A thorough understanding of the nature of the parturient’s pain is the first aspect in providing optimal obstetric anesthetic care. Once the biology and pathophysiology of this special acute pain is discussed, the benefits of analgesia for this pain will appropriately follow. Pharmacology of local anesthetics and related drugs will be reviewed, with special emphasis on complications associated with their administration. A variety of techniques including epidural, subarachnoid, and other regional and analgesia techniques will be discussed with benefits and complications reviewed. General anesthesia (GA) for cesarean section delivery will be outlined. A variety of special consideration patients will be addressed, including: (1) the preeclamptic patient, (2) preterm birth patient on tocolytics, (3) human immunodeficiency virus (HIV)–positive mothers, (4) coagulopathies, (5) cardiac disease, and (6) pulmonary disease. Highlights from the updated report by the American Society of Anesthesiologists task force on Obstetric Anesthesia and Society for Obstetric Anesthesia Perinatology will be reviewed.
The current concept of pain focuses on the peripheral nervous system relaying a stimulus to the central nervous system (CNS) for interpretive evaluation—the somatosensory system (Fig. 20-1). The peripheral system consists of afferent neurons that are embedded in body tissues awaiting nociceptive (painful) stimuli. These afferent neurons are termed Ad (A-delta) and C-fibers. These fibers transverse into the spinal segments and synapse at the dorsal spinal ganglion. Here, substance-P is released causing the painful effect to be initiated. From each spinal segment stimulated, these messages ascend through one of two pathways to the thalamus for further modulation: the lateral spinothalamic tract or the medial lemniscus tract. Once at the thalamus, adjustment and regulation from inherent emotional and psychological factors occur. The data support the emphasis on the importance of perceptual factors that influence a patient’s total pain experience (Table 20-1). The psychodynamics of prior experience, motivation, anxiety, anticipation of pain, attention, personality, and ethnic and cultural factors all influence the modulation of substance-P release, affecting the pain experience. From the thalamus, this information is synthesized in the sensory cortex for relay to the many effector sites that contribute to the pain response. Once pain has been perceived, there is an initiation of the pain response that has neuroendocrine, behavioral, and psychological implications.
Psychological | Anxiety, fear, emotional arousal |
Behavioral | Verbalization, motor activity |
Neuroendocrine | Hyperventilation—maternal respiratory |
Endocrine (stress) response | |
↑ adrenocorticotropic hormone (ACTH), ↑ cortisol | |
↑ epinephrine, norepinephrine | |
↑ lipolytic metabolism—metabolic acidosis | |
Cardiovascular Response | |
↑ systemic vascular resistance | |
↑ cardiac output | |
↑ blood pressure | |
↑ oxygen consumption | |
↑ left ventricular stroke work | |
Gastrointestinal function | |
↓ gastric motility | |
↑ risk for gastroesophageal reflux/aspiration | |
↑ nausea and vomiting | |
Urinary function | |
↓ emptying—urinary retention oliguria | |
Fetal effects | ↓ uterine blood flow |
↑ fetal heart rate alterations |
In humans, there is a 300% to 600% increase in epinephrine and 200% to 400% increase in norepinephrine levels produced during severe pain experienced in active labor. There is a 200% to 300% increase in cortisol levels, as well as, increases in corticosteroid and adrenocorticotropic hormone levels reaching their peaks at or after delivery. During labor, the cardiac output (CO) increase is 40% to 50% with a further 20% to 30% increase during painful contractions. The systolic and diastolic blood pressures also increase by 20 to 30 mm Hg. These increases in CO and systolic blood pressure (SBP) lead to a significant increase in left ventricular stroke work that may be harmful to patients with preeclampsia, hypertension, cardiac valvular disease, pulmonary hypertension, or severe anemia.
With the sympathetic-induced lipolytic metabolism, increases in free fatty acids and lactate produce relative maternal acidosis. Increased sympathetic activity increases metabolism and oxygen consumption, and decreases gastrointestinal and urinary bladder motility. Respiratory rate is increased with resultant respiratory alkalosis. The kidney compensates by excreting HCO3–. Diminished PaCO2 levels below 25 to 27 mm Hg may result in increased uteroplacental resistance and reduced oxygen delivery to the fetus.
