Outline
Amniotic Fluid Physiology and Dynamics, 704
Determination of Amniotic Fluid Volume, 706
Potential Difficulties in Amniotic Fluid Volume Estimation Using Ultrasound, 707
Normal Amniotic Fluid Volume in Singleton Pregnancies, 708
Amniotic Fluid Volume and Pregnancy Outcomes, 710
Assessment of Amniotic Fluid Volume in the High-Risk Patient, 712
Polyhydramnios, 712
Fetal Growth Restriction and Amniotic Fluid Volume, 714
Amniotic Fluid Volume in Twin Pregnancies, 714
Conclusions, 715
Summary of Key Points
- •
The pathways influencing amniotic fluid volume (AFV) are dynamic and complex and are not completely understood.
- •
The balance of maintaining the overall AFV occurs at three key areas: fetal urine production and fetal swallowing, the intramembranous pathway, and the osmotic fluid gradient created between the mother and the fetus.
- •
There are several published curves that attempt to define a normal AFV.
- •
Abnormal fluid volumes should be defined as specific high or low values across gestation that are linked with adverse pregnancy outcomes.
- •
The single deepest pocket measurement for estimation of the AFV is the preferred technique for assessment during the ultrasound evaluation.
Amniotic fluid provides an ideal setting for the developing fetus by providing an environment that protects from trauma, provides a source of water, allows for normal fetal movements essential for anatomic development, and contributes to the development of the fetal lungs. The amniotic fluid volume (AFV) can reflect fetal well-being, and changes in the volume may indicate maternal or fetal disease processes. Therefore, the assessment of the AFV is an essential component to the sonographic evaluation of the gravid patient.
Amniotic Fluid Physiology and Dynamics
Once thought to be a static collection of fluid with turnover once per day, the AFV is now recognized to be a complex system involving multiple dynamic pathways that influence the inflow and outflow of fluid and solutes within the amniotic space. The physiologic processes that influence the AFV are complex and not yet completely understood. In order to appreciate the AFV, knowledge of the pathways for potential amniotic fluid movement and the regulatory mechanisms involved need to be considered.
Several potential variables influence the overall AFV. Most influential are fetal urine production and fetal swallowing. Others include secretion of fetal lung fluid, the intramembranous pathway (movement of water and solutes between amniotic fluid and fetal blood and the placenta), the transmembranous pathway (movement of water and solutes across the surface area of the amnion and chorion), secretions by the fetal oronasal cavities, and movement of water across the highly permeable fetal skin during early gestation. Amniotic fluid consists primarily of water (98-99%). In early pregnancy, amniotic fluid is isotonic with maternal or fetal plasma and contains a small amount of proteins. Although amniotic fluid is present in early gestation prior to fetal urine production, not much is known regarding the dynamics of amniotic fluid early in human pregnancy. However, there is likely an active transport of solutes across the amnion into the amniotic space with water moving passively down an osmotic gradient. A transudate of plasma may also occur across nonkeratinized fetal skin or from the mother across the uterine decidua and the placental surface, which may contribute to the AFV in early pregnancy ( Fig. 20-1 ).

