Levetiracetam (LEV) and brivaracetam (BRV) are analogues of piracetam. This series of drug molecules was derived from a γ-butyrolactam ring structure. LEV, (S)-2-(2-oxopyrrolidin-1-yl)butanamide (Figure 58.1), is an antiepileptic drug (AED) chemically related to the nootropic (cognition-enhancing) agent piracetam, derived by the addition of an ethyl moiety to the carboxamide side chain of piracetam (1). BRV, (2S)-2-[(4R)-2-oxo-4-propylpyrrolidin-1-yl]butanamide, on the other hand, has been derived by the addition of a 4-n-propyl side chain to the structure of LEV (Figure 58.1). As piracetam was known to suppress myoclonic jerks (2), similar drugs were evaluated for anticonvulsant efficacy, leading to the discovery and development of LEV, BRC, and other congeners that were not further developed for clinical use as AEDs.
LEV has been available in the United States since 2000 and is marketed under the trade name Keppra®. It is currently indicated as adjunctive therapy in the treatment of partial-onset seizures in adults and children 1 month of age or older, and primary generalized tonic–clonic seizures in patients 6 years of age and older with idiopathic generalized epilepsy (IGE). It was recently approved for adjunctive treatment of myoclonic seizures in adolescents and adults 12 years of age and older with juvenile myoclonic epilepsy. However, it is used as monotherapy and in other epilepsy types. BRV received approval by the U.S. Food and Drug Administration (FDA) in February of 2016 for the treatment of focal onset seizures in patients 16 years and older under the brand name Briviact®, and at the time of this writing awaits DEA classification.
LEVETIRACETAM
Preclinical Studies
The pharmacologic activity of LEV has been evaluated in rats and mice. LEV is not active in the classical acute models of maximal electroshock (MES) and pentylenetetrazole (PTZ) seizures in mice (3). However, LEV does display potent and selective protection against kindled seizures and kindling acquisition in a broad range of animal models of chronic epilepsy (3–5). This profile distinguishes LEV from most other AEDs, which demonstrate similar activity in both acute and chronic seizure models (3).
The fact that LEV protects animals that have been made epileptic via kindling establishes its anticonvulsant activity (4). However, in addition to its anticonvulsant activity, LEV may also be antiepileptogenic, as suggested by the fact that LEV exposure retards the acquisition of kindling (5). It is important to note that this type of antiepileptogenic activity has been demonstrated by some newer AEDs (6–8), but not by others (9).
LEV also has a high safety margin, as evidenced by a remarkable separation between the dose that protects against convulsions and the dose that impairs rotarod performance. This observation was born out of data in corneal electroshock–kindled mice and genetic absence epilepsy rats from Strasbourg (GAERS), which showed a high therapeutic index for LEV (3). Additionally, LEV demonstrates activity in animal models believed to represent generalized epilepsy. LEV has been shown to afford protection against seizures induced by acoustic stimulation in sound-sensitive mice (10) and rats (11) and partial protection against spike-and-wave discharges in GAERS (11). Levetiracetam was able to terminate self-sustaining status epilepticus in rats and displayed synergy with diazepam in this model (12). Some limited animal data also indicate neuroprotective effects of LEV in models of status epilepticus (13,14).
There has also been interest in the possible neuroprotective effects of LEV in the neonatal brain, particularly in hypoxic-ischemic injury. Kilicdag et al (15) exposed 7-day-old Wistar rat pups to right common carotid artery ligation and hypoxia for 2 hours, followed by treatment with LEV or saline. A sham group did not undergo ligation or hypoxia. Apoptotic cell counts were found to be similar in the sham group and the LEV treatment group. There was also a significant decrease in apoptotic cells in the LEV-treated group compared to the saline group. This suggests that LEV administration after hypoxia may reduce neuronal apoptosis in the developing brain (15). This effect may be enhanced during hypothermia, while there is some suggestion that hypoxic-ischemic brain injury in the neonatal rodent brain may be exacerbated by LEV in normothermic conditions (16), although the exacerbation discerned under normothermia was not considered significant.
FIGURE 58.1 Chemical structures of Levetiracetam and Brivaracetam. Brivaracetam differs from Levetiracetam by the presence of the propyl group in the 4-position of the pyrrolidine ring.
