Sleep and epilepsy are interrelated; worsening of one leads to worsening of the other and a vicious cycle starts. Sleep activates interictal epileptiform discharges (IEDs) and seizures. Epilepsy, on the other hand, worsens sleep architecture and quality. The interface of sleep and epilepsy is well known since the ancient times of Aristotle. He suggested that “sleep is similar to epilepsy and in some way sleep is to epilepsy”. In 1885, Gower noted that in a fifth of patients, grand mal seizures occurred exclusively at night (1). Additionally, he noted two peaks of seizure occurrence in sleep—2 hours after sleep onset and between 3 a.m. and 5 a.m. Fere described that nocturnal seizures reduced sleep efficiency (2–4). The activating nature of sleep on epileptiform discharges was first described by Gibbs and Gibbs in 1947 (2–4). In this chapter, we discuss these interactions and provide guidelines on how to approach a child with seizures and sleep problems.
MECHANISMS OF INTERACTION FOR SLEEP AND EPILEPSY
Sleep is well known to activate IEDs and seizures. There are several mechanisms hypothesized to explain the interrelationship between sleep and epilepsy.
Neuronal Hypersynchrony
The first theory focuses on neuronal network hypersynchrony during nonrapid eye movement (NREM) sleep. Distinct sleep promoting (ventrolateral preoptic region of hypothalamus—VLPO) and wake promoting (ascending arousal) systems exist in the brainstem and hypothalamus. The wake promoting system inhibits the sleep promoting systems during wakefulness and the sleep promoting system inhibits wake promoting regions during sleep; thus, sleep–wake cycles are maintained. During the wake state, the arousal systems exert tonic cholinergic inhibition on the thalamocortical system. The thalamocortical system comprises a complex interrelationship between cortical pyramidal cells, relay nuclei, and the reticular nucleus of thalamus. At sleep onset, VLPO inhibits this tonic inhibition, leading to liberation of thalamocortical neurons. This allows for development of slow oscillations below 1 Hz in the cortex, which has depolarizing and hyperpolarizing phases. The depolarizing phase is characterized by extensive synaptic activity and hypersynchrony of neurons, as well as formation of delta activity and sleep spindle. The slow oscillations build up in small regions but gradually become widespread, leading to synchronized delta activity seen in N3 (slow-wave sleep). The slow oscillations have been shown to be associated with the formation of spike–wave discharges. Due to hypersynchrony, spikes spread in wider areas. It is believed that the spikes–waves are also generated by the same thalamocortical system; hence, they are more common during NREM sleep. The reactive events in sleep, which occur in response to internal or external stimuli, lead to microarousals resulting in sleep stage shifts. During REM sleep, a part of the arousal system is activated, which results in desynchrony in the networks. Hence, the spike activity is reduced (5).
Cyclic Alternating Pattern
Cyclic alternating pattern (CAP) represents an endogenous rhythm in NREM sleep, which is characterized by sequences of transient electrocortical activations that are distinct from background activity. These sequences are repeated several times during sleep and are a marker of unstable sleep. A scoring system for CAP is based on electroencephalogram (EEG) activity during sleep. Phase A of CAP represents the transient activation and is subdivided into (a) phase A1 of synchrony, comprising delta bursts, K-complex sequences, and vertex waves; (b) phase A2 of a mixture of slow and fast rhythms; and (c) phase A3 of rapid low-voltage rhythms. A1 subtypes dominate the first part of the NREM sleep and are involved in generation and maintenance of EEG synchronization. On the other hand, A2 and A3 subtypes dominate the final part of the NREM sleep cycle and disrupt EEG synchronization, leading to desynchronization for REM sleep onset. Phase B represents the background activities in between two phase A activities. The sequences are interrupted by the presence of a stable sleep without oscillations, called non-CAP (NCAP) (6,7). Based on the sequences, a CAP rate is calculated by the percentage ratio of total CAP time to NREM sleep time, which is a measure of arousal instability (6).
The thalamocortical system is suggested to be the generator of the CAP sequences, similar to the generation of IED in sleep. Moreover, most IEDs occur during phase A of CAP, as discussed in the following text in detail. Hence, it is believed that a CAP sequence triggers paroxysmal events such as IEDs, while IEDs promote the generation of phase A and, hence, increase instability. On the other hand, the non-CAP part of sleep is unfavorable to IED generation due to reduced arousals (8).