Much more is known about the dynamics of the AFV in the latter half of pregnancy. Fetal skin keratinizes at approximately 22 to 25 weeks’ gestation, preventing further movement of water across the fetal skin. As a result of an increasing production of dilute fetal urine, amniotic fluid osmolality and sodium concentrations decrease as the gestational age increases. Ultimately amniotic fluid osmolality reaches 250 to 260 mOsm/mL at term. Fetal osmolality (≈278 mOsm/mL) remains close to maternal osmolality (280 mOsm/mL), thereby limiting the amount of water transfer between the fetal and maternal circulations under normal conditions.
Fetal urine production is the primary source of amniotic fluid in the second half of pregnancy. This is supported by the near complete absence of amniotic fluid in the setting of renal agenesis or fetal urinary tract obstruction. Fetal urine first enters the amniotic space at 8 to 11 weeks’ gestation and continually increases throughout gestation. Fetal urine production per kilogram of fetal body weight at 25 weeks’ gestation is approximately 110 mL/kg per 24 hours, and it increases to approximately 190 mL/kg per 24 hours at 39 weeks’ gestation. Based on sonographic studies of the fetal bladder measured at regular intervals, the human fetal urine output at term is estimated to be 700 to 900 mL/day. In order to contribute to the regulation of the AFV, the fetus is able to respond to changes in fluid status by adjusting urine flow. Thurlow and Brace observed fetal ovine hypoxia to be associated with increased urine flow, as opposed to the often associated chronic fetal hypoxia and low AFV. Similarly, Gagnon and associates found the reduction in overall AFV in the setting of chronic severe placental insufficiency in sheep was due to an increase in the intramembranous absorption of amniotic fluid, not a decrease in fetal urinary production. This finding suggests that human regulation of the AFV is mediated by other mechanisms (e.g., intramembranous absorption) in addition to changes in fetal urine production at times of fetal hypoxia.
Fetal swallowing plays a key role in maintaining a stable AFV. In humans, the fetus begins to swallow around the same time that fetal urine first enters the amniotic space, at 8 to 11 weeks’ gestational age. An estimated 210 to 760 mL/day is swallowed by the near term fetus. In the ovine fetus, hypoxia has been shown to suppress fetal swallowing, whereas decreases in amniotic fluid osmolality and increases in fetal plasma osmolality will increase fetal swallowing. In primates, esophageal ligation leads to the development of hydramnios; however, the AFV returns to normal before delivery. It is thought the maintenance of a constant AFV despite esophageal ligation in the presence of continued urine production is a result of increasing intramembranous absorption. Based on these studies, it seems the human fetus is able to alter its swallowing to some degree under various conditions; however, it is unlikely that this represents the major regulatory mechanism by which the human fetus maintains its AFV.
Secretion of fetal lung fluid into the amniotic cavity is well established. This concept is supported by the fact that phospholipids are present in the amniotic fluid that can be used as tests for fetal lung maturity. These phospholipids are of pulmonary origin and are not present in substantial quantities in the fetal urine. Ligation of the trachea in utero in other animal species results in expansion of the fetal lung seemingly due to the continued production of fetal lung fluid. Fetal lung fluid secretion allows for pulmonary expansion serving to promote fetal lung development. Near term fetal sheep demonstrate an outflow of lung fluid of 200 to 400 mL/day. Approximately half of the secreted lung fluid enters the amniotic fluid, and the remainder is swallowed as it exits the trachea, resulting in a net secretion of 100 to 200 mL/day. Secretion flow is likely the result of active transport of chloride ions across the epithelial lining of the developing lungs. The fetal glottis serves to prevent backflow of amniotic fluid into the trachea; thus, there appears to be little osmotic exchange between amniotic fluid and fetal plasma in the lung. Secretion of lung fluid into the amniotic fluid is likely a main contributor to the AFV, although factors that regulate fetal lung fluid secretion are poorly understood, and the exact amount secreted into the amniotic cavity in the human fetus has not yet been quantified.
An excess of approximately 400 mL is present within the amniotic cavity after considering the volume of fetal urine production and secreted lung fluid less the amount removed via fetal swallowing. Furthermore, as gestation advances, fetal urine production as well as secreted lung fluid increases. The process by which this excess volume is compensated to maintain AFV balance is referred to as the intramembranous pathway. Based on studies in the near term ovine fetus, it is estimated that approximately 200 to 500 mL/day of amniotic fluid is absorbed via the intramembranous pathway. Studies in other animal species have demonstrated that the AFV returns to a homeostatic state even after large volumes of fluid are infused into the amniotic fluid cavity or after esophageal ligation. This finding suggests there is a continuous flow of water and solutes from the amniotic fluid into the fetal circulation. The osmotic gradient between the fetal circulation and the amniotic fluid appears to influence the intramembranous movement of water and solutes across the fetal vessels on the surface of the placenta into the fetal circulation. However, in one ovine study, it was demonstrated that only approximately 35% of the intramembranous movement of fluid depends on the osmotic difference between the amniotic fluid and the fetal circulation. Consequently, there must be other nonpassive mechanisms that contribute to this pathway.
The maternal fluid status can influence the fluid balance between the fetal circulation and the AFV. At times of maternal dehydration, the intramembranous pathway likely plays a role in correcting the fetal volume status. With maternal dehydration, the maternal serum osmolality increases, resulting in water movement from the fetal circulation to the maternal circulation. This results in a fetal dehydration state causing increased fetal osmolality and promoting movement of water from the amniotic fluid back into the fetal circulation in order to restore the fetal intravascular volume, leading to a decrease in the AFV. The reverse is also true. As demonstrated by Magann and colleagues, intravenous maternal hydration with 1 L of fluid increased both the actual and sonographically estimated AFV in the human fetus, with an average increase in the actual AFV of 188 mL. Similarly, Kilpatrick and coworkers showed that in a patient with a low AFV, maternal hydration with 2 L of water can increase the human fetal amniotic fluid index (AFI) by up to 31%. There is little evidence to support the movement of fluid via the transmembranous pathway as a major contributor to the overall AFV. Studies in sheep suggest that only 10 mL/day is absorbed by the uterus in late gestation in the setting of normal osmolality. Given the large surface area of the amnion and chorion, it is clear why membrane permeability has been proposed as a regulator of the AFV. However, very little is known regarding the permeability and filtration characteristics of the membranes as they relate to amniotic fluid dynamics.
The amount of fluid secreted by the fetal oronasal cavities is small and not considered a main contributor to the AFV. Studies in sheep demonstrate that approximately 25 mL/day is secreted by the fetal oronasal cavities in late gestation. Based on information regarding transepidermal water loss in the preterm infant, it is generally believed there is movement of water across the fetal skin before 22 to 25 weeks’ gestation, which contributes to the AFV in the first half of pregnancy. It seems reasonable to assume there may be a relationship between fetal weight and the AFV because a larger fetus would presumably produce more urine output. However, neonatal birth weight has not been shown to be correlated with a either a dye-determined or an ultrasound-estimated AFV. In addition, no clinical correlation has been shown between the AFI and estimated human fetal weight ( Fig. 20-2 ).

