of all airway cells including eosinophils, T lymphocytes, macrophages, mast cells, bronchial smooth muscle, epithelial cells, and fibroblasts (9). These activated cells further regulate airway inflammation and release cytokines and growth factors leading to chronic inflammation, airway remodeling, airflow obstruction, bronchial hyperresponsiveness, and increased risk of asthma exacerbations. Although the exact etiology of the inflammatory process leading to asthma is not well defined, innate immunity (the balance between Th-1 and Th-2 cytokine response), genetics, and environmental factors seem to interact for different asthma phenotypic expressions (10).
The developing immune system begins in the early weeks of gestation, going through several steps of maturation up until and beyond birth. However, because of this immaturity and continual development of the immune system, asthma per se is not observed in the neonate. Approximately 80% of childhood asthma develops before the age of 6 years (10), and may present differently among children. The asthma phenotype differentiates between transient wheezers and persistent wheezers. Transient wheezers (wheezing only at <3 years old) are not at an increased risk for developing asthma later in life; persistent wheezers or late onset wheezers (wheezing only between 3 and 6 years old) are at greater risk for development of asthma later in life (11). Interestingly, longitudinal studies have shown that a decline in lung function growth occurs by age of 6 with the most deficits in children with the onset of symptoms before age of 3 (11). Recently, a positive asthma predictive index has been developed to identify children younger than 3 years with high risk of developing persistent asthma later in life. The risk factors are either one of the following—parental history of asthma, a physician diagnosis of atopic dermatitis, or evidence of sensitization to aeroallergens—or two of the following: evidence of sensitization to foods, 4% or more peripheral blood eosinophilia, or wheezing apart from colds (9,12). In general, a decline in lung growth has not been noted in children, 5 to 12 years of age with mild or moderate persistent asthma through 11 to 17 years of age except for a subset of children (13).
Asthma begins early in life and what determines the severity of asthma or its persistent versus intermittent characteristics remains yet to be determined. It has consistently been shown that asthma is a chronic inflammatory disease regardless of its severity, and the therapy has been focused on the prevention and suppression of inflammation. The current therapeutic options are use of long-term controller medications to manage underlying inflammation and use of rescue medications to manage acute exacerbations (9). The EPR3 provides recommendations for assessment of severity and control, which require assessing both impairment
and risk domains in children aged 0 to 4 years and 5 to 11 years (Table 40.1) (9). The EPR3 also provides guidance on classifying severity based on the level of therapy required to control asthma (Table 40.2) (9). The steps of therapy recommended by EPR3 for infants and young children are provided in Figure 40.1 (9). Adolescents are treated as adults and not presented here (9). This chapter will provide the pharmacologic basis for those recommendations.
and risk domains in children aged 0 to 4 years and 5 to 11 years (Table 40.1) (9). The EPR3 also provides guidance on classifying severity based on the level of therapy required to control asthma (Table 40.2) (9). The steps of therapy recommended by EPR3 for infants and young children are provided in Figure 40.1 (9). Adolescents are treated as adults and not presented here (9). This chapter will provide the pharmacologic basis for those recommendations.
Aerosol Delivery in Children
The most effective antiasthmatics are delivered as aerosols, and delivery of the aerosols into the airways of children is the primary determinant of dose, but like all dosage forms, aerosol delivery presents many unique challenges depending on the age of the child (9). There are three systems of aerosol delivery in clinical practice: (1) nebulizers that generate aerosol clouds by forcing air and fluid up through adjacent open-ended tubes that strike a baffle at high speed creating the aerosol (jet nebulizer) and ultrasonic nebulizers that produce an aerosol by vibrating liquid above a transducer; (2) metered-dose inhalers (MDIs) that force a propellant and solution or micronized suspension of drug stored in a pressurized canister out through a small orifice by opening a stem valve creating an aerosol of particles that diminish in size as the propellant evaporates; and (3) breath-activated, dry-powder inhalers (DPIs) that contain either individually packed micronized doses of drug plus excipients such as lactose or a compact bulk of drug from which micronized doses are shaven off prior to inhalation (they are called “breath activated” because inhalation by the patient creates the aerosol) (14). Because of these differing mechanisms for aerosol production, each device creates different challenges in children.