Adenosine
Adenosine is the third mechanism believed to influence the complex relationships of sleep and epilepsy. Extracellular adenosine exerts antiepileptic effects through pre- and postsynaptic adenosine receptors. Adenosine kinase (ADK) activity regulates the adenosine levels; increased activity reduces adenosine level and increases neuronal excitability. This endogenous adenosine mechanism is disrupted in chronic epilepsy. In studies, ADK activity was found to be overexpressed in mesial temporal sclerosis and temporal lobe epilepsy. Additionally, there was decreased expression of the level of A1 receptors (9). Moreover, adenosine is essential in the regulation of sleep homeostasis; poor sleep occurs in the presence of dysfunction (10). So, a dysregulated adenosine system may cause poor sleep in patients with chronic epilepsy.
EFFECTS OF SLEEP ON EEG, SEIZURES, AND EPILEPSY
Sleep and Interictal Epileptiform Discharges
Sleep activates IEDs in focal and generalized epilepsy. Almost two-thirds of the patients with grand mal seizures have IEDs in sleep. Studies in adults with focal epilepsy show that the spike frequency increases with increasing depth of NREM sleep, while in REM sleep spikes are reduced (11–13). Children also have activation of IEDs in sleep. In children, IEDs in focal epilepsies are more common during N1 and N2 (14). Moreover, children with their first unprovoked seizure had IEDs only during sleep (15). Hence, obtaining sleep EEG is essential in the diagnosis of seizures/epilepsy.
Sleep also affects morphology and the spread of IEDs. In studies, the field of IEDs increases with increasing depth of NREM sleep (11,12). In about 18% of subjects with unilateral IEDS during wakefulness, bilateral IEDs were seen in sleep (12). On the other end, spike field is reduced in REM sleep (13). Furthermore, EEG of certain epilepsy syndromes such as benign epilepsy with centrotemporal spikes (BECTS) and electrical status epilepticus during sleep (ESES) had marked improvement in REM sleep (16). Hence, REM-related IEDs are helpful in localizing seizures even for epilepsy surgery evaluation (12,17,18). Moreover, sleep also alters the morphology of IEDs. Typical 3 Hz spike-and-wave discharges of absence epilepsy present as single spike-wave or polyspike-and-wave discharges during NREM sleep. There may be frontal and/or temporal bisynchrony during sleep (1). Specific syndromes associated with particular sleep EEG patterns are discussed later in the Sleep-Related Epilepsy Syndromes section.
CAP, IEDs, and Seizures
CAP is altered in patients with epilepsy, as discussed earlier. CAP rate, which is suggestive of sleep instability, is increased in genetic generalized epilepsy and in children with drug-resistant epilepsy (19). In genetic generalized epilepsy, and in lesional temporal lobe epilepsy, higher IEDs are seen during phase A1 as compared to non-CAP. Additionally, in both focal and generalized epilepsy, about 90% of spikes are seen in CAP phases (8). Moreover, in children with BECTS, CAP analysis showed reduced instability and suggested that the centrotemporal spikes may disrupt the physiologic synchronization mechanism (20). Furthermore, seizures also occurred during the CAP cycle and in phase A more than in phase B (21).
Sleep and Seizures
Due to changes in sleep rhythmicity, seizures tend to occur during specific sleep stages. In various studies, about 20% of seizures occurred in sleep. In multiple studies, seizures in partial epilepsy were more likely to occur during N2 and were rare in REM sleep (22,23). A recent study reviewed 42 studies evaluating the issue of seizure occurrence in different sleep stages. As compared to wakefulness, in REM sleep 7.83 times fewer focal seizures, 3.25 times fewer generalized seizures, and 1.11 times fewer focal interictal discharges occurred (16). Additionally, seizures in N1, N2, and N3 were 87, 68, and 51 times more common than in REM sleep, respectively (16). Moreover, seizure occurrence in sleep may also be determined by the location of the origin of seizures. Frontal lobe seizures occurred 78% of the time in sleep, while only 20% of temporal lobe seizures occurred during sleep. This was especially true for mesial temporal seizures (22). However, temporal lobe seizures were more likely to generalize in sleep (23). Furthermore, psychogenic nonepileptic seizures (PNES) do not occur in sleep, so the occurrence of events during sleep helps to diagnose seizures. Hence, sleep can provide a differential diagnosis for PNES from epileptic seizures (24).
