Class
Description
I
The patient is normal and healthy
II
The patient has mild systemic disease that does not limit their activities (e.g., controlled hypertension or controlled diabetes without systemic sequelae)
III
The patient has moderate or severe systemic disease, which does limit their activities (e.g., stable angina or diabetes with systemic sequelae).
IV
The patient has severe systemic disease that is a constant potential threat to life (e.g., severe congestive heart failure, end-stage renal failure).
V
The patient is morbid and is at substantial risk of death within 24 h, with or
E
Emergency status: In addition to indicating underlying ASA status (1–5), any patient undergoing an emergency procedure is indicated by the suffix “E”. For example, a fundamentally healthy patient undergoing an emergency procedure is classified as I–E. If the patient is undergoing an elective procedure, the “E” designation is not used.
Preoperative Anaesthesiological Assessment
Besides the general assessment of the physical status of the patient, as established by the American Society of Anesthesiologists (Table 1), the evaluation of a child who is undergoing a surgical procedure on the CVJ should take into account the possible coexistence of malformations or physiological problems associated to pathogenic conditions to be dealt with. Anaesthesiologists should also not underestimate the importance of the psychological background of the patients and the family, especially in case of repeated surgical procedures and hospitalizations.
Intubation Versus Tracheostomy
The choice whether intubation or tracheostomy would assure the best airway management requires a full agreement between the anaesthesiologist and the surgeon. Such a common decision should be based on the precise preoperative knowledge of the ‘syndromic’ pattern of a given malformation, that is, all the possible anatomical features limiting the manoeuvres of intubation and/or a thorough evaluation of the risk of performing the surgical procedure itself as well as postoperative complications, namely, postoperative upper airway oedema [5]. A further aspect to be taken into account is the choice of the anterior or posterior surgical approach [64].
Micrognathia and mandibular hypo-dysplasia as well as macroglossia constitute an absolute cause of difficult intubation due to reduced anatomical space between the basihyoid bone and the mandible which limits the displacement of the soft parts from the laryngoscopes (during laryngoscopy). Difficult intubation may be encountered also in Goldenhar syndrome and Klippel-Feil syndrome due to the reduced mobility between atlanto-occipital and the cervical vertebrae [52]. Anaesthesia and surgery were associated with worsening of previously unrecognized abnormalities in children with Down syndrome, abnormalities that probably reflected some degree of pre-existing cord compression. Therefore, if there is any suspicion of signs or symptoms consistent with cervical cord compression, a thorough history and physical examination should be conducted [36]. In mucopolysaccharidosis, mainly in type IV (i.e. Morquio syndrome), the macroglossia and the reduction of the upper airway volume play a role in favouring alternatives to classic orotracheal intubation. Airway management in this patients can be complicated by macroglossia, prognathism, and hypertrophy of pharyngeal and laryngeal tissues, which make mask fit, mask ventilation, laryngoscopy, and correct tracheal tube placement difficult [42]. In this and similar pathological conditions, no method of airway assessment is foolproof for predicting the difficult airway; nevertheless, commonly accepted preoperative assessment evaluation does exist, such as the Mallampati classification, which is based on the anatomical structures visualized when patients open their mouths (Table 2).
Table 2
Mallampati score
Class | Anatomy of the oral cavity |
---|---|
Class I | Full visibility of soft palate, tonsils, and uvula |
Class II | Visibility of soft palate but only the upper portion of the tonsils and uvula |
Class III | Soft and hard palate and base of the uvula are visible |
Class IV | Only hard palate is visible |
A Mallampati class of III or IV is predictive of airway management difficulty in acromegalics [59]. In older children, an alternate airway assessment modality is the upper lip bite test (ULBT) in which a patient is asked to cover the vermillion of the upper lip with the lower incisors (Table 3). A higher grade can be predictive of airway management difficulty [27]. The Cormack and Lehane grade, at the time of laryngoscopy, is used to define difficulty with laryngoscopy (Table 4). Patients with a Cormack and Lehane grade 3 or 4 are considered to pose difficulty with laryngoscopy.