Device-Specific Issues
The primary determinant of potential delivered dose from each of the systems is particle size generated. All devices produce heterodispersed aerosol clouds that are characterized by their mass median aerodynamic diameter (MMAD). In spontaneously breathing adults, particles greater than 10 μm deposit in the oropharynx, those between 5 and 10 μm deposit in the trachea and large bronchi, particles 1 to 5 μm deposit in the lower airways (often called “the respirable fraction”), and particles smaller than 0.5 μm act as a gas and can be exhaled (14). Very few of the delivery devices generate aerosols with the same MMAD, thus significant differences in the percentage of drug dose delivered to the lung occur both across devices (i.e., jet nebulizer vs. DPI) and within devices (MDI vs. MDI) across the manufacturers. The labeling of doses is also dependent on the type of device but is not dependent on actual dose delivered to the lung. The dosing for nebulizers is based upon the amount of drug put into the nebulizer. The dose for MDIs is based upon the amount of drug that reaches the patient’s mouth (leaves the actuator) following actuation in the United States and the amount that exits the canister in most of the rest of the world. The dose of a DPI is that dose that is available for inhalation following activation of the dose (puncturing the blister, breaking the capsule, or shaving off the dose).
The most frequently prescribed delivery device is the MDI due to its portability and convenience. Recently, the MDI has undergone significant changes due to the Montreal Protocol to eventually end the use of ozone depleting chlorofluorocarbons (9). The introduction of hydrofluoroalkanes (HFAs) as the propellant has been associated with reformulation of some of the inhaled corticosteroids (ICSs) as solutions instead of micronized suspensions (14,15). The reformulation in concert with valve stem and actuator redesign has resulted in products with significantly reduced MMADs markedly improving lung delivery. For example, HFA-propelled beclomethasone dipropionate has a lung delivery in adults of 50% to 60% versus 4% to 10% delivery for the older chlorofluorocarbon-propelled preparations (16). However, other HFA-MDIs such as albuterol, fluticasone propionate, and budesonide/formoterol combination are still formulated as micronized suspensions and deliver 10% to 25% of the actuated dose (9).
The MDI is the most complicated system to use for patients. It requires multiple steps and good coordination of actuation during a slow deep inhalation. Many patients have significant difficulty coordinating actuation and inhalation reducing delivery of drug from MDIs (9,14). Spacer devices, particularly valved holding chambers (VHCs), have been developed to overcome the inability to coordinate actuation and inhalation. The VHCs are attached to the mouthpiece of an MDI, and the valve allows flow out of the VHC during inhalation and not into the VHC during exhalation (14). This allows young patients to breathe normally through a mouthpiece or a snug fitting face mask while an adult actuates the MDI. Although this method of delivery has proven to be efficacious in numerous clinical trials, it is noteworthy that currently no MDI + VHC combination has been approved by the US Food and Drug Administration (FDA) (12,17,18). The various VHCs also produce significant differences in delivery of drug that is drug and device dependent. For example, a VHC may improve delivery from one MDI product and decrease delivery from another MDI product, or two different VHCs may result in significantly different deliveries from the same MDI product (14,19). When using VHCs, it is important to actuate the inhaler only once before taking the inhalation, as actuating multiple times into the device reduces delivery due to coalescence of the particles (14,20). Last, the older plastic VHCs could develop static electricity on the walls over time resulting in attraction of the smaller respirable particles decreasing drug availability (20). This could be reduced by rinsing the devices with dilute home dishwashing detergent weekly or by using the newer VHCs with antistatic lining (21).
The DPIs were developed in response to the Montreal Protocol, but the FDA has determined that they are not considered an alternative to chlorofluorocarbon MDIs. However, they continue to be developed and are probably the easiest to teach patients to use and are associated with fewer mistakes by patients (14). The DPIs require a different inhalation technique that is rapid and deep and most produce optimal delivery at inspiratory flows 60 L per minute or more (14). The rapid deep inhalation, while not optimal for delivery of drug into peripheral airways, helps to break up the larger drug particle aggregates. The effect of inspiratory flow is dependent upon the DPI. For
example, the Pulmicort® Flexhaler™, with a higher internal resistance, has a reduction in respirable particles of 50% at an inspiratory flow of 30 L per minute compared with that of 60 L per minute, whereas with the Asmanex® Twisthaler™, the amount of drug emitted from the device was constant at inspiratory flows of 30 to 70 L per minute (22,23).
example, the Pulmicort® Flexhaler™, with a higher internal resistance, has a reduction in respirable particles of 50% at an inspiratory flow of 30 L per minute compared with that of 60 L per minute, whereas with the Asmanex® Twisthaler™, the amount of drug emitted from the device was constant at inspiratory flows of 30 to 70 L per minute (22,23).