Chronobiology of Seizures
The pattern of seizure occurrence during sleep has been further evaluated based on circadian rhythm. Tonic, tonic–clonic, gelastic, and hypermotor seizures occurred more frequently in sleep, while clonic, absence, atonic, and myoclonic seizures occurred in wakefulness. Epileptic spasms had two peaks at 6 a.m. to 9 a.m. and 3 a.m. to 6 p.m. in wakefulness (25). Frontal lobe seizures occurred between 11 p.m. and 5 a.m. while temporal lobe seizures occurred mainly between 11 a.m. and 5 p.m. (26). In another study, seizure peaks were seen at 4 a.m. to 7 a.m. for parietal and frontal, 4 p.m. to 7 p.m. for occipital and bimodally at 7 a.m., and 4 p.m. to 7 p.m. for temporal lobe seizures (27). To prove that this occurrence at specific times was not just based on clock times but truly related to a circadian cycle, a study evaluated these in respect to dim light melatonin onset (DLMO). Temporal seizures occurred most frequently during times 6 hours before DLMO and frontal seizures mainly in 6 to 12 hours after the DLMO (28). As the seizure occurrence times can be biased due to the epilepsy monitoring unit environment, a study evaluated the seizure occurrence in patients at home by using ambulatory EEGs. The results showed that frontal lobe seizures occurred more frequently between 12 a.m. and 12 p.m. and temporal lobe seizures occurred more frequently between 12 p.m. and 12 a.m. Moreover, frontal lobe seizures clustered between 5:15 and 7:30 a.m. while temporal lobe seizures clustered between 6:45 and 11:56 p.m. (29). Furthermore, one of the factors in influencing daytime seizure occurrence is the presence of sunlight. Complex partial seizures were less likely to occur on bright sunny days than dull days (30). This may open up the potential for light therapy for treatment of seizures, although further studies are needed (31).
In addition to the circadian pattern of seizure occurrence, medication metabolism also follows the circadian pattern (32). This is helpful in designing treatment strategies for epilepsy. A study suggested that differential dosing with two-thirds dose in the evening for predominantly nocturnal seizures led to seizure freedom in 64.7% of patients, while 88.2% experienced more than 50% reductions in seizures (33). There was a mild increase in nocturnal peak levels as compared to daytime levels with the differential dosing. This approach may also be helpful in reducing the side effects of antiepileptic drugs (AEDs).
The Effects of Sleep Deprivation
A number of studies have shown that sleep deprivation increases IEDs. In various studies performed under different conditions and populations, 11% to 50% of patients have activation after sleep deprivation. The activation is highest in children under 10 years of age. It is believed that sleep deprivation increases cerebral irritability and the occurrence of IEDs is independent of the presence of sleep on EEG recording (1). Hence, sleep-deprived EEGs are commonly performed by most EEG laboratories. Moreover, sleep deprivation is one of the most commonly reported seizure precipitating factors in children with intractable epilepsy (34). Additionally, patients with juvenile myoclonic epilepsy (JME) are very sensitive to seizure occurrence due to sleep deprivation. Sleep deprivation is also known to induce seizures in certain epilepsies and in patients with no history of seizures (35–37). Hence, sleep deprivation is commonly used as a seizure precipitant in epilepsy monitoring units.
SLEEP-RELATED EPILEPSY SYNDROMES
Certain epilepsy syndromes are closely related to the sleep–wake cycle or occur depending on sleep state or arousal from sleep. These are termed sleep-related epilepsies, which include West syndrome, Panayiotopoulos syndrome, BECTS, ESES and related disorders, JME, and nocturnal frontal lobe epilepsy (NFLE). These syndromes are discussed in detail in other chapters and the relationship with sleep is discussed in what follows.
West Syndrome
The syndrome is characterized by epileptic spasm (tonic seizures), hypsarrhythmia, and developmental delay. The classic EEG pattern of hypsarrhythmia is only seen in NREM sleep at the onset of the disorder and sometimes exclusively. The tonic seizures/epileptic spasms occur soon after awakening. These associations are important to know as a sleep EEG will be needed to diagnose cases in early stages of the presentation (38).
Panayiotopoulos Syndrome
This is an age-related benign focal epilepsy syndrome with seizures characterized by nausea, visual changes, and prominent autonomic symptoms with preserved consciousness. The majority of seizures occur in sleep (70%) or on awakening (13%). It is important to know the presentation in sleep and not to confuse these events with other nocturnal events such as nightmares or parasomnia (38).
Benign Epilepsy With Centrotemporal Spikes
BECTS or benign rolandic epilepsy is the most common epilepsy in childhood. About 70% of seizures occur in sleep. Additionally, the IEDs are amplified in NREM sleep. Hence, sleep EEG may be needed to identify the characteristic EEG pattern of spike-and-wave discharges in the frontocentral and temporal head region occurring independently or synchronously with a horizontal dipole (38).