Mechanism of Action
Fortuitously, LEV was discovered to have antiepileptic properties prior to undergoing NIH-standardized animal-model testing, which showed that LEV was not active in the standard models (17). This observation was an indication that LEV has a novel mechanism of action. It also indicates that the NIH-standardized screening may be missing important therapeutic agents and opportunities. Further evidence supporting novel activity lies in the fact that LEV does not produce changes in neuronal excitability by modulation of any of the three main mechanisms underlying classical AED activity (ie, use-dependent blockade of voltage-gated sodium channels, inhibition of low-threshold [T-type] calcium currents, and augmentation of GABAergic inhibitory responses) (18–20).
In vitro testing has shown that LEV does not displace ligands specific for 55 different binding sites, including receptor systems, reuptake sites, second messenger systems, and channel proteins (3). The exact mechanism of antiepileptic action of LEV remains to be elucidated; however, the following preclinical studies indicate that certain atypical cellular effects may be involved in reducing neuronal excitability:
• In isolated CA1 hippocampal neurons, LEV inhibits high-voltage-activated calcium currents (21,22), with selective blockade of N-type calcium channels (22).
• Modest effect in antagonizing α-amino-3-hydroxy-5-methyl-4-isoxazolepropionic acid (AMPA)-mediated currents is better discerned in cultured cortical neurons than in cultured hippocampal neurons (23).
From studies in isolated hippocampal, spinal, and cerebellar neurons, and in sound-susceptible mice, LEV has been shown to suppress negative allosteric modulators (zinc and beta-carbolines) of GABA- and glycine-gated currents (24). Although hyperexcitability may underlie some of the genetic epilepsies that are presently regarded as channelopathies, the acquired epilepsies seem to reveal anatomic and physiologic evidence of seizure-associated circuit rearrangement, which may be, in part, responsible for their intractability. Space in this chapter does not permit extensive discussion of the role of mossy fiber sprouting in the epileptogenic process, but it should be mentioned that mossy fiber sprouting is seen both in experimental models of temporal lobe epilepsy as well as in human hippocampi resected during surgical treatment of mesial temporal lobe epilepsy. Mossy fiber synapses release zinc, and zinc antagonizes GABA-mediated inhibition. The ability of LEV to reverse this interference by zinc of GABAergic inhibition (24) may thus be relevant to its effect on chronic or established epilepsy in animal models.
• One study, in isolated CA1 hippocampal neurons, suggests that LEV may produce a modest reduction in the delayed-rectifier potassium current, thereby lengthening repolarization time (25). A later study, however, detected no effect of LEV on the delayed-rectifier potassium current (26).
• LEV has shown activity in blocking synchronization in a slice experiment in which conventional AEDs such as valproic acid, benzodiazepines, and carbamazepine (CBZ) were inactive (27,28). This is quite interesting because conventional AEDs have been shown to have impact mainly on hyperexcitability, even though the two underpinnings of the epileptic state are hypersynchrony and hyperexcitability. How does LEV accomplish this? Could it be antagonizing currents mediated by gap junctions that are responsible for “local” synchrony? We do not know for certain, but the possibility is intriguing.
• LEV has been shown to bind to a synaptic vesicle–associated protein known as SV2A (29). Mice homozygous for SV2A gene disruption exhibit deficiency in action potential-dependent GABAergic neurotransmission and develop spontaneous seizures (30). It has been proposed that SV2 may enhance neurotransmitter release probability at quiescent synapses by priming vesicles (31). The full details pertaining to the interaction of LEV with vesicle docking and neurotransmitter release remains to be elucidated.
TABLE 58.1
LEVETIRACETAM PHARMACOKINETIC PROFILE | |
Parameter |
Effect |
Absorption |
Rapidly and almost completely (95%) absorbed No saturable absorption No food interaction |
Distribution Tmax Cmax AUC Enzyme kinetics |
1.3 hr 2.3 mcg/mL 222 mcg· hr/mL Linear kinetics; Cmax and AUC are proportional in single doses up to 5,000 mg |
tss Plasma–protein binding Vd Metabolism |
After 48 hr <10% 0.5–0.7 L/kg Minimal; in blood to the inactive deaminated metabolite L057 Not dependent on the hepatic CYP system No autoinduction |
Elimination |
In urine; 66% as parent drug, 24% as L057 |
t1/2 |
Adults 6–8 hr, elderly 10–11 hr, children 6 hr |
AUC, area under the curve; tss, time to plasma steady-state concentration; t1/2, elimination half-life; Vd, volume of distribution.