Table 3
Upper lip bite test
Grade | Description |
---|---|
Grade 1 | Ability of lower incisors to fully cover the upper lip and extend superior to the vermillion border |
Grade 2 | Lower incisors can bite the upper lip but only inferior to the vermillion border |
Grade 3 | Lower incisors are not able to bite the upper lip at all |
Table 4
Cormack and Lehane grades
Grade | Anatomy of the oral cavity |
---|---|
Grade 1 | Most of glottis visualized |
Grade 2 | Only posterior portion of the glottis is visualized |
Grade 3 | Only epiglottis but no glottic structures are visualized |
Grade 4 | Neither epiglottis nor the glottis is visualized |
In general, tracheal intubation is considered difficult if more than two failed attempts occur in requiring rescue anaesthesiological techniques, e.g. laryngoscope blade change, bougie, video laryngoscopy, and fibre-optic bronchoscope [55]. In this case awake fibre-optic intubation can be strongly advocated in case of surgical procedures on the CVJ but also tracheostomy must be taken into consideration.
Modern fibre-optic technology offers a safe and effective method of airway management. However, it requires great skills and acquired experience and is very difficult to perform with uncooperative patients, especially children [59]. Moreover, this technique may be particularly difficult in relation with retropharyngeal swelling and adenoidal hypertrophy in children.
It is worth to note that in the last 15 years, adequate instruments for fibre-optic bronchoscopy have been introduced in the anaesthesiological armamentarium, thus making easier the application of this technique in the paediatric population, including infants and small children [6], consequently overcoming the difficulties encountered in the past when only ‘adult’ fibre-optic apparatus, 6 mm in diameter, were available. Failure to intubate may necessitate alternative airway control devices such as video-assisted intubation devices or intubation through laryngeal mask airways (LMA). LMA may be particularly useful in this setting, and endotracheal tube placement can be achieved using the fibrescope through the LMA/iLMA as a guide [38]. However, till more evidence is available, there are not strong recommendations for standardized procedures in children with difficult or failed intubation. This is the reason why for a continuous search of better intubating devices such as, for example, the video laryngoscopes, are able to provide a better laryngeal view as compared to direct laryngoscopy [69].
The decision to intubate or perform a tracheostomy is essentially made by estimating the risk of upper airway oedema that may occur postoperatively. If excessive oedema is expected, a tracheostomy should be taken into consideration to assure a controlled and stable airway in the postoperative period. This would permit while allowing an early wake-up, useful to obtain an immediate neurological postoperative evaluation and prevent from harmful consequences of a prolonged tracheal intubation [58].
Although tracheostomy and tracheotomy are used interchangeably, it should be reminded that tracheostomy from the Greek word stomoun refers to the act of providing an opening, whereas tracheotomy, from the Greek word tome, to cut, means specifically the result of surgical cut. The need of cervical external orthosis, that is, a Halo Vest system, should suggest to proceed to the intervention of tracheostomy in order to prevent the necessity of performing an emergency procedure in the postoperative phase due to overwhelming respiratory distress.
The Choice of the Surgical Route
Two surgical routes can be chosen by the paediatric neurosurgeon, the anterior dealing with direct decompression of the cervical spinal cord and the posterior dealing with both neurological decompression and instrumentation and fusion of the cervical spine [67].
The Anterior Route
Orotracheal intubation can be chosen in case of endoscopic transnasal approach since no conflict with the surgeon activity is provoked. The choice of orotracheal intubation can be more debatable in case of transoral, transmandibular, or transmaxillary approaches as some surgeons do not mind about the possible conflict of pharyngeal spreaders of the oral distractor with the orotracheal tube; others prefer a nasotracheal intubation performed by the anaesthesiologist, so reducing the possible conflict with the oral distractor, while others prefer to keep the surgical channel free of any foreign body by using tracheostomy (Figs. 1 and 2).