During peak contractions, there is a reduction in intervillous blood flow leading to a significant decrease in placental gas exchange. This exaggerates the already dramatic reduction in uterine blood flow, secondary to the increases in norepinephrine and cortisol (see Table 20-1).
By blocking nociceptive input, there is a reduction in the release of catecholamines, adrenal corticotropic hormone, and cortisol in the parturient (Table 20-2). Effective analgesia significantly reduces pain-related hemodynamic changes. Maternal CO fluctuations are modulated and oxygen consumption is reduced. Gastric and bladder motility is not adversely affected by neuroblockade. Cautious epidural analgesia has been reported to provide a vasomotor blocking effect that increases intervillous blood flow and oxygen delivery to the fetus.
Psychological | ↓ Anxiety, ↓ fear, ↑ emotional stability |
Behavioral | ↓ Motor activity |
Neuroendocrine | ↓ Respiratory alkalosis (maternal) |
↓ Catechol release | |
↓ Cortisol | |
↓ Adrenocorticotropic hormone (ACTH) | |
↓ Metabolic acidosis (maternal) | |
↓ Cardiac output | |
↓ Oxygen consumption | |
↓ Left ventricular stroke work | |
Normal gastrointestinal function | |
Normal urinary function | |
Fetal effects | ↑ Uterine blood flow |
More stability in fetal heart rate tracing |
The first stage of labor refers to the beginning of cervical dilation and effacement until its completion. The second stage of labor extends from complete cervical dilation until delivery of the infant. The third stage is the delivery of the placenta (see Table 20-2).
Transmission of pain during the first stage of labor is from spinal segments (pain fibers) T10, T11, T12, and L1, and the second and third stage of labor is from S2, S3, and S4 (Fig. 20-2). It is important for the care provider to understand the differences between the pain conduction (spinal levels) during the first stage of labor, as compared with the second and third stage, in order to provide appropriately directed analgesia. It is also important to consider that pain may also be due to the pathology of the gestation, such as abruptio placenta, infection, adnexal torsion, or appendicitis, and these may be masked if total neuroblockade is achieved from T4 to S5 at the onset of labor. Only segments necessary for the specific pain of labor should be blocked (Table 20-3). Relatively decreased fetal perfusion has been reported to occur both in the presence and absence of overt maternal hypotension secondary to a sympathectomy-mediated reduction in uterine blood flow.
Uterus | Motor fibers from parasympathetic pelvic nerves from S2, S3, S4, and sympathetic sensory |
Tubes/ovaries | Motor fibers from parasympathetic pelvic nerves from S2, S3, S4, and sympathetic sensory fibers via the ovarian plexus from T12, L1 |
Broad ligament | Motor fibers from parasympathetic pelvic nerves from S2, S3, S4, and sympathetic sensory fibers via the hypogastric plexus from T12, L1 |
Cervix | Motor fibers from parasympathetic pelvic nerves from S2, S3, S4, and sympathetic sensory fibers via the hypogastric plexus from T12, L1 |
Vagina | Motor fibers from parasympathetic pelvic nerves from S2, S3, S4, and sympathetic sensory fibers via the hypogastric plexus from T12, L1 |
Vestibule/hymen | Erectile vasodilator fibers from parasympathetic pelvic nerves from S2, S3, S4 |
Labia | Posterior labial nerve S2, S3, and perineal branch of the posterior femoral cutaneous nerve S1, S2, S3 |
Clitoris | Erectile vasodilator fibers from parasympathetic pelvic nerves from S2, S3, S4 |
Perineum | Motor and sensory innervation from the pudendal nerve arising from S2, S3, S4 |
Bladder | Sympathetic fibers from T11, T12, L1, L2 via the superior/inferior hypogastric plexuses control the sphincter and parasympathetic fibers from S2, S3, S4 control filling/emptying of the bladder |
Anus | Motor and sensory innervation from the pudendal nerve arising from S2, S3, S4 |
The desired action of all local anesthetics is the reversible blockade of nerve conduction and, therefore, the cascade of events producing perception of pain. These drugs prevent the development of an action potential in a nerve by blocking sodium channels responsible for propagating a response in the nerve fiber (Fig. 20-3). Local anesthetics exist in both charged and uncharged form. The uncharged state of the drug crosses the lipid nerve membrane and enters the cell. Once in the cell, it re-equilibrates into the charged form that is readily dissolved in water. This charged form now reaches the sodium channels and blocks them from inside (see Table 20-3).