Source: From Ref. (32). Patsalos PN. Pharmacokinetic profile of levetiracetam: toward ideal characteristics. Pharmacol Ther. 2000;85:77–85. With permission.
BIOTRANSFORMATION, PHARMACOKINETICS, AND INTERACTIONS IN HUMANS
Over 30 clinical studies have evaluated both the single- and multiple-dose pharmacokinetics of LEV following oral administration. Data from these studies have delineated the pharmacokinetic profile of LEV (32,33) (Table 58.1).
Absorption and Distribution
Pharmacokinetic data in adults show that LEV is rapidly and almost completely absorbed after oral administration. Absolute oral bioavailability is nearly 100%, and food does not affect the extent of absorption, but does slow the rate of absorption. Peak plasma levels are generally achieved within 1 hour following oral administration in fasted subjects, and steady-state plasma levels are reached after 2 days of twice-daily dosing. LEV exhibits linear pharmacokinetics at recommended doses (32). LEV is not extensively bound (10%) to plasma proteins, and its volume of distribution is close to that of intracellular and extracellular body water (32).
Metabolism and Elimination
LEV is not extensively metabolized in humans (24% of a dose). The main metabolic pathway involves enzymatic hydrolysis of the acetamide group, is independent of the cytochrome P450 system, and results in the formation of the major metabolite of LEV, which is pharmacologically inactive (32,33). LEV plasma half-life is 7.0 hours and is unaffected by dose or repeated administration. Elimination of both LEV and its major metabolite is via renal excretion, whereby 66% of a dose is excreted as unchanged drug. Total body clearance of LEV is 0.96 mL/min/kg. Renal clearance is 0.6 mL/min/kg and is directly proportional to creatinine clearance (32,33).
In patients with renal impairment, the elimination half-life of LEV is prolonged and total body clearance is decreased 35% to 60%, compared to that in healthy subjects. Thus, daily maintenance doses should be reduced in these patients. LEV is dialyzable; therefore, supplemental doses should be given to patients following dialysis (32,33).
Elderly patients experiencing age-related renal function decline may also require dose reduction of LEV based on their estimated creatinine clearance. Patients with hepatic impairment have no changes in LEV pharmacokinetics, and therefore require no dose reduction (32).
Pediatric Pharmacokinetics
Initial pharmacokinetic data in children came from a multicenter, open-label, single-dose titration study (34). Twenty-four patients (aged 6 to 12 years) received oral LEV as adjunctive therapy at a dose of 20 mg/kg/day. In this pediatric study, LEV clearance was 30% to 40% higher than in adults, resulting in a lower Cmax and area under the curve (AUC) equated for a 1 mg/kg dose. A correspondingly shorter half-life, of 6.01 hours, was observed. Additionally, no significant gender or age differences in pharmacokinetic parameters of LEV were noted. Similar results were found in children 4 weeks to 4 years of age with weight-normalized clearances of approximately 1 to 1.5 mL/min/kg and elimination half-lives of 4 to 6 hours (35). Based on these data, a dose equal to 130% to 140% of the usual daily adult dose in two divided doses, on a weight-normalized level, may be the most appropriate daily maintenance dose in children. Another study, however, found that the apparent clearance of LEV increased less rapidly than body weight, suggesting that the weight-normalized dose should decrease with increases in body weight (36). Chhun and colleagues developed a population pharmacokinetic model to suggest recommended doses of LEV in children, using patients 4 to 16 years of age. Monte Carlo simulations showed that a 10 mg/kg twice-daily dose achieved a similar plasma concentration to the recommended 500 mg twice a day dose in adults, and a 20 mg/kg twice daily regimen would achieve the 6 to 20 mg/L target range for trough concentration in adults (37).