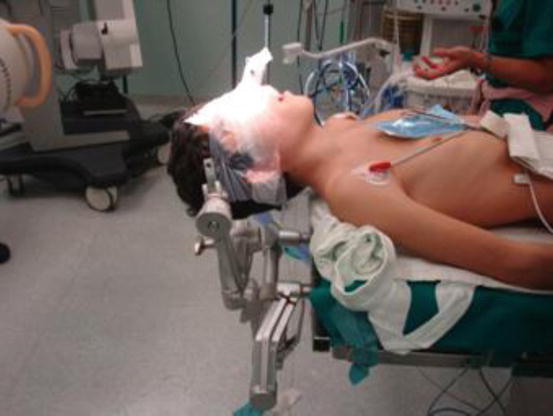
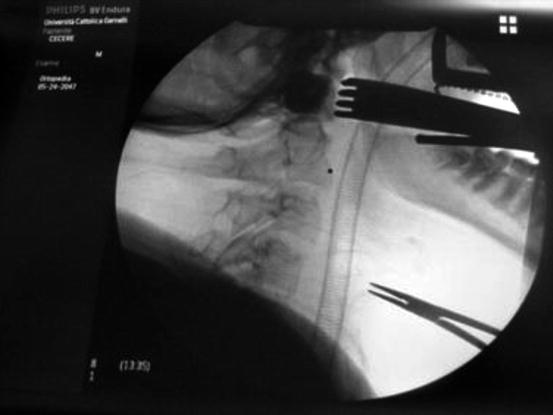
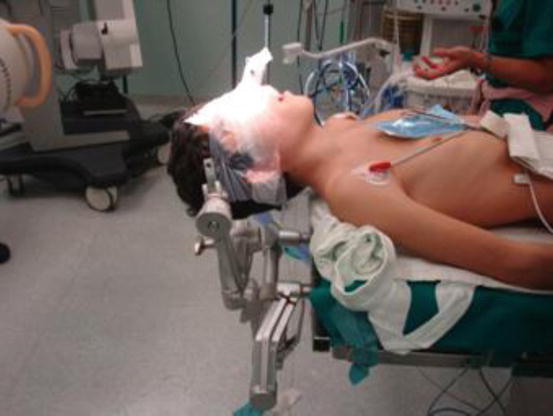
Fig. 1
Endonasal intubation, head fixation with Mayfield head holder, and oral distraction with Crockard distractor (Codman J&J) for anterior transoral decompression
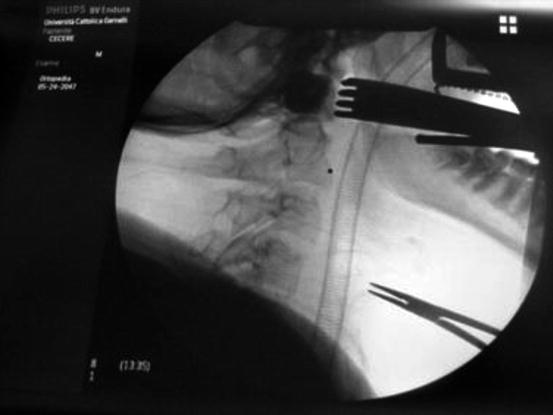
Fig. 2
Intraoperative assessment of Crockard distractor visualizing the nasotracheal tube (the black square is the operative ‘minus’ filled of contrast medium (Iopamiro) to visualize the decompression
The Posterior Route
In case of posterior surgical approach (decompressive and instrumentation and fusion techniques), tracheostomy is not obtained for surgical requirements, since the airways are far from the surgical target. Nevertheless, in case of pre-existing brainstem damage which could suggest an occurrence of postoperative respiratory disturbances, along with the need of a Halo Vest system, tracheostomy could be advocated (Figs. 3 and 4) [64, 65, 66].
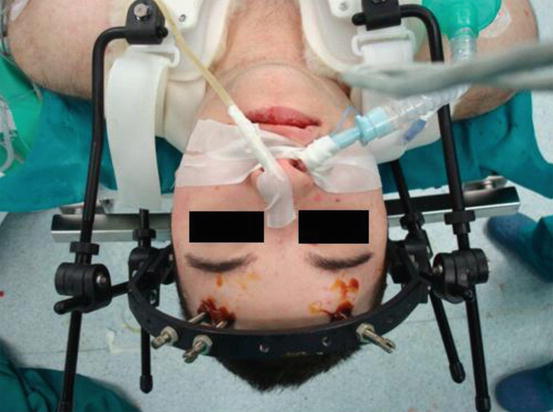
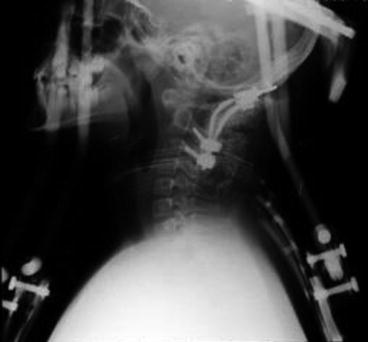
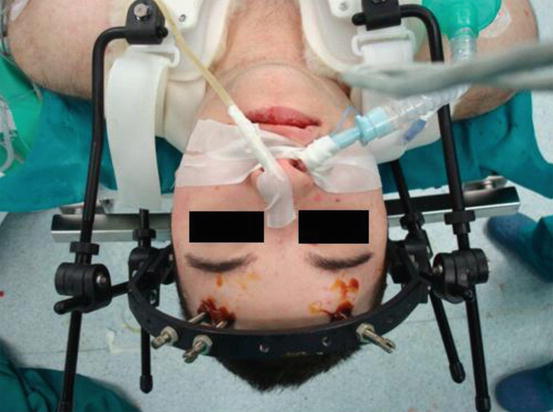
Fig. 3
After operation, the patient is fixed with a paediatric external orthosis Halo Vest (De Puy Spine Bremer). Nasogastric tube in the left nostril and nasotracheal tube in the right nostril are shown
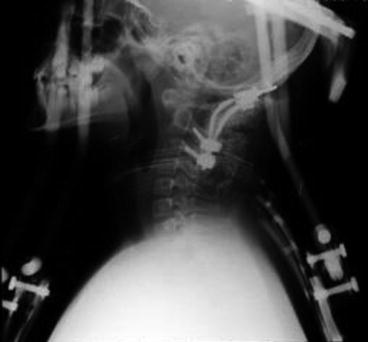
Fig. 4
Postoperative X-ray control of external orthosis after double-staged instrumentation and fusion procedure. Note the airway’s patency and chest expansion with the vest
Sedation and Drug Delivering
Sedation is necessary to evaluate spontaneous breathing and ventilation. When the oxygenation and ventilation are adequate, it is possible to proceed to laryngoscopy after induction with administration of not irritating inhalational agents (sevoflurane) or intravenous drugs (propofol and remifentanil). In cases where difficult intubation is anticipated, it is useful to consider the use of a fibre-optic bronchoscope. Indeed, fibre-optic techniques have gained greater use in selected paediatric patients following a combination of topical anaesthesia, local airway blocks and IV sedation, or under general anaesthesia by mask with maintenance of spontaneous ventilation [44]. The ideal size of fibrescope depends on the diameter of the endotracheal tube to be passed through. Intubation requires an ETT size that is only slightly larger than the diameter of the fibrescope. The modern flexible fibrescopes arrive to a diameter of 2 Fr and allow the passage of endotracheal tube inside diameter of 2.5 Fr.
Providing moderate levels of sedation without causing respiratory distress or haemodynamic instability, dexmedetomidine could be successfully used in patients requiring awake fibre-optic intubation for cervical spine surgery [3, 33]. In all patients, electrocardiography pulse oximetry and blood pressure recordings must be monitored throughout the procedure. The maintenance of anaesthesia is obtained with Sevorane (MAC 1, 1.5) in O2 plus air with a paCO2 target level of 32–35 mmHg. Muscle relaxation is obtained with cisatracurium besylate.