Electrical Status Epilepticus During Sleep and Related Disorders
ESES, continuous spike-wave discharges during sleep (CSWS), and Landau–Kleffner syndrome represent a group of disorders that are characterized by spike-and-wave discharges occurring almost continuously during slow-wave sleep and some form of cognitive decline. As the name suggests, sleep EEG abnormalities are an integral part of the disorder; hence, overnight sleep EEG is needed for diagnosis and prognosis (38).
Juvenile Myoclonic Epilepsy
JME is a common genetically mediated epilepsy syndrome. It is very closely related to sleep as sleep deprivation is a strong trigger for seizure occurrence. Additionally, classic myoclonic seizures occur during awakening and sometimes progress to generalized tonic–clonic seizures. Additionally, on EEG, bursts of generalized discharges are seen during the early morning and close to awakenings. Hence, sleep hygiene and treatment of sleep disorders are very important in patients with JME (38).
Nocturnal Frontal Lobe Epilepsy
NFLE represents an epilepsy syndrome that is often misdiagnosed as parasomnia or nonepileptic events. The seizures may vary from brief tonic posturing to complex motor movements but are characteristically stereotypic for an individual patient. An autosomal dominant type exists, which is called autosomal dominant nocturnal frontal lobe epilepsy (ADNFLE) and is associated with mutations in the nicotinic acetylcholine receptor (CHRNA4) and other genes (KCNT1 and DEPDC5) (38,39). The differentiating features from parasomnia are discussed in the following text.
EFFECT OF SEIZURES AND EPILEPSY ON SLEEP
Seizures disrupt sleep, especially when nocturnal. Daytime seizures reduce REM sleep the following night. Nocturnal seizures cause significant reduction of REM sleep, sleep efficiency, and increased N1. REM sleep and sleep efficiency are further reduced if seizures occur before the first REM cycle. This leads to overall reduction in total sleep time (40). Moreover, increased wake times in sleep have also been reported with nocturnal seizures (41).
Epilepsy also affects sleep macro- and microarchitecture. Epileptic K-complexes with spikes before and/or after a K-complex have been described (42). These were more likely in temporal lobe epilepsy and were associated with arousals (22). In terms of sleep macroarchitecture, increased N1 and decreased N3 and REM were seen in adults with epilepsy (43). Additionally, increase in sleep instability and sleep fragmentation was seen (41). This fragmentation was more common in temporal lobe epilepsy as compared to frontal lobe or generalized epilepsy even though nocturnal seizures are more common in frontal lobe epilepsy (41,44). In children with refractory focal epilepsy, decreased time in bed, decreased total sleep time, and increased N3 were seen (45). In children with generalized epilepsy, increased N1% and REM sleep latency were seen (46). Similar results have been reported in children with epileptic encephalopathies (47), refractory epilepsy with a brain lesion (19), and tuberous sclerosis (48). As discussed earlier, poor quality of sleep essentially creates a sleep-deprived state, which then can precipitate seizures. Additionally, reduction in REM sleep and increased N1 sleep also perpetuate seizures, which further reduces REM sleep. On the other hand, treatment of nocturnal seizures improved sleep. In patients with temporal lobe epilepsy, on seizure-free nights, only mildly reduced sleep efficiency was seen (40). Additionally, after successful treatment of seizures, daytime sleepiness improved (1).