Drug Interactions
Early study of metabolic interactions indicated a low potential for LEV drug interactions (38), and support of this observation has been borne out in additional studies. Pooled data from pivotal Phase III trials confirm that LEV has no known interactions with other AEDs (39). Additionally, LEV does not influence the pharmacokinetics of an oral contraceptive containing ethinyl estradiol and levonorgestrel; on the basis of serum progesterone and luteinizing hormone levels, it does not affect contraceptive efficacy (40). LEV also does not affect the pharmacokinetics of digoxin (41) and warfarin (42).
CLINICAL EFFICACY
Focal Seizures
The efficacy of LEV in adults has been evaluated extensively. Three randomized, double-blind, placebo-controlled clinical trials conducted in the United States and Europe that enrolled 904 patients were pivotal in supporting LEV efficacy as adjunctive therapy in adults with refractory partial-onset seizures with or without secondary generalization (43–45). All three of these studies evaluated LEV using a similar methodology. After an 8-week to 12-week baseline period, patients entered a titration phase where, upon randomization, they had doses titrated upward every 2 weeks over a period of 4 to 6 weeks to a final dose of 1,000, 2,000, or 3,000 mg/day in divided doses, or placebo. Subsequent to titration, a 12-week to 14-week evaluation phase, followed by an optional open-label phase, was completed. A pooled efficacy analysis of these placebo-controlled studies (46) indicates the following. In all three of these studies and in all dose groups, compared to placebo, treatment with LEV resulted in reduced seizure frequency that was statistically significant. Patients receiving LEV achieved a 31% median decrease in seizures from baseline, compared to only a 5.4% median decline in placebo-treated patients (P < .001). Additionally, 35% of LEV-treated patients achieved 50% seizure reduction, compared to 9.4% of placebo-treated patients (P < .001). In these studies, increasing response was associated with increasing dose. Whereas the 50% responder rate in placebo-treated patients was 9.4%, 50% responder rates at doses of 1,000, 2,000, and 3,000 mg/day were 28.6%, 35.2%, and 39.5%, respectively. This same trend was seen in patients who were 100% responders (Figure 58.2).
FIGURE 58.2 Pooled response rates by dose from pivotal Phase III trials.
Source: From Ref. (46). Privitera M. Efficacy of levetiracetam: a review of three pivotal clinical trials. Epilepsia. 2001;42(Suppl 4):31–35. Reprinted with permission from John Wiley & Sons.
Other blinded, placebo-controlled (47), and open- label trials (48–50) support efficacy in partial seizures established by the three pivotal trials. In these trials, conducted using doses from 1,000 to 4,000 mg/day, responder rates in patients with partial-onset seizures ranged from around 40% to 60%. Pooled response rates by dose from pivotal Phase III trials (46) were compared to baseline in nearly 75% of patients. Results of a subset analysis revealed that these findings confirm efficacy results seen in Phase III trials. In the SKATE trial, 178 adult patients were treated for 16 weeks with 1,000, 2,000, or 3,000 mg LEV in an open-label, add-on study of medically refractory patients. The retention rate at 16 weeks was 84.8%. The 50% responder rate was 46.6% with 16.7% of patients achieving seizure freedom (51).
Monotherapy Studies
Initial studies also showed promise for LEV as monotherapy. A responder-selected study of monotherapy was performed in 286 patients. The study began as an add-on study with patients receiving either placebo or 1,500 mg twice daily. Patients who had at least a 50% reduction in seizures entered into a monotherapy phase with a 12-week down-titration of previous medications followed by 12 weeks of monotherapy at 1,500 mg twice daily. In the initial placebo-controlled component of the study, 42.1% of the LEV group achieved a 50% reduction compared to 16.7% of controls. In the LEV monotherapy group, the median percentage reduction was 73.8%. Nine of the original 181 patients randomized to LEV were seizure-free on monotherapy (52). Data from retrospective chart reviews also provide a basis for efficacious monotherapeutic performance. In a review of 45 patients with newly diagnosed partial-onset epilepsy, 5 of 11 patients on LEV therapy for at least 6 months achieved 50% seizure reduction, and 6 became seizure-free (53).