Intraoperative Neurophysiological Monitoring
Intraoperative monitoring of spinal cord function is considered a standard of care in spinal surgery [54]. The methods of monitoring the integrity of the spinal cord function intraoperatively include:
1.
The wake-up test
2.
Somatosensory evoked potentials (SSEP)
3.
Motor evoked potentials (MEPs)
4.
Dermatomal responses
The Wake-Up Test
Before the use of electrical monitoring, this test was a method of assessing spinal cord function during corrective procedures of the spine. The hazards of this test include accidental extubation, dislodgement of fixators and air embolism on deep inspiration. Its major limitation is that spinal cord function is evaluated only at one specific time (i.e. during the wake-up test) and not continuously during the procedure. Both inhalation and i.v. anaesthetic techniques are suitable for the ‘wake-up’ test. Furthermore, a standard wake-up test cannot be performed in infants and small children. Alternatives such as tetanic stimulation of the lower legs and observation of the clinical response can be tried in this age subgroup.
SSEP and MEPs
Somatosensory evoked responses represent the more widespread method of electrical monitoring of the spinal cord [30]. The response of constant current stimulation of the median, tibial, and, rarely, the sural nerve is recorded at the level of the cortex using surface scalp electrodes or a bipolar electrode placed epidurally. Baseline SSEPs are recorded in order to exclude neurological dysfunction and also to determine the feasibility of the operative monitoring. Neurophysiology technicians monitor the latency and amplitude of the recordings continuously during anaesthesia and surgery. A 0.50 % decrease in SSEP amplitude is deemed significant. Numerous anaesthetic agents interfere with the latency and amplitude of the SSEP. Surgical stimulation level and hypothermia also interfere with SSEP. Volatile agents, cold, hypoxia, hypercarbia, and spinal ischaemia suppress both SSEPs and MEPs. Responses recorded from the cerebral cortex are more sensitive to the effects of anaesthetic agents than epidurally recorded responses. Neuromuscular block reduces muscle artefact on the recorded SSEPs. SSEP monitoring is a pathway-specific method; an injury not involving the sensory pathway will not be detected. In cervical spine surgery, SSEPs and MEPs should be used. Although MEPs are still not available in many intraoperative neurophysiological departments, their use should be encouraged since MEPs represent the most appropriate and reliable tool to assess the functional integrity of descending motor tracts [53].