FIGURE 20-3
Uncharged (1) local anesthetic passes through the nerve membrane (2) into the cell (3) where the local anesthetic gains a H+ (hydrogen ion) to become charged. (4) The Na+ channel is blocked in the nerve cell by the charged local anesthetic and does not allow the nerve to propagate a nerve impulse.

The capacity for an uncharged species to assume a charged form is the essential property of all local anesthetics. They are a combination of a weak base and a strong acid.
As a general principle, lowering the pH will increase the ionized percentage of the drug, and raising the pH will increase the uncharged form of the drug. Because the local anesthetics are usually supplied in an acidic medium, the addition of NaHCO3 will increase the relative uncharged portion, allowing the drug to cross the nerve membrane more readily resulting in a quicker onset of block.
0.1 cc NaHCO3/10 cc bupivacaine
1.0 cc NaHCO3/10 cc lidocaine
Precipitation of the local anesthetic will occur if too much NaHCO3 is added. Comparative properties of lidocaine, ropivacaine, and bupivacaine are outlined in Table 20-4.
Property | Lidocaine | Bupivacaine | Ropivacaine |
---|---|---|---|
Molecular weight | 234 | 288 | 274 |
pKa | 7.7 (at pH 7.4, more drug in basic form) | 8.1 | 8.0 |
Lipid solubility (directly related to speed of onset) | ↑↑↑↑↑ | ↑↑ | ↑↑↑ |
Protein binding | 64% | 95% | 94% |
Elimination T1/2 (h) | 1.6 | 2.8 | 1.9 |
Maximum dosea | 5 mg/kg (350 mg in a 70-kg patient) (7 mg/kg with epinephrine) | 2-3 mg/kg (175 mg in a 70-kg patient) | >2 mg/kg |
Toxicity | Seizures | Cardiac arrest | Seizures with much larger doses |
Protein binding is another property important to understand. All local anesthetics bind to albumin and μ-1-acid glycoprotein (AAG). It is the free portion of the drug that is responsible for toxicity. In pregnancy, albumin levels are depressed, so AAG binding becomes most important. AAG is released in response to surgery, trauma, infection, and inflammation. Once AAG sites are saturated with local anesthetics, the free drug levels progressively increase. Protein binding also decreases with decreasing pH. Therefore, in an acidotic environment, a high proportion of free drug with the potential for cardiac or neurotoxicity should be anticipated.
Absorption of local anesthetics refers to the movement of the drug from the site of injection to the bloodstream. Therefore, the more vascular the area and the larger the total dose of anesthetic used, the higher the resulting serum level of the drug. The addition of a vasoconstrictor (epinephrine) to the local anesthetic can decrease the absorption and, therefore, toxicity of the drug used (see Table 20-4).
Local anesthetic toxicity may manifest with CNS or cardiac effects. As the doses increase, disinhibition and CNS excitation occurs producing seizures. Local anesthetic bind and inhibit cardiac Na+, Ca2+, and K+ channels as concentrations increase causing cardiac arrest. Treatment of adverse reactions depends on the severity of their effects from spontaneous recovery, to supportive care with oxygen and maintaining the airway, to advanced cardiac life support (ACLS).