A randomized, placebo-controlled trial (54) comparing LEV (n = 288) and controlled-release carbamazepine (CBZ, n = 291) in new-onset epilepsy produced equivalent seizure-freedom rates using conservative doses (LEV, 500 mg twice daily versus CBZ 200 mg twice daily). One-year remission was achieved by 86% of the patients treated with LEV, while CBZ resulted in remission in 89.3% of patients (54). The European Medicines Agency (EMA) has approved LEV for monotherapy based on such noninferiority trial-based data, while the FDA remains committed to placebo-controlled data to grant such an indication.
Generalized Seizures
LEV first garnered attention for its possible use in generalized epilepsies after a number of uncontrolled or retrospective reports showing positive response. In a small case series of three patients who had failed at least three other AEDs, monotherapy for primary generalized epilepsy (PGE) was assessed (55). All three patients became seizure-free for at least 6 months on LEV therapy. Wever reported on 10 patients with generalized seizures, including six with PGE and four with Lennox–Gastaut syndrome. One became seizure-free, and four more had a greater than 50% reduction in seizures (56). The add-on monotherapy trials in children by Lagae et al (57) confirmed the efficacy of LEV in both partial and generalized epilepsies. A retrospective study of 59 children with intractable epilepsy reported a greater than 50% reduction in 40% of intractable partial seizures, 55% of generalized seizures, and 61% of those with mixed seizures (58).
Berkovic and colleagues (59) assessed the efficacy of LEV in IGE in a multicenter, randomized, double-blind, placebo-controlled, parallel-group study which enrolled patients 4 to 65 years of age with three or more generalized tonic–clonic seizures (GTCs) during an 8-week period while stable on one to two AEDs. Patients were randomized to LEV at a target dose of 3,000 mg/day for adults and 60 mg/kg/day in children versus placebo, with a 4-week titration period followed by a 20-week evaluation period. LEV showed a greater mean reduction in seizure frequency per week (56.5%) over placebo, as well as a higher response in greater than 50% reduction in seizure frequency (72.2%). As a result, LEV obtained FDA approval as an adjunctive therapy for IGE in 2007.
LEV has also been shown to ameliorate interictal spike-wave density in patients with IGE, with two studies showing robust response based on baseline and posttreatment 24-hour electroencephalogram (EEG) (60,61). Gallagher et al (60) reported complete cessation of spike-waves in 9 of 10 patients studied in the awake state and significant reduction during sleep. Rocamora et al (61) reported a 72% reduction in median spike-wave duration in their eight patients. Efficacy of LEV on a variety of epilepsy syndromes involving myoclonus is discussed subsequently under a separate heading.
Pediatric Studies
Following the establishment of its efficacy and safety in adults, LEV adjunctive therapy was evaluated in an open-label trial in children. The 24 pediatric patients enrolled in the open-label, single-dose pharmacokinetic study (34) were also evaluated subsequently for LEV efficacy and safety (62). After a 4-week baseline period, these children (mean age 9.4 years) participated in a 6-week titration phase, followed by an 8-week evaluation phase in which doses ranged from 20 to 40 mg/kg/day. Compared to their baseline seizure frequency, 12 of 23 (52%) patients who entered the evaluation phase achieved 50% seizure reduction, and 5 of 23 (21.7%) achieved 75% seizure reduction. Two patients remained seizure-free throughout the evaluation period. In another open-label study of 39 pediatric patients (mean age 8.6 years) with refractory epilepsy, adjunctive LEV therapy was evaluated during a 9-month treatment period (63). Results of this study support reduced seizure frequency, improved cognition and behavior, and good tolerability.
A double-blind placebo-controlled trial of LEV as adjunctive therapy was performed in 198 children with medically intractable partial seizures. The reduction of LEV-treated patients over placebo treated was significant at 26.8% (P = .0002) with a greater than 50% reduction in 44.6% of the LEV group. Significantly, 6.9% of LEV-treated patients became seizure-free (64). This study led to FDA approval of LEV as adjunctive therapy for children.
Use of LEV in primary generalized seizures as adjunctive therapy has also been supported. An open-label, long-term follow-up study of patients aged 4 to 65 years with primary generalized seizures (tonic–clonic, myoclonic, and absence) treated with LEV at individualized doses (1,000–4,000 mg/day in adults and 20–80 mg/kg/day in children <50 kg) demonstrated seizure freedom in 56.2% of patients for greater than 6 months, with complete seizure freedom in 22.6% for all seizure types (65).