Dermatomal Potentials
Dermatomal somatosensory evoked potentials (DSEPs) involve recording cerebral evoked responses from cutaneous stimulation of areas of known dermatomal innervation providing a pure sensory input to any level of the spinal cord. However, given the lack of evidence in the literature, current evidence is insufficient to determine appropriateness.
Perioperative Fluid and Blood Volume Management
Intraoperative fluid therapy may start at the time of the surgical treatment as simply as replacing the deficits of the preoperative fasting status and maintenance of normal fluid condition or as complex therapy need at correcting preoperative abnormal deficits. During the surgery, care should be given to compensate intraoperative translocated fluids and variable blood loss.
Because of their high metabolic rate and water turnover, significant hypoglycaemia and dehydration may occur in infants who are required to fast preoperatively for prolonged periods of time. If not provided previously, the fluid deficit occurred during fasting should be replaced during anaesthesia. Assuming that a healthy child is in water and electrolyte balance at the time oral feedings are discontinued, the fluid deficit at the start of anaesthesia can be estimated by multiplying the child’s hourly maintenance fluid requirement by the number of hours that have elapsed since the last feeding. This deficit may be replaced by giving half of the calculated volume during the first hour of anaesthesia and the other half over the next 2 h, in addition to intraoperative maintenance fluids. Five percent dextrose in quarter-normal saline (5 % dextrose/0.25 NS) is frequently used for fluid maintenance.
Conflicting data do exist in the literature about the intraoperative use of glucose. The metabolic need for glucose in the infant age group is 5 g/kg/day. Infants under 4 years of age who are kept fasting for over 6 h may become hypoglycaemic and irritable. Since the metabolic rate and oxygen consumption of infants and small children is significantly greater than older children and adults, it has been recommended to provide 5 % dextrose for all infants at risk of hypoglycaemia (serum glucose <40 mg/dl) from preoperative starvation. It must be remembered, however, that preterm infants receiving glucose infusions should be monitored carefully for hyperglycaemia, since their kidneys are unable to hyperfiltrate in the presence of hyperglycaemia. Hyperglycaemia (glucose >200 mg/dl) has also been noted in older infants and children given 5 % dextrose in lactated Ringer’s as an intraoperative maintenance fluid. Elevated blood glucose concentrations may lead to an osmotic diuresis and may exacerbate neurological injury associated with a cardiac arrest or other cerebral insults (i.e. cerebral ischaemia and asphyxia). The clinical significance of postoperative hyperglycaemia in otherwise healthy children would seem to be minimal. However, in major surgical cases, such hyperglycaemia and osmotic diuresis may complicate or confuse ongoing fluid therapy. Therefore, we can consider blood glucose control as a reasonable goal for the majority of the cases and a critical goal in a few children. Blood glucose control can be achieved in several ways. Most children receiving glucose-free intravenous fluids during surgical procedures demonstrate an increase in their blood glucose concentration. Physiological factors accounting for the hyperglycaemic response to anaesthesia and surgery include increased sympathoadrenal activity leading to a decrease in glucose tolerance, decreased glucose utilization, and increased gluconeogenesis. However, about 12 % of patients who receive glucose-free solutions fail to increase their blood glucose concentrations. Since hypoglycaemia may be undetected during anaesthesia, it would seem prudent to measure intraoperative glucose concentrations if glucose-free solutions are used and to treat hypoglycaemia if diagnosed. It seems paradoxical, however, to be depending on the stress of surgery to increase glucose at a time when ‘stress-free’ anaesthetics are being advocated. Therefore, in general, we advocate the use of glucose-containing solutions. One way to avoid hyperglycaemia with a 5 % dextrose solution is to decrease the infusion rate, but this may contribute to hypotension in fluid-depleted children. A less concentrated glucose solution (e.g. 2.5 % dextrose in lactated Ringer’s) given at recommended infusion rates provides adequate glucose for healthy children (300 mg/kg/h) and adequate volume replacement while avoiding hyperglycaemia and hypoglycaemia. Since 2.5 % dextrose in lactated Ringer’s is not commercially available, it can easily be made by the individual practitioner with 50 % dextrose solution.
Intraoperative Third-Space Losses
Surgical traumas are associated with the isotonic transfer of fluids from the extracellular fluid compartment (and to a lesser extent from the intracellular compartment) to a nonfunctional interstitial compartment. This acute sequestration of fluid to a nonfunctional compartment has been called third-space loss and should be replaced. In neurosurgery, it is negligible (1–2 ml/kg/h) [16]. Generally, lactated Ringer’s solution or normal saline is used to restore third-space losses.