Ropivacaine is a local anesthetic with similar characteristics to bupivacaine. This is produced solely as the S-enantiomer, whereas bupivacaine is a mixture of the S and R forms. This S-form has less ability to bind Na-channels in the myocardial conduction system. Therefore, there is less risk of cardiotoxicity with ropivacaine. As seen in Table 20-4, ropivacaine and bupivacaine have very similar physical properties except for the margin of safety, in ropivacaine, which produces less toxic side effects. There also appears to be less of a motor block compared to bupivacaine, which theoretically should improve patient satisfaction during the laboring process. Caution has been used in administering this agent as it has several side effects that occur in a significantly high occurrence (>10% of patients). These include cardiovascular: hypotension (dose-related and age-related: 32%-69%), maternal bradycardia (6%-20%), gastrointestinal: nausea (11%-29%), vomiting (7%-14%), and neuromuscular and skeletal: back pain (7%-10%) of patients.
The pharmacologic treatment of local anesthetic systemic toxicity (LAST) is different from other cardiac arrest scenarios.
Airway management—ventilate with 100% oxygen
Seizure suppression with benzodiazepines avoiding propofol
Use ACLS avoiding vasopressin, calcium channel blockers, β-blockers, or local anesthetics reducing epinephrine dose to less than 1 μg/kg
20% Intralipid 1.5 cc/kg bolus over 1 minute; start continuous infusion 0.25 cc/kg/min
Bolus may be repeated for persistent cardiac arrest and infusion rate may be doubled to 0.5 cc/kg/min; approximate upper limit is 10 cc/kg of the lipid emulsion.
Lidocaine → 5 mg/kg
1% lidocaine = 10 mg lidocaine/cc
Maximum dose 35 cc
2% lidocaine = 20 mg lidocaine/cc
Maximum dose 17.5 cc
Lidocaine with epinephrine → 7 mg/kg
1% lidocaine with = 10 mg lidocaine/cc
1: 200,000 epi plus
5 μg epinephrine/cc
Maximum dose 49 cc
2% lidocaine with = 20 mg lidocaine/cc
1: 100,000 epi plus
10 μg epi/cc
Maximum dose 24.5 cc
Marcaine with or without epinephrine → 2.5 mg/kg
0.25% Marcaine = 2.5 mg/cc
Maximum dose 70 cc
0.5% Marcaine = 5 mg/cc
Maximum dose 35 cc
Lumbar epidural block is the most common form of analgesia used to provide relief from the nociceptive pathways during the stages of labor. The epidural space is the interval superiorly bounded by the foramen magnum, inferiorly by the lower end of the dural sac, anteriorly by the posterior longitudinal ligament, and posteriorly by the ligamentum flavum. The approach to the epidural space is posteriorly through the skin, subcutaneous fat, supraspinous ligament, interspinous ligament, ligamentum flavum, and into the epidural space (Fig. 20-4).
The size of the epidural space varies along its course with the largest diameter existing at the L2 interspace with a range of 4 to 9 mm. A simple maneuver that helps open up the bony entrance to the epidural space is flexion of the lumbar spine (Fig. 20-5).
The contents of the epidural space include fat, vertebral venous plexus, lymphatics, arteries, and dural spinal nerve projections. In pregnancy, with the increase in intra-abdominal pressure, the venous plexus becomes distended. This phenomenon and the accompanying increase in epidural fat that occurs during pregnancy, functions to reduce epidural volume substantially. Therefore, pregnant patients usually require less volume of local anesthetic, as compared to nonpregnant controls, to produce a similar level of blockade.