Table 40.1 Assessing Asthma Control and Adjusting Therapy in Children (9) | |
---|---|
|
Table 40.2 Classifying Asthma Severity and Initiating Therapy in Children (9) | |
---|---|
|
![]() Figure 40.1. The stepwise approach for management of asthma in children (9). |
Nebulizers are approved as medical devices and so do not have as stringent criteria for approval as generic drugs. They only need to be as good as any product that is already marketed (14). Jet nebulizers require a source of compressed air and so are not as portable as either an MDI or DPI. Each nebulizer has optimal operating conditions but in general an airflow of 5 to 12 L per minute produces aerosol clouds with an MMAD of 4 to 8 μm (14,17). Ultrasonic nebulizers produce similar delivery as jet nebulizers with the exception of micronized suspensions for which they are ineffective (14). Once nebulizers are set up, they are the simplest for the patient, as they require only normal tidal breathing and so are often favored for young infants who use them with a face mask.
Patient-Specific Issues
Infants and young children clearly have significant differences in airway geometry so that data from normal adults do not necessarily apply, but because of logistical and ethical considerations, radiolabeled aerosol studies are rare in children. However, the adult values for respirable particles are used as a measuring stick for the development of aerosol delivery devices, all of which are designed for adults and then used in children. Many children 5 years of age or younger cannot coordinate inhalation and actuation with MDIs, and children 4 years of age or younger often require the use of a face mask for delivery from both nebulizers and MDI + VHC (14,17,18). The use of a face mask reduces delivery to about one-half that of a mouthpiece as infants are obligate nose breathers, and part of the dose is filtered out by the nose (24). Infants also have lower tidal volumes (tidal volume is a relatively constant 7 to 10 mL per kg throughout life). Infants weighing less than 15 kg will not necessarily be able to completely empty the VHC; however, they may receive higher μg per kg doses based on lack of air entrainment on inhalation (25). So there are a number of factors that can have potentially offsetting effects on aerosol delivery in infants compared with older children. For example, in in vitro models, tidal volumes for those 2 years or younger versus those for 4- to 5-year-olds show higher microgram per kilogram doses delivered to those aged 2 years or younger (26,27,28). However, a wait time between actuation and inhalation of 5 seconds decreases delivery by 30% to 50% (20,27). Finally, the child should be breathing quietly and not crying as delivery by both nebulizer and MDI + VHC in the crying infant reduces delivery by as much as 80% (24). Unfortunately, if infants and young children resist the use of a face mask, parents and caregivers revert to a “blow-by” method, where they hold the mask or end of the tube connected to the nebulizer close to the infants’ mouth and nose. However, studies have shown that holding the mask 1 cm from the face can reduce delivery 50% to 60% and at 2 cm as much as 85% (25). In clinical trials, superiority has not been established for MDI + VHC over nebulization (17,18).
Unfortunately, many children aged 5 years or younger cannot attain a peak inspiratory flow of 60 L per minute (14). The internal resistance of the DPI can also affect the ability of children to generate flow through the device. In one study, 78% of 4-year-olds could generate an inspiratory flow of at least 30 L per minute through a Diskus™, 89% through an Aerolizer™, but only 56% through a Turbuhaler™ (29). The use of DPIs should be reserved for children aged 4 years or older.
Inhaled Corticosteroids
Mechanism of Action
Corticosteroids are the most potent anti-inflammatory agents available for management of asthma. They have a broad array of anti-inflammatory effects. The glucocorticoid receptor (GR) resides in the cytoplasm of cells and is ubiquitous in tissues and cells throughout the body explaining its myriad effects. The corticosteroid enters the cell cytoplasm via passive diffusion across the cell membrane and binds with the inactive GR complex, dissociating the GR complex from heat shock proteins and immunophilin (30). Then the activated GR translocates into the nucleus where it binds to DNA at specific sequences called glucocorticoid response elements where it promotes the production of anti-inflammatory proteins such as lipocortin-1, β2-adrenergic receptor (β2-AR), secretory leukocyte inhibitory protein, and 1κB-α (31). In addition, it inhibits transcription activation of many proinflammatory cytokines that are activated through tumor necrosis factor-α and nuclear factor-κB (i.e., interleukins 1–6, 8, 11, 12, 13; chemokines; matrix metalloproteinase-9; adhesion molecules; inducible nitric oxide synthase; endothelin-1) (30,31). Although there is a single gene encoding the human GR, splice variants have been identified and one, the GRβ isoform, although usually inactive, has been reported to be increased in corticosteroid-resistant patients (30).