Blood and Blood Component Therapy
Paediatric patients undergoing major procedures (e.g. tumour resection, reconstructive surgery, cardiac surgery, organ transplantation, trauma surgery, or surgery for burns) will invariably require some form of blood volume replacement. Indications for blood or blood component therapy are not always clear-cut; hence, the anaesthesiologist should formulate a preoperative plan of management utilizing scientific rationale for a decision on blood therapy. Factors on which this decision is based include the patient’s blood volume estimates, preoperative haematocrit, general medical condition, and ability to provide adequate oxygen transport to the tissues, as well as the nature of the surgical procedure and the risks vs. benefits of transfusion [49, 62]. All blood loss in neonates, infants, and children should somehow be replaced. An accurate measurement of blood loss and an accurate assessment of acceptable blood loss in the child are vital to any replacement regimen. Calculation of sponge weight, the use of calibrated miniaturized suction bottles, and a visual estimation (combined with a ‘guess factor’) make it possible to define the magnitude of the blood loss. The transfusion practice guidelines of the American Society of Anesthesiologists Task Force on Blood Component Therapy [3] are largely applicable to paediatric patients without cardiopulmonary disease. The concept of allowable red-cell loss or allowable blood loss, preferable as a guide to blood replacement normovolemic haemodilution to a predetermined haematocrit, can be achieved with crystalloid or colloid solutions. Blood volume is age-related: The neonatal blood volume has been reported to vary widely from 65 to 129 ml/kg, depending on the magnitude of the placental transfusion [17].
Estimating Allowable Blood Loss
Several methods have been proposed for estimating allowable blood loss from the blood volume, weight, and haematocrit. The formulas range from the simple to the complex, but all involve an estimate of blood volume. The safest haemoglobin or haematocrit that is allowed during surgery is debatable and clearly depends on the type of surgery, the patient’s postoperative activity, and his/her underlying medical condition. As a general rule, we accept haematocrits of 28–30 as the lowest acceptable haematocrit. In the newborn, 40 % is the lowest acceptable haematocrit. A simple method of calculating allowable blood loss (ABL) uses the original haematocrit (Ho), the lowest acceptable haematocrit (H1), and the average of the two haematocrits
as follows [9]:
![$$ \text{ABL}=\text{Wt}\times \text{EBV}\times \left[\text{Ho}-\text{H}1\right]/\left[\text{H}\right]$$](/wp-content/uploads/2016/12/A978-3-319-01065-6_5_Chapter_TeX2GIF_Equa.gif)

![$$ \text{ABL}=\text{Wt}\times \text{EBV}\times \left[\text{Ho}-\text{H}1\right]/\left[\text{H}\right]$$](/wp-content/uploads/2016/12/A978-3-319-01065-6_5_Chapter_TeX2GIF_Equa.gif)
In this equation, all haematocrits are decimal values (i.e. 0.6, 0.5). There is controversy as to whether the blood volume should be supported with crystalloid or colloid, while the haematocrit is being allowed to decrease. If colloid (i.e. albumin, fresh frozen plasma) is used, then ongoing blood loss is replaced millilitre for millilitre. If isotonic crystalloid is administered, Furhman et al. recommend replacing ongoing blood loss with an equal volume of lactated Ringer’s solution until the serum protein concentration approaches 5 g/dl; after this point, blood volume is maintained with 5 % albumin until the allowable blood loss has been exceeded [56]. Others replace blood loss with 2–4 times the volume of lactated Ringer’s solution. However, with these large volumes of crystalloid replacement, hypoproteinaemia may result. We replace blood loss with volumes of crystalloid 2 times the measured or calculated loss. In major surgery involving 1–2 body cavities, intravascular albumin may be transiently depleted or translocated. Consequently, we follow serum protein levels and begin replacing with 5 % albumin when serum protein levels approach 5.0 g/dl.