Verrotti et al (66) used LEV in 21 patients with benign rolandic epilepsy (BECTS) and found that all of the patients had at least a 50% reduction in seizures. A longer follow-up study comparing the efficacy of oxcarbazepine (OXC) to LEV in rolandic epilepsy by the same group found 19 of 21 (90.5%) children treated with LEV to be seizure-free, compared to 13 of 18 (72.2%) treated with OXC (67).
Case reports suggest that LEV may be helpful in children with seizures due to tuberous sclerosis complex (68). While one specific case of LEV-responsive Landau–Kleffner syndrome has been reported (69), other reports (70,71) describe the utility of LEV in syndromes of continuous spikes and waves during slow sleep (CSWS). An open-label, prospective trial in pediatric epilepsy syndromes reported two of three patients with CSWS to benefit from LEV therapy (72). A Danish prospective study confirmed a favorable electrographic response in 11 of 20 patients with CSWS treated with LEV (73). There are no convincing data to advocate the use of LEV in catastrophic childhood epilepsies, such as infantile spasms or Lennox–Gastaut syndrome.
There has been emerging evidence for the safety of LEV in the treatment of acute seizures in term and preterm neonates, especially given the breadth of animal data suggestive that LEV does not induce neuronal apoptosis in the developing brain (74). A survey of neonatologists and neurologists by Glass and colleagues in 2011 demonstrated its growing use in the neonatal intensive care unit (NICU), with a small percentage using it as first-line treatment (75). A prospective feasibility study used LEV as first-line treatment intravenously in 38 newborns with EEG-confirmed seizures. Initial doses of 10 mg/kg were given and gradually increased over the following week to 45 to 60 mg/kg. Up to two additional doses of phenobarbital (PHB) 20 mg/kg were allowed to treat refractory seizures. Thirty infants were seizure-free with LEV at the end of the first week and 27 remained seizure-free at 4 weeks, with EEG improvement in 24 patients. Nineteen patients received a single dose of PHB, however, which may have influenced the results. Also, seizures due to hypoxic–ischemic encephalopathy in particular often wane independently by the end of the first week of life, making LEV appear more efficacious if it is the only therapy continued during that time period (76). Further studies are still needed to establish the safety and efficacy of LEV in neonatal seizures.
Myoclonus
Several open-label studies of LEV in myoclonus due to a variety of underlying disorders have been reported. In a study of juvenile myoclonic epilepsy, 38 patients were enrolled and titrated to a dose of 3,000 mg/day over 2 weeks. One-half of the 10 newly diagnosed patients were seizure-free, as were 11 of 38 previously refractory patients (77), indicating that LEV may be a reasonable alternative to the more traditional medications, such as valproic acid. LEV appears to be effective in many patients with Unverricht–Lundborg disease, with 8 of 13 patients demonstrating a measurable improvement. Most responders were the younger patients in the study (78). The report by Labate et al (79) involved patients with both IGE and symptomatic generalized epilepsy who exhibited myoclonus. They reported 50% or greater seizure reduction in 82% of the 35 patients studied. LEV also shows promise in treatment of absence epilepsy with eyelid myoclonia (Jeavons syndrome). An open-label study of 35 patients showed a response rate of 80%, with 6 patients achieving seizure freedom; in addition 15 showed greater than 75% and 7 showed greater than 50% seizure reduction. There was also an improvement in paroxysmal abnormalities with eye closure and photoparoxysmal response (80).
Status Epilepticus
Since LEV became available in IV formulation in 2006, there has been growing interest in its use for status epilepticus. Several retrospective chart reviews showed promise for its use in adults, especially in the elderly and patients with comorbidities, such as hepatic failure and respiratory insufficiency, which would preclude the use of high-dose benzodiazepines and phenytoin (81,82). In a survey of neurocritical care experts in 2013, 6% would use IV LEV as second-line therapy in status epilepticus, 20% as third-line, and 6% as fourth- or fifth-line (83). A small study randomized 44 patients with status epilepticus to treatment with either IV phenytoin (20 mg/kg) or IV LEV (20 mg/kg), with 15 patients achieving clinical termination of seizure activity within 30 minutes after drug infusion with phenytoin (68.2%) versus 13 with LEV (59.1%) (84). Seizure recurrence rates within 24 hours were similar.