The effects of corticosteroids are cell and tissue specific which explain many of their clinical effects. Corticosteroids reduce mucus secretion, produce vasoconstriction reducing airway mucosal blood flow and endothelial cell leaking (31,32). These latter effects, along with reduction in nitric oxide production, decrease vascular and tissue edema that contribute to airways obstruction. It has been posited that the vascular effects of corticosteroids contribute to the acute improvement following frequent high doses of inhaled corticosteroids (ICSs) in patients presenting to the emergency department (33). Corticosteroids inhibit cytokine release from respiratory epithelial cells, macrophages, and T lymphocytes (31). Corticosteroids increase the number of β2-ARs in airway smooth muscle attenuating or modulating tolerance due to chronic β2-agonist stimulation but not completely reversing it (34). Corticosteroids decrease the number of dendritic cells and eosinophils in the airways by inducing apoptosis and reduce the number of circulating eosinophils and reduce the number and activation of T lymphocytes. Corticosteroids do not inhibit the release of soluble mediators from tissue mast cells (histamine and leukotrienes) but reduce their number and influx into airway smooth muscle cells (31). This explains
why acute administration of a corticosteroid has no effect on the early asthmatic response to inhaled allergen or exercise but that chronic administration results in significant attenuation of these responses. As the GR is found throughout all cells and tissues, a potential for significant adverse effects listed in Table 40.3 exists (35). Therefore, corticosteroids with high topical activity with pharmacokinetic properties that limit systemic activity that can be administered by inhalation were developed.
why acute administration of a corticosteroid has no effect on the early asthmatic response to inhaled allergen or exercise but that chronic administration results in significant attenuation of these responses. As the GR is found throughout all cells and tissues, a potential for significant adverse effects listed in Table 40.3 exists (35). Therefore, corticosteroids with high topical activity with pharmacokinetic properties that limit systemic activity that can be administered by inhalation were developed.
Table 40.3 Adverse Effects of Glucocorticoid Administration (35) | ||||||||||||||||||
---|---|---|---|---|---|---|---|---|---|---|---|---|---|---|---|---|---|---|
|
The ICSs are considered the preferred long-term controller medications for all levels of persistent asthma due to their consistent efficacy resulting in reduction in bronchial hyperresponsiveness and asthma exacerbations and improvement in lung function. The current ICSs approved for use in the United States and their recommended doses for children are listed in Table 40.4 that is modified from the EPR3 (9,14). Chemical modifications of the corticosteroids produce different binding affinities (potency) for the GR (Table 40.5) that can simply be overcome by giving equipotent doses (14,35). Thus, when assessing “clinical comparable doses,” lung delivery from various delivery devices as well as potency need to be taken into account. Table 40.4 also presents the clinically comparable doses for children established by comparative clinical trials (9,15). There are relatively little data in children aged 4 years or younger where delivery issues become even more variable. Although it has been postulated that the newer HFA-MDI ICSs formulated to deliver ultrafine particle may have greater efficacy due to penetration into the small airways, clinical trials do not support a difference other than that produced by total lung delivery (15).