EFFECT OF ANTIEPILEPTIC TREATMENTS ON SLEEP AND SLEEP DISORDERS
It is well known that AEDs affect sleep both in health volunteers and in patients with epilepsy. In healthy adults, carbamazepine reduced sleep latency, arousal, and wake times; in patients with epilepsy, it increased N3. In healthy adults, clobazam reduced sleep latency, arousals, and wake time. Ethosuximide increased N1 and REM sleep but reduced N3 in patients with epilepsy. Gabapentin increased REM sleep in healthy subjects, while in patients with epilepsy it reduced arousals and N1 and increased N3 (ie, stabilized sleep). Lacosamide did not affect sleep architecture in healthy adults (49). Studies evaluating lamotrigine in patients with epilepsy showed conflicting results with either no change or increased N2 and REM sleep and reduced N3. No changes were seen in sleepiness. Levetiracetam, in healthy subjects, increased total sleep time, N2, N3, and decreased WASO and REM sleep. On the other hand, in patients with epilepsy, levetiracetam increased N2 but reduced N3. Conflicting results were seen for changes in sleepiness with levetiracetam; at low doses, it did not affect it, while at doses higher than 2,000 mg/day, it reduced wake time on daytime testing (maintenance of wakefulness test [MWT]). Phenobarbital decreased arousals and REM sleep, and increased N2. In patients with epilepsy, it reduced sleep latency, in addition to the changes in healthy adults. Phenobarbital also increased sleepiness in patients with epilepsy. Phenytoin decreased sleep latency, N2, and REM sleep while increasing N1. Pregabalin, in healthy adults, increased total sleep time and N3 with reduction in arousals. However, in patients with epilepsy, it only reduced N1 and enhanced N3. Studies in healthy adults using tiagabine showed conflicting results for changes in wake times in nocturnal sleep and REM sleep but consistently showed increased total sleep time and N3. Topiramate did not change sleep architecture nor increased sleepiness in patients with epilepsy. Similarly, valproic acid did not change sleep in healthy subjects. However, in patients with epilepsy, it increased N1. In children undergoing weaning from valproic acid, reduced napping was seen. Additionally, it increased sleepiness in other studies. Vigabatrin and zonisamide did not affect sleep architecture or sleepiness in patients with epilepsy (50).
In addition to AEDs, nonmedication treatments of epilepsy also influenced sleep. Vagus nerve stimulation (VNS) improved daytime sleepiness and slow-wave sleep. Epilepsy surgery improved total sleep time and reduced arousals if the seizure frequency was also improved. Ketogenic diet also improved nocturnal sleep. Improved seizure frequency may affect the sleep architecture by itself. Hence, it is possible that some of the effects in patients with epilepsy were related to changes in seizure frequency (50).
Treatments of epilepsy also have been implicated in sleep disorders. The use of vagus nerve stimulator precipitates or worsens obstructive sleep apnea (OSA) and central apneic patterns (51,52). AEDs such as benzodiazepine and phenobarbital have been suggested to worsen OSA (53). Valproate is also suggested to worsen OSA, but this is not consistently proven (54). Felbamate and lamotrigine may worsen insomnia (53). Zonisamide may improve OSA in obese adults with epilepsy by affecting carbonic anhydrase activity and causing weight loss (55).
Hence, antiepileptic treatments significantly affect sleep, so the selection of AED for an individual patient should be customized based not only on side effect profile but also on sleep influences; that is, in a patient with insomnia, gabapentin may be useful. In a patient with higher risk of sleep apnea, proper sleep evaluation and treatment should be undertaken and continued before VNS is implanted and stimulation is increased to rapid cycling. Felbamate and possibly lamotrigine should be avoided or administered early in the evening in patients with insomnia. Phenobarbital and sedative AEDs should be avoided in patients with excessive sleepiness.
SLEEP DISORDERS IN EPILEPSY
Sleep disorders are common in epilepsy and may have a higher prevalence than in the general population.
Obstructive Sleep Apnea
OSA is one of the most commonly recognized sleep disorders with a prevalence of 1% to 13% in children. Higher prevalence is seen in boys, obese children, and in African American children. Symptoms of OSA include habitual snoring (at least 3 nights/week), agitated sleep with frequent awakenings, breathing pauses, and mouth breathing. Additionally, bedwetting, daytime sleepiness, behavioral problems, attention problems, and stuffy nose may also be seen. Signs of OSA may include increased tonsil and adenoid size, increased body mass index (BMI), hypertrophied nasal turbinates, and higher Mallampati grade (56). Polysomnography is the gold standard test for the diagnosis of OSA. Apnea hypopnea index (AHI) of more than 1/hour on polysomnography associated with these symptoms is diagnostic for pediatric OSA (57). Initial treatment, even in obese children, is surgical removal of the adenoids and tonsils (tonsillectomy and adenoidectomy [T&A]), which has a success rate of 85%. Other treatments are positive airway pressure (PAP), dental devices, and palate and/or tongue surgery. Improved behavior, quality of life, and academic performance are noted following treatment of OSA in children (58,59).
OSA is one of the most commonly studied sleep disorders in patients with epilepsy. Various studies in adults suggest increased prevalence of OSA in patients with epilepsy as compared to the general population. This is especially true for adults with refractory epilepsy in whom the prevalence is 1 in 3 (60). In high-risk children with epilepsy as determined by abnormal sleep questionnaires, 80% had obstructive apnea (61). Among children referred to sleep labs, 40% had sleep-disordered breathing (62). Similar to adults, uncontrolled epilepsy was a risk factor for OSA as compared to primary snoring (54). The severity of OSA increased with the increasing number of AEDs (54). Moreover, in children with OSA, poorer sleep architecture and higher desaturations were seen as compared to controls with higher severity of OSA (62).