Several studies have also shown promise for IV LEV in the treatment of acute seizures and status epilepticus in children. One center performed a retrospective chart review of patients who received a dose of IV LEV for acute seizures, and identified 73 children who received IV LEV within 30 minutes of a seizure. The mean dose administered was 29.4 ± 13.5 mg/kg, with the majority (79%) receiving IV LEV after a series of seizures, 12% for a single seizure, and 8% for status epilepticus. Of the 24 patients receiving only IV LEV for the management of acute seizures, 100% became seizure-free after 1 hour, and 87% to 90% remained seizure-free at 12 to 24 hours (85). A 2-year observational study from the United Kingdom evaluated 45 pediatric patients who presented with acute refractory seizures or status epilepticus. Patients were treated with an initial dose of IV LEV ranging from 5 to 30 mg/kg. Fifty-nine percent of patients with acute seizures became and remained seizure-free; in addition LEV terminated status in three of four patients with convulsive status and two patients with nonconvulsive status epilepticus (86). Further randomized controlled trials are needed to assess the efficacy of IV LEV and appropriate dosing in the treatment of pediatric status epilepticus.
CLINICAL USE IN PEDIATRIC PATIENTS
LEV is an appropriate medication to use in the pediatric age group. It is approved for add-on therapy for partial seizures in children older than 1 month of age and for myoclonus associated with JME in children aged 12 years and older. It has also received approval for use in patients aged 6 years or older for primary generalized tonic–clonic seizures. However, it should be noted that special consideration must be given to use of LEV in children. Based on pharmacokinetic data, LEV clearance is enhanced in children as compared to adults. Thus, in patients under the age of 12, 130% to 140% of the adult daily dose, normalized to body weight, may be appropriate.
The fact that many adult patients will respond to 1,000 mg/day should be taken into account when prescribing for children. It is prudent to start at a low dose, such as 20 mg/kg/day, then titrate upward slowly, as this may diminish the propensity for adverse effects, to a maximum of 60 mg/kg/day. LEV is currently available as tablets and as a liquid preparation, so it can be readily administered to children of all ages and children with special needs, such as those fed by gastrostomy tube.
Long-Term Studies
Long-term clinical usefulness of LEV has been evaluated. In one study, data from all 1,422 epilepsy patients exposed to LEV during any phase of development were analyzed (87,88). The median duration of treatment was 399 days (range 1–8 years), and the median reduction from baseline in seizure frequency over the entire treatment period was 39.6%. Furthermore, no decrease in efficacy was observed over time in each cohort exposed to LEV ranging from 6 to 54 months.
Other studies looking at refractory epilepsy patients followed for 1 year support findings of sustained efficacy. In one open-label study of 98 patients, 57 achieved 50% seizure reduction and 14 were seizure-free for the first year. This study particularly attempted to investigate types of patients who would not be eligible for clinical trials because of factors such as intellectual disability and comorbid neuropsychiatric disorders (89). Another study reported a 26% seizure-free rate at 1 year, and this benefit correlated with the fact that 77% of patients continued on LEV therapy at 1 year (90).
Quality of Life Studies
The effect of LEV on quality of life has been investigated. Using the 31-item Quality of Life in Epilepsy (QOLIE-31) questionnaire, patients randomized to LEV at 1,000 mg/day or to placebo were studied over an 18-week period. Significant improvements in several areas, including seizure worry, overall quality of life, cognitive functioning, and total score, were seen in patients taking LEV compared to those taking placebo (91). A subsequent evaluation of a subset of these patients who were followed for 4 years indicates that improvements in QOLIE-31 scores remained stable during the 4-year follow-up period (92).