|
Pharmacokinetics
Differences in the molecular structures of ICSs result in altered pharmacokinetic properties of these agents (Table 40.5), which are the primary determinants of the topical to systemic activity (therapeutic index) (15). Figure 40.2 provides a schematic of where each of the pharmacokinetic variables can affect the therapeutic index (9,14,35). The inhaled route of administration has the advantage of delivering drug to the site of action, but only 5% to 60% of the inhaled dose is delivered to the lung; about 50% to 80% of the drug deposits in the oropharynx and swallowed while the rest is exhaled or left in the device (14,15). Topical selectivity of the ICSs can be improved by decreasing oral bioavailability, increasing systemic clearance, and prolonging residence time in the lung (15,35,36). Systemic
bioavailability of ICSs depends on the oral bioavailability and the amount of drug which enters the systemic circulation via lungs. Oral bioavailability can be altered by oral absorption, increased first-pass intestinal wall and hepatic metabolism mediated by the cytochrome P450 3A4 (CYP3A4) isozymes, and decreasing oropharyngeal deposition by using a VHC with MDI for compounds with significant oral absorption such as beclomethasone dipropionate (15,35,36). However, for compounds with very high first-pass metabolism such as fluticasone propionate, using a VHC with an MDI may actually increase systemic exposure by increasing lung delivery (19,35). This can adversely affect the therapeutic index (35,37). Although children aged 2 years or younger can theoretically receive higher milligram per kilogram doses of ICSs than do older children as discussed above, there is insufficient data to determine whether they are at greater risk for adverse systemic effects.
bioavailability of ICSs depends on the oral bioavailability and the amount of drug which enters the systemic circulation via lungs. Oral bioavailability can be altered by oral absorption, increased first-pass intestinal wall and hepatic metabolism mediated by the cytochrome P450 3A4 (CYP3A4) isozymes, and decreasing oropharyngeal deposition by using a VHC with MDI for compounds with significant oral absorption such as beclomethasone dipropionate (15,35,36). However, for compounds with very high first-pass metabolism such as fluticasone propionate, using a VHC with an MDI may actually increase systemic exposure by increasing lung delivery (19,35). This can adversely affect the therapeutic index (35,37). Although children aged 2 years or younger can theoretically receive higher milligram per kilogram doses of ICSs than do older children as discussed above, there is insufficient data to determine whether they are at greater risk for adverse systemic effects.
|
Systemic clearance is the primary determinant other than dose for steady-state serum concentrations with chronic dosing. Most of the ICSs undergo unrestrictive hepatic extraction and metabolism by CYP3A4 so that their clearances approach liver blood flow (L90 L per hour) (15,36). The exceptions are the active metabolites of beclomethasone dipropionate (beclomethasone 17-monopropionate) and ciclesonide (desisobutyryl-ciclesonide) that apparently undergo extrahepatic metabolism possibly via blood esterases (15). Retention in the lung allows ongoing exposure to the GRs and exposes the systemic circulation to lower concentrations. It occurs with more lipophilic compounds such as fluticasone propionate and is evidenced by a longer elimination half-life for inhaled than intravenous administration (15). Some of the ICSs undergo intracellular fatty acid esterification at the free hydroxyl group at the carbon 21 position (i.e., budesonide, triamcinolone acetonide
and desisobutyryl-ciclesonide), which maintains a depot of drug in the cell (15,36). However, this property has not been established to confer an improved therapeutic index or prolonged duration of effect over agents that do not undergo esterification.
and desisobutyryl-ciclesonide), which maintains a depot of drug in the cell (15,36). However, this property has not been established to confer an improved therapeutic index or prolonged duration of effect over agents that do not undergo esterification.
Clinical Use
Figure 40.1 presents the current EPR3 recommendations that, regardless of age, ICSs are the preferred agents for persistent asthma and the dose should be based on the severity and control of the disease. The ICSs are the only agents demonstrated to reduce the risk of dying from asthma (9). Furthermore, low-medium doses of ICSs have consistently been shown to improve lung function, decrease bronchial hyperresponsiveness and asthma exacerbations, and reduce the need for as needed rescue with short-acting β2-agonists and oral corticosteroids (9,12,38,39). In addition, ICSs reduce sputum eosinophils and fraction of exhaled nitric oxide (FeNO), a downstream end product of eosinophilic inflammation. Comparative clinical trials in children aged 5 to 11 years show that they are more effective than all alternative long-term controllers (9,38,40,41,42,43,44,45). In addition, studies in children younger than 5 years have shown fluticasone propionate by MDI + VHC and budesonide nebulization suspension to be more efficacious than cromolyn and budesonide nebulization to be more effective than montelukast (46,47,48). Chronic administration of ICSs reduces the degree of bronchial hyperresponsiveness in children, including exercise-induced bronchospasm (EIB) (38,49). They reduce EIB more effectively and consistently than does montelukast (50). Despite their activity in preventing exacerbations and reducing symptoms and airway responsiveness, ICS therapy does not prevent loss of lung function, does not prevent development of asthma in high-risk infants, and does not enhance the lung growth in children with asthma (12,13,38,51).