In studies in adults with epilepsy and OSA, continuous positive airway pressure (CPAP) treatment improved seizure frequency and improved IEDs in wakefulness and sleep in the CPAP-compliant subjects (63,64). In a pilot study, CPAP complaint subjects had a 45% reduction in seizure frequency (65). In a randomized study, 28% of subjects on CPAP had greater than 50% reduction as compared to 15% of controls (66). The odds of 50% reduction in seizure frequency were significantly higher in PAP-treated subjects compared to non-PAP-treated subjects (67). Additionally, one study in children with epilepsy showed a trend toward improvement in seizure frequency after T&A and successful treatment of OSA (68–71).
Central Sleep Apnea
Primary central sleep apnea (CSA) is rare in children past the newborn age, but still exists, especially in children with neurologic disorders. Breathing pauses, daytime sleepiness, and behavioral and attention problems are usual symptoms of CSA. Presence of five or more central apnea and/or hypopneas in the absence of Cheyne–Stokes breathing associated with these symptoms in the absence of other sleep disorders is diagnostic of CSA (57). Types of central apnea include sleep–wake transition apnea, substance-induced central apnea, complex sleep apnea (associated with OSA), and apnea of prematurity. The treatment depends upon the etiology and may include oxygen supplementation and/or PAP therapy.
Limited data exist for CSA in children with epilepsy. A retrospective study suggested that children with abnormal MRI of the brain are at higher risk (72). In adults with epilepsy, CSA was more commonly associated with OSA. Male gender and focal epilepsy were risk factors for CSA (73). Central apneas are also associated with seizures (35), which is discussed later in the chapter in the Sudden Unexpected Death in Epilepsy (SUDEP) section.
Restless Leg Syndrome/Periodic Limb Movement Disorder
Restless leg syndrome (RLS) and periodic limb movement disorder (PLMD) are two separated sleep disorders that frequently coexist in children and are believed to share a common pathophysiologic mechanism and treatment. RLS is described as unpleasant sensations in the legs, causing the urge to move, which are worse during rest or periods of inactivity and improve with movements. The prevalence of RLS is 1.9% of children and 2% of adolescents in the United States and United Kingdom in large population-based studies, with 25% of children and 50% of teens reporting moderate to severe symptoms. In children with RLS, periodic limb movements (PLMs) 5/hour or more are seen in 63% to 74% (74).
PLMD is defined as PLMs on polysomnography with a frequency of 5/hour or more, which causes clinically significant sleep disturbances or impairment in mental, physical, social, occupational, educational, behavioral, or other important areas of functioning and is not explained by any other disorder (74).
Limited data exist regarding the prevalence, risk factors, and prognosis of PLMD in children with epilepsy. In a cohort of children referred to a sleep lab, PLMs/PLMD was found in 5% to 10% (62,75). In adults with epilepsy, 15% had PLMD and 17% had PLMs in sleep (76,77). In adult patients with epilepsy, RLS was identified in 18% to 35% (76,78). No data exist on RLS in children with epilepsy.
Insomnia
Insomnia is not uncommon in children, with a prevalence of up to 11% in 13- to 16-year-old children. The prevalence increases significantly to 30% to 80% of children with severe mental retardation and 50% in children with less-severe cognitive impairment (79). The symptoms of insomnia include difficulty falling asleep, awakenings in night, difficulty waking up in the morning, and daytime drowsiness. Additionally, in younger children insomnia with a larger behavioral component may occur and may be divided into limit setting type (resisting bedtime/sleep) and sleep-onset association type (requiring specific object or person for sleep onset and at each arousal).
Diagnosis is based on clinical assessment and ruling out other sleep disorders. Treatment strategies include behavioral management, cognitive behavioral therapy, and pharmacologic management. Currently, there are no Food and Drug Administration (FDA) approved medication treatments for children with insomnia.
Insomnia is widely underrecognized in children with epilepsy. Prior to referral to a sleep specialist, 82% of surveyed providers used melatonin or a hypnotic for sleep problems (75). Difficulty initiating and maintaining sleep, as well as cosleeping, were more common in children with epilepsy as compared to siblings (80–84). Sleep problems were present in 45% of children with new-onset seizures and were associated with worse neuropsychologic functioning (81). Nocturnal seizures and increased seizure frequency were associated with higher sleep problems (80).