ADVERSE EFFECTS IN ADULTS
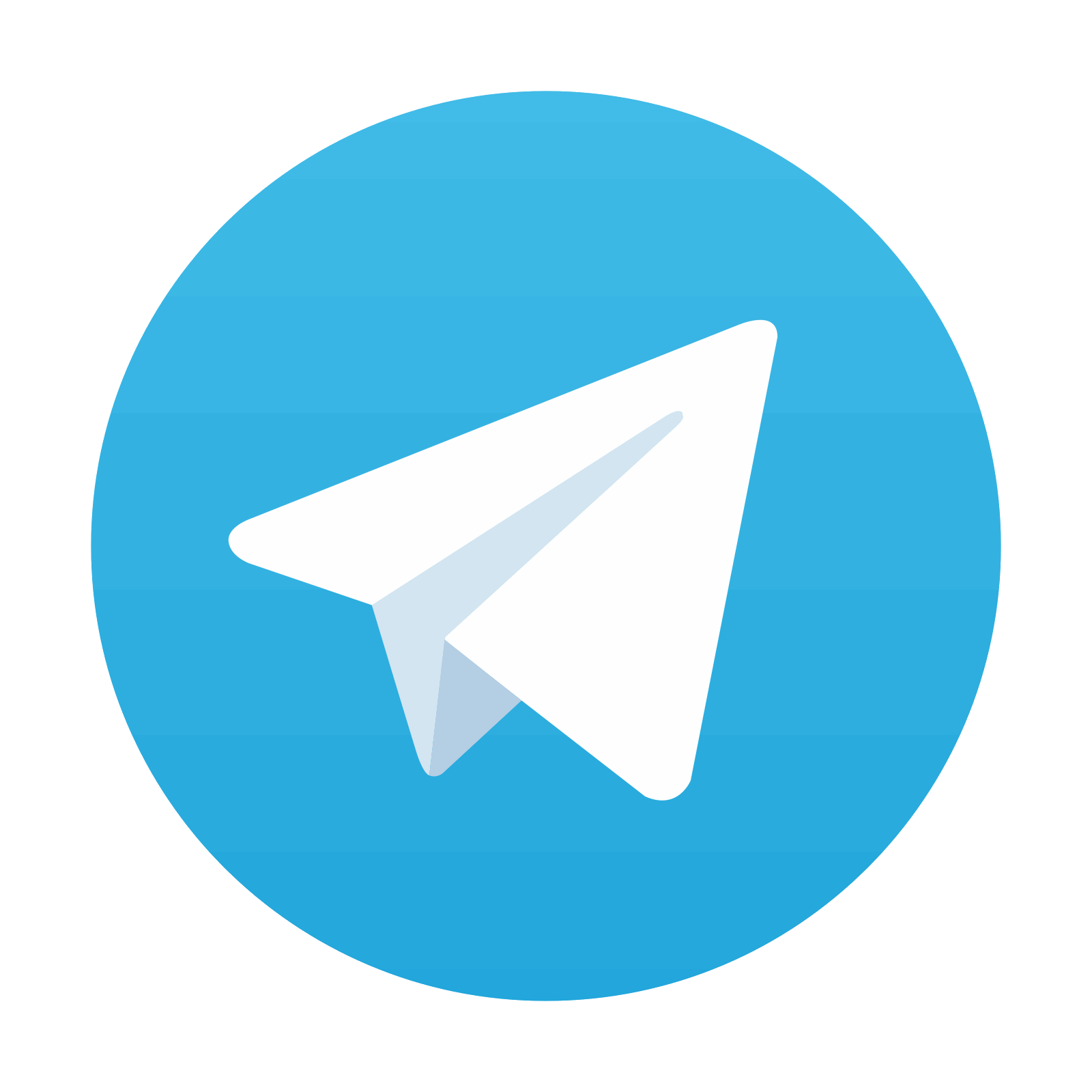
Stay updated, free articles. Join our Telegram channel

Full access? Get Clinical Tree
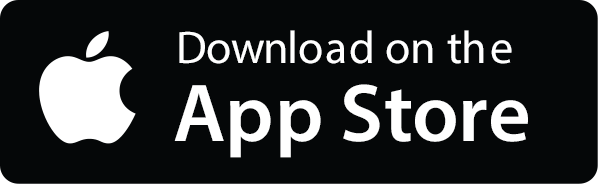
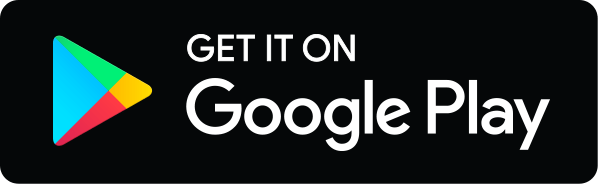