Blood Component Therapy
Blood component therapy depends on the clinical setting and the availability of various blood products. Bleeding from thrombocytopaenia is rare with a platelet count of more than 50,000; serious bleeding is usual below 20,000. Normal adult values for clotting factors are attained between 1 and 12 months of age, depending on the specific factor. Fresh whole blood (less than 4 h old) has a limited availability. If predicted blood loss is greater than or equal to 40 % of blood volume, it is helpful to supply platelets and clotting factors. Packed red blood cells have a haematocrit of between 70 and 80 %. An average of 1 ml/kg packed cells increases the haematocrit by 1.5 %. The units of packed cells can be subdivided into paediatric packs of 80–100 ml. The fluid of these cells is relatively hyperkalaemic (K+, 15–20 mEq/l), acidotic (pH<7.0), and low in ionized Ca++. When the maximum allowable blood loss is approached, consider transfusion with packed fresh red blood cells (PRBC) to adjust the haematocrit to about 30 %. When the amount of blood lost approaches one blood volume, labile clotting factors are greatly reduced; normal clotting requires 5–20 % of factor V and 30 % of factor VIII. All of the coagulation factors except platelets are present in normal quantities in fresh frozen plasma.
We prefer to give 2:1 volumes of lactated Ringer’s solution and packed cells (haematocrit 35–40 %) to patients with massive blood loss (i.e. >1 blood volume). This ratio of cells and crystalloid can be varied to produce any desired haematocrit. In patients with clinical signs of bleeding or documented abnormal clotting studies, crystalloid is replaced with fresh frozen plasma. The FFP is then administered with equal volumes of packed cells. The therapeutic value of FFP is related to volume expansion and provision of procoagulants. FFP contains 70 % or more of the procoagulant factors of fresh plasma [57] and is best utilized to replace depleted clotting factors in patients with von Willebrand’s disease, haemophilia A, and liver disease. It is also used in association with massive blood transfusion and perhaps DIC. The latter two conditions do not always represent clear-cut indications for FFP transfusion. Guidelines for FFP administration include reversal of oral anticoagulant, replacement of factor deficiencies as determined by the laboratory, treatment of immunodeficiencies, treatment of thrombotic thrombocytopaenia purpura, treatment in antithrombin III deficiency, and massive blood transfusion (when factors V and VIII are less than 25 % of normal) [41]. The use of FFP carries with it risks of transfusion transmitted diseases, as is the case with any blood product [3]. The need for platelets intraoperatively may be predicted from the preoperative platelet count. Platelets can be mobilized from the spleen and bone marrow as bleeding occurs. An infant with a high preoperative count (>250,000) may not need a platelet transfusion until 2–3 blood volumes are lost, whereas an infant with a low count (<150,000) may need platelets after only 1 blood volume is lost. One platelet pack per 10 kg is usually adequate.
Postoperative Care
Admission in PICU shortly after the CVJ surgery is mandatory to ensure haemodynamic and respiratory stability and for prompt recognition of postoperative complications and management of airways.
Postoperative Fluid Management
Fluid management is carefully monitored, avoiding fluid overload as well as hypovolaemia. It is advisable to give crystalloids (normal saline 0.9 % NaCl or Ringer’s lactate) in order to allow an adequate glycaemic level. It has been documented that hyperglycaemia is common in critically ill children and is associated with mortality and morbidity in PICU [1, 39]. However, the actual relevance of strict glycaemic control is particularly controversial in the context of neurocritical care. In the first 24 h after neurosurgery, hyperglycaemia is observed in many children, even in the absence of glucose infusion. After traumatic brain injury (TBI), hyperglycaemia has been associated with poor outcome, explained either by a more severe TBI or by the neurotoxicity of glucose [7, 60]. Hyperglycaemia increases oxidative stress and inflammation, augments excitotoxicity mediated by NMDA receptors, and alters microcirculatory perfusion. On the other hand, brain metabolism depends on a constant supply of glucose, and hypoglycaemia also favours excitotoxicity, apoptosis, and oxygen free-radical accumulation. Neuroglycopaenia and metabolic crisis can occur with even mild hypoglycaemia. It is therefore advisable to keep blood glucose levels less than 150 mg/dl through insulin infusion and, at the same time, prevent hypoglycaemia by adding glucose solution when serum glucose falls below 70 mg/dl [25].
Prophylactic and Supportive Measures
Short course antibiotic prophylaxis is initiated just before the operation. Guidelines recommend that antibiotic prophylaxis should be given only at premedication, but in clinical practice, antibiotic prophylaxis is often unnecessarily prolonged through the next few days. Prophylaxis against stress ulcers should be recommended for the prevention of upper gastrointestinal bleeding in these patients. A systematic review and meta-analysis found limited high-quality data to recommend routine use of stress ulcer prophylaxis [47]. At the present time, stress ulcer prophylaxis using ranitidine, sucralfate, or proton-pump inhibitors (PPIs) is instituted in children with risk factors for development of stress ulcers.