The clinical response to ICSs varies among patients, but most asthma symptoms will improve in 1 to 2 weeks, with maximum improvement in 1 to 2 months. Maximum improvement in lung function requires 3 to 6 weeks of treatment and at least 1 to 3 months to improve bronchial hyperresponsiveness, although it may continue to improve over many months (31,38). The response for FeNO occurs within a few days so that it has been touted as a means of monitoring ICS therapy for adherence and adjusting doses (52). In clinical trials, FeNO has been used as an inflammatory biomarker to characterize patients and to compare relative potency of ICSs as well as compare ICSs to other therapies (43,52,53,54). A chemoluminescence analyzer has been approved by the FDA for use in monitoring ICS therapy and handheld devices are being developed (52). However, clinical trials in children assessing the utility of monitoring FeNO in addition to symptoms and lung function have obtained mixed results; so, although it has been shown to be able to predict future exacerbations, its routine use is uncertain and requires further study (55,56).
For those patients who have not achieved adequate control on low doses of ICSs, the EPR3 recommends an increase in the dose of ICSs or add an adjunct therapy such as a leukotriene-receptor antagonist (LTRA) or an inhaled long-acting β2-agonist (LABA) (9). The preferred recommendations vary with age primarily because no adjunctive therapy to ICSs has been evaluated in children younger than 5 years, and minimal studies have compared adjunctive therapy to increased ICS in children aged 5 to 11 years and only recently have adjunctive therapies been directly compared (56b). In addition, dose–response studies for ICSs in children are few. The ICSs are considered to have a relatively flat dose–response curve. In children 4 to 14 years old, the response to fluticasone propionate tends to plateau between 100 and 200 μg daily in most patients with some further benefit in more severe patients obtained at 400 μg daily (57). A dose–response study of budesonide between 200, 400, and 800 μg daily, which is one-half as potent as fluticasone propionate, confirms these findings (58). In adults with relatively moderate-to-severe asthma, doubling the dose of ICS does not decrease asthma exacerbations but quadrupling the dose does despite minimal effect on lung function (59,60). Similar studies have not been carried out in children, but in general, a doubling of the dose of ICS does not significantly increase benefit (15,57,58). In young children and infants, the data are even less clear. One study comparing fluticasone propionate 100 and 200 μg daily by MDI + VHC and face mask in 237 infants 12 to 47 months old reported a dose response for decreased exacerbations; however, the decrease in exacerbations for the 100 μg daily was not significantly different from placebo (39). In addition, the pivotal trials for budesonide nebulizer suspension failed to demonstrate a dose–response for 500 to 1,000 μg daily after the dose exceeded 250 μg once daily which was not consistently more effective than placebo across studies (61).
The issue of once-daily administration of the ICSs to improve compliance is often raised. Although only budesonide and mometasone furoate currently have once-daily FDA approved labeling, most mild persistent patients controlled on low to medium dose ICS can be adequately controlled with once-daily administration with all the ICSs. None of the ICSs have specific pharmacokinetic properties that allow for once-daily dosing and they all work more effectively in moderate-to-severe asthma with twice-daily dosing (15).
Oral corticosteroids are indicated for asthma exacerbations not responding to inhaled short-acting β2-agonists (9). They are usually administered as a short course or burst (3 to 10 days) at the equivalent dose of 1 to 2 mg per kg daily prednisolone equivalent once or twice daily. Multiple daily dosing may provide superior activity and decreased gastrointestinal effects. The number of oral corticosteroid bursts is used to determine both risk and the level of control (Table 40.2) (9). Oral corticosteroids administered in the emergency department and continued for 3 to 7 days reduce the risk of relapse (9).
Adverse Effects
The adverse effects of ICSs are dose related (35). The degree of systemic exposure depends on the inhaler design, patient’s technique, physical property of the particles, and the pharmacokinetics. All of the ICSs have relatively few adverse effects at the low-medium doses and when they are used properly. Although there are potential differences based upon pharmacokinetics, patients are at
risk of experiencing systemic effects at high doses of the ICSs and should be monitored appropriately.
risk of experiencing systemic effects at high doses of the ICSs and should be monitored appropriately.