In a retrospective study, insomnia was identified in 11% of children referred to sleep labs (75). Prospective studies in adults showed that 40% to 55% of subjects had insomnia (81,85). Sleep-onset insomnia was seen in 34% while sleep maintenance insomnia was more common, with identification in 52% (78). Insomnia correlated with the number of AEDs, higher scores on depression scales, and poorer quality of life (86).
Only a few randomized studies exist that specifically evaluated the effect of treatment of insomnia in patients with epilepsy. These studies used various doses and formulations of melatonin, which is the most commonly used medication for hypnotic purposes in patients with epilepsy. Melatonin significantly improved sleep as assessed by questionnaires (87). In children and young adults, no improvement was seen on sleep subjectively or objectively; however, seizure frequency reduced significantly (88). In another study, sleep latency was reduced on melatonin on sleep logs (89). A class I study showed that sustained release melatonin significantly improved sleep latency and awake time after sleep onset compared to placebo. A trend toward improvement was seen in sleep efficiency (3.8%) on polysomnography, total sleep time on actigraphy, and sleep duration on sleep diary on melatonin. Moreover, N3% was increased on melatonin, suggesting that melatonin enhanced sleep consolidation. Furthermore, there was a trend toward improvement in epileptiform discharges (90). Hence, the data suggest some scope of improvement in overall quality of life and possibly epilepsy by treating insomnia. Larger studies are needed to confirm this confidently.
Parasomnia
Parasomnias are undesirable physical events or experiences that occur during entry into sleep, within sleep, or during arousals from sleep (39,57). Based on the onset out of the type of sleep, parasomnias are divided into REM and NREM parasomnia. NREM parasomnias occur during the first half of the night from deep sleep or N3. While these are the most common forms of parasomnia in children, primary REM parasomnias are extremely rare in children. There is a strong family history for NREM parasomnia (91). Other sleep disorders, such as sleep apnea, PLMD, and RLS, may precipitate the events in up to 61% of children with sleep terror and sleepwalking, and resolution occurs upon successful treatment of the other sleep disorders (92). NREM parasomnia events reduce with age as the amount of N3 sleep reduces with increasing age. However, a few teenage children have persistent sleepwalking or sleep terrors, and the majority of them have a psychiatric comorbidity (93). Medications that suppress slow-wave sleep, like benzodiazepines, are beneficial and should also include treatment of associated sleep disorders.
Parasomnias are common in children, especially younger children. Hence, just by the mere prevalence of parasomnia, these are also common in children with epilepsy. Provini et al reported 100 cases of NFLE; 34% had a personal history, and 39% a family history, in a first-degree relative, suggestive of parasomnia (94). Hence, differentiation of nocturnal events is important for adequate treatment. Careful evaluation with detailed history and diagnostic testing with prolonged video-EEG monitoring may be needed. Additionally, a sleep study may also be required. Parasomnias are more common in the younger age group, and occur out of N3, while nocturnal seizures, especially NFLE onset, occurs in teenage years, and seizures are more likely to occur out of N1 or N2. The most important differentiating feature is that frontal lobe seizures are brief, and follow a very consistent stereotypic semiology for a given patient. Parasomnias, on the other hand, typically last longer and have different semiology during any given event (95). Hence, multiple nocturnal events for a patient should be evaluated for stereotypy.
Sleepiness
Sleepiness is very common in children with epilepsy. However, the referrals for sleep evaluations are limited for this complaint. In one study, 70% of neurologists attributed sleepiness to AEDs (75). However, prospective studies in adults with epilepsy suggest that sleepiness is correlated with sleep disorders such as sleep apnea and RLS symptoms, habitual snoring, observed apneas and neck circumference, and recurrence of seizures (76,96,97). In these studies, the number of AEDs was not related to sleepiness. Similarly, in children with epilepsy, 46.2% of children with epilepsy had sleepiness. This was associated with symptoms of OSA and parasomnia and not with epilepsy syndrome, AEDs, and the presence or absence of seizure freedom (98).