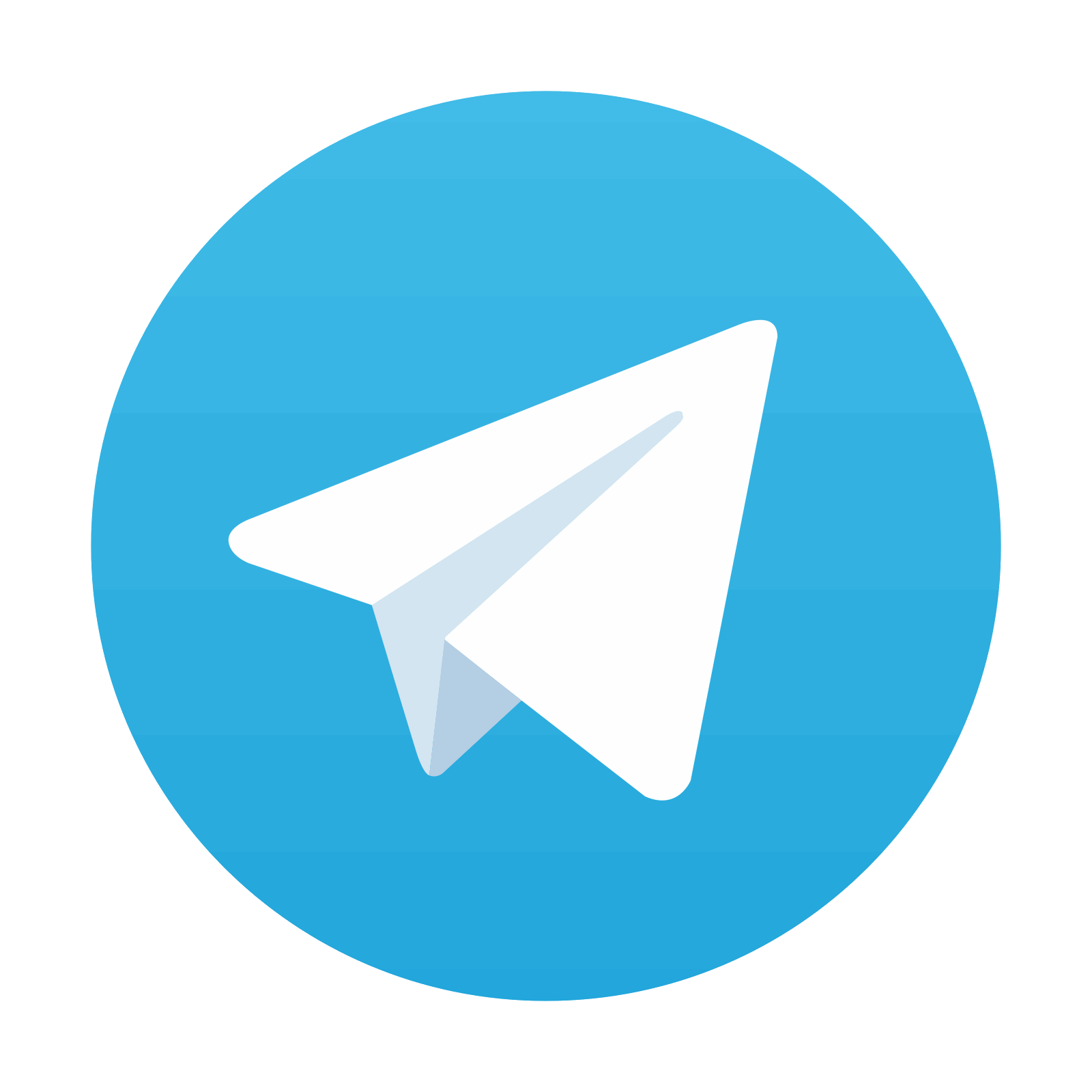
Stay updated, free articles. Join our Telegram channel

Full access? Get Clinical Tree
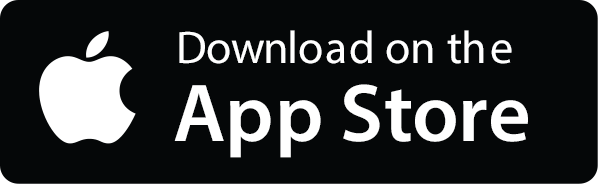
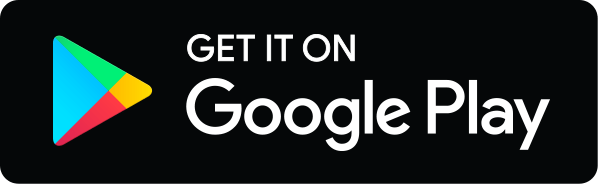