Deposition of the ICSs in the oropharynx may lead to dysphonia and hoarseness, oral candidiasis, and cough at the time of inhalation. Dysphonia and hoarseness are dose-related side effects with an incidence of 5% to 50% and may be due to the myopathy of the laryngeal muscles (31,62). Oral candidiasis is also dose related with an incidence of less than 5%, and may be prevented by rinsing the mouth with water after the dose (62). In infants using either nebulized ICS or MDI + VHC with a face mask, the nose and mouth should be washed off after each treatment. Cough is mainly due to local irritation and may be resolved by changing the delivery device, the rate of inhalation, or using a VHC.
The systemic side effects of the ICSs include suppression of the hypothalamus–pituitary–adrenal (HPA) axis, adrenal insufficiency, Cushing syndrome, osteoporosis, skin thinning and ecchymoses, cataracts, and growth retardation in children. Suppression of HPA axis is a rare event and is usually clinically insignificant (35,63). Nonetheless, adrenal insufficiency has been reported in children when high doses have been used (64,65). A recent large survey in France found 46 cases of adrenal insufficiency, 24 of which were associated with ICS use, 14 of which were children 0.3 to 14 years old (median 9.5 years old) (65). Seven were with fluticasone propionate, five with budesonide, and two with beclomethasone dipropionate. All patients received a daily dose of 500 μg or more beclomethasone equivalent (≥250 μg daily fluticasone propionate). Of the total 24 cases, 12 were associated with potential drug interactions (6 ritonavir and 6 itraconazole) with fluticasone propionate and budesonide (see discussion later). There are several methods for assessing the integrity of the HPA axis, ranging from testing for basal production of the adrenal glands to reserve capacity. Tests of basal production include spot morning serum cortisol, serial overnight (12-hour) or 24-hour area under the curve serum cortisol (AUC0–24), and 12-hour (overnight) and 24-hour urine free cortisol (UFC) (corrected for creatinine to normalize data) concentration testing (35,66,67). Serum cortisol AUC0–24 and 24-hour UFC are the most sensitive, and UFC has the advantage of not requiring blood draws in children; however, they do not assess the ability of the HPA axis to respond to stress (35,66). The gold standards for assessing full integrity of the HPA axis are the insulin tolerance test and metyrapone tests, which are relatively invasive and difficult to perform; therefore, adrenocorticotropic hormone (ACTH) cortisol stimulation test is performed as the standard. Low-dose slow infusion of ACTH is being touted as more sensitive, as it gives a dose of ACTH similar to that seen during stress as opposed to the supraphysiologic doses used with the standard ACTH stimulation that may give false negative results (66). However, the results of low-dose ACTH tests have not been completely validated and may produce false-positive results in patients without clinically relevant suppression (35,66). There does not appear to be a chronic cumulative effect of medium dose ICSs on the HPA axis as measured over 3 years in children receiving budesonide 400 μg daily (68). Four or less oral courses of prednisone per year is not associated with adrenal suppression, and significant adrenal suppression does not occur following individual short courses less than 10 days (9).
High-dose ICSs can reduce bone mineral density and increase the risk of fracture in older patients at risk; however, the data in children are less clear (35). Bone mineral density does not seem to be altered with low to medium doses of the ICSs (38); however, a recent prospective study over a median of 7 years in children 5 to 12 years old reported a decrease in bone mineral accretion with medium dose ICS in boys but not girls that did not result in an increased risk of osteopenia or fractures (69). An epidemiologic study of 97,387 children 4 to 17 years old receiving ICSs in the United Kingdom reported an increased crude risk of fracture in those receiving greater than 200 μg per day beclomethasone dipropionate equivalent, but when adjusted for measures of asthma severity, the increased risk disappeared (70). A nested case-control analysis using the same UK database also failed to demonstrate a significant increase in fracture risk from current ICS use or current long-term exposure (≥20 prescriptions) (71). However, short courses of oral prednisone have been associated with a dose-dependent decrease in bone mineral accretion and an increased risk of osteopenia (at least two bursts per year) and fractures (four or more bursts per year) (69,72).