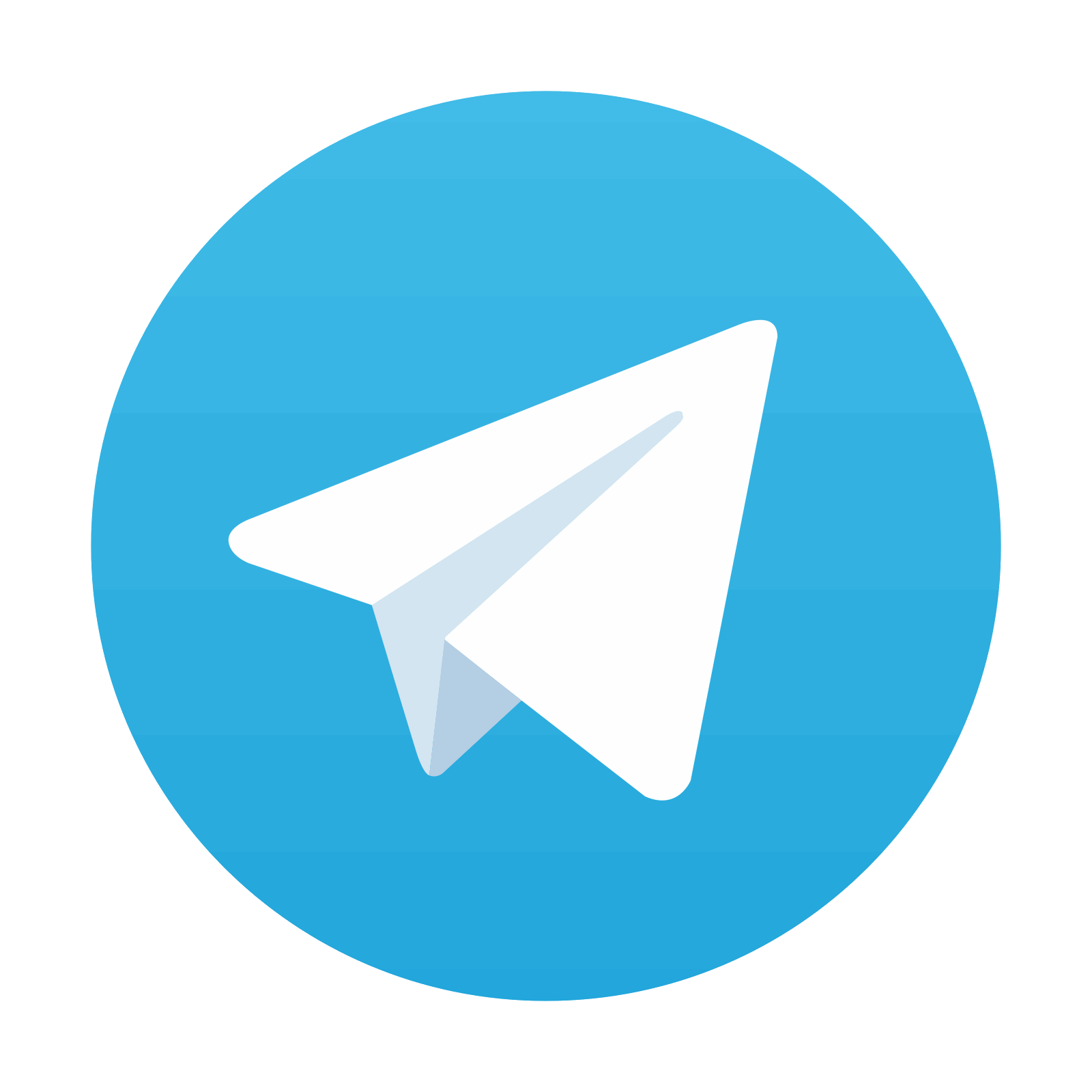
Stay updated, free articles. Join our Telegram channel

Full access? Get Clinical Tree
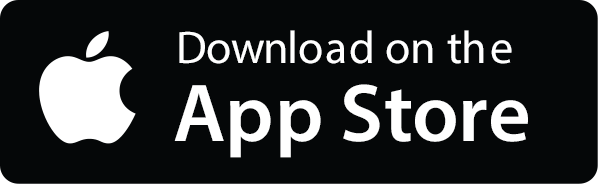
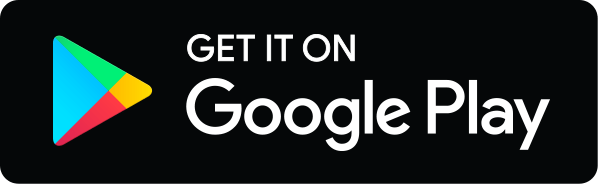