SUDDEN UNEXPECTED DEATH IN EPILEPSY (SUDEP)
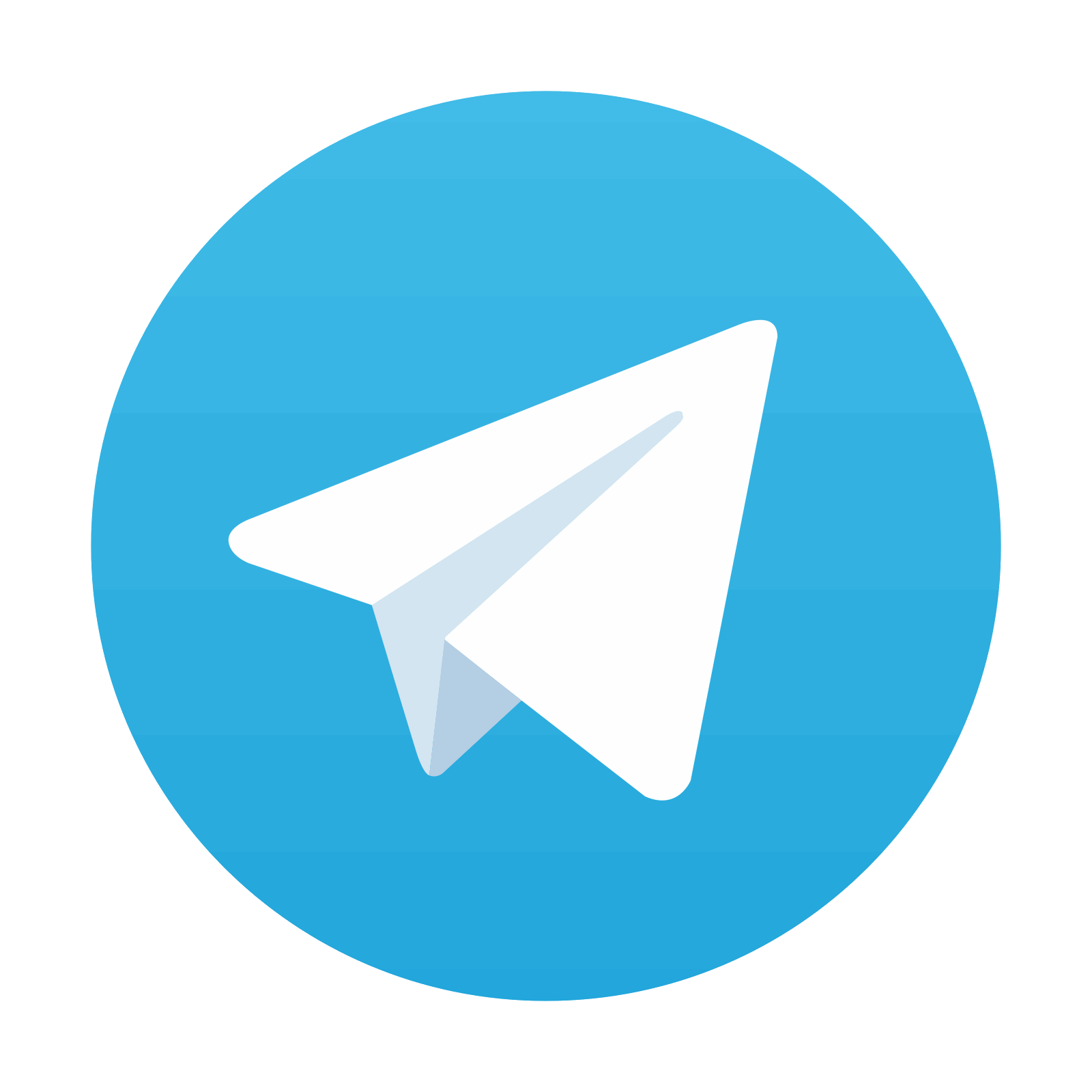
Stay updated, free articles. Join our Telegram channel

Full access? Get Clinical Tree
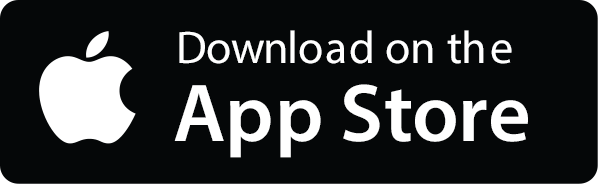
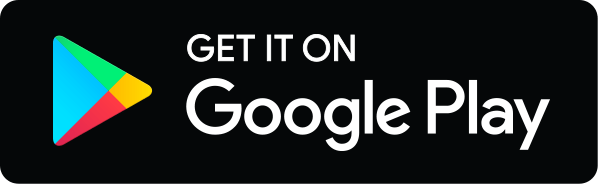