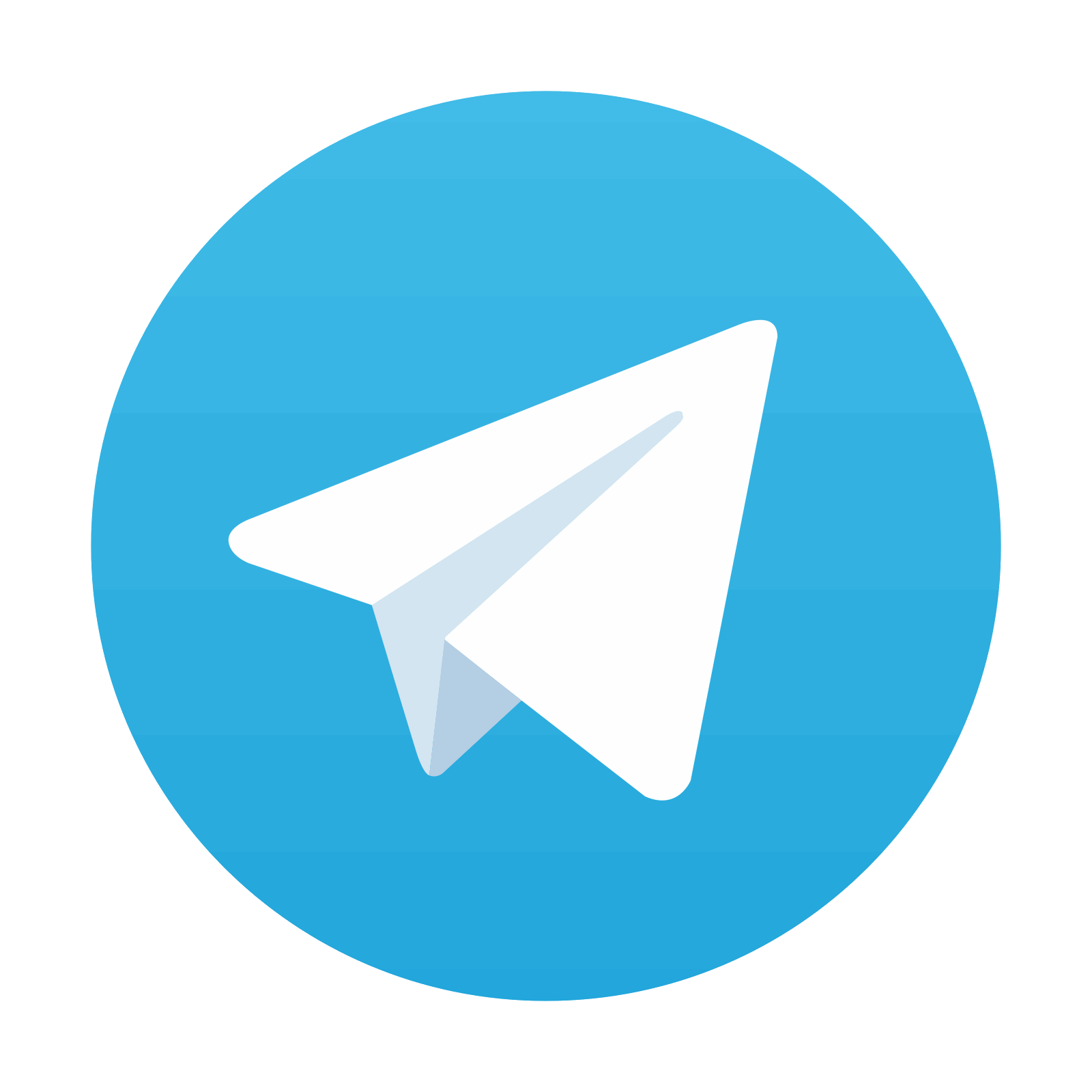
Stay updated, free articles. Join our Telegram channel

Full access? Get Clinical Tree
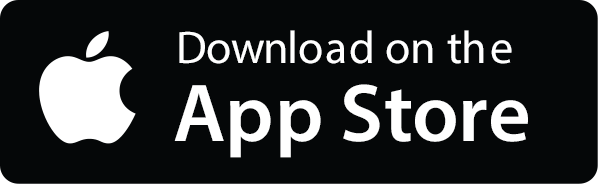
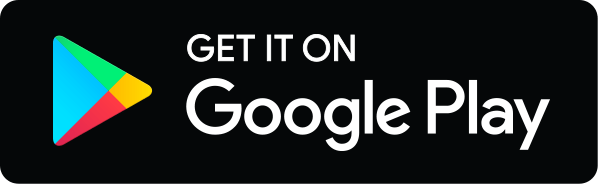