Determination of Amniotic Fluid Volume
How does one measure the AFV during pregnancy? The true AFV can be measured by dye-dilution techniques utilizing amniocentesis or can be directly measured at the time of cesarean delivery. However, these methods are obviously not practical for routine clinical use. Alternatively, the AFV is commonly estimated with the use of ultrasound techniques. In the literature, four techniques have been described to estimate the AFV: the AFI ; the single deepest pocket (SDP), also referred to as the deepest vertical pocket (DVP) or maximal vertical pocket (MVP) ; the two-diameter pocket ; and subjective assessment. The two methods most commonly used are the AFI and DVP. The two-diameter pocket measurement (horizontal and vertical dimensions of the maximum vertical pocket are multiplied together to obtain a single value, in cm 2 ) was shown to not be a better predictor of AFV than the AFI and is no longer widely used. As originally described by Phelan and associates, in order to calculate the AFI, the abdomen is divided into four quadrants in which the umbilicus divides the upper and lower halves and the linea nigra divides the right and left halves ( Fig. 20-3 ). With the patient supine, the linear (or curvilinear) transducer is placed along the maternal anterior abdominal wall and held perpendicular to the floor. The maximum vertical pocket of fluid (usually reported in centimeters) is measured in each of the four quadrants, and the AFI (in centimeters) equals the sum of these four measurements. The DVP is simply the largest single vertical pocket of fluid that is at least 1 cm in width, obtained following the same criteria described here ( Fig. 20-4 ).


Studies have demonstrated that the dye-determined amniotic fluid calculation and direct measurement at the time of cesarean delivery have good concordance ( r = 0.99) and either can be used to determine actual AFV. It has been shown that both the AFI and the DVP technique can reliably identify normal AFVs. Unfortunately, most studies have demonstrated poor reliability for ultrasound-estimated AFVs to predict actual low or high volumes. Subjective assessment (visual interpretation without ultrasound measurements) of AFV has been compared with dye-determined or directly measured AFV, and studies have shown no difference in the accuracy of subjective compared to objective assessment (visual interpretation with ultrasound measurements) in the correct determination of AFV. Likewise, when comparing subjective assessment of AFV in the second and third trimesters and objective assessment with dye-determined fluid volumes, neither operator experience nor sonographic technique was found to affect the accuracy of ultrasound estimates of the AFV ( Figs. 20-5 and 20-6 ).


Traditionally, pockets filled with umbilical cord have not been used in measurement of the AFV. The use of color Doppler ultrasound can help detect umbilical cord, which may be present within an apparent pocket of amniotic fluid. Thus, it has been suggested that color Doppler be applied during evaluation of the AFV to identify segments of umbilical cord that may not be appreciated on standard gray-scale ultrasound images ( Fig. 20-7 ) in order to improve detection of low AFV. However, the use of color Doppler in the sonographic estimation of AFV has been shown to result in overdiagnosis of low AFV (oligohydramnios). In a study by Magann and colleagues, the use of color Doppler inappropriately diagnosed 21% of women with low AFV who actually had normal dye-determined AFVs. In addition, color Doppler did not identify any more pregnancies with true dye-determined low AFVs compared with traditional gray-scale ultrasound imaging.

Potential Difficulties in Amniotic Fluid Volume Estimation Using Ultrasound
Determining an estimated AFV either objectively or subjectively is generally straightforward. However, a few potential pitfalls are worth mentioning. Maternal positioning, whether supine or at a 45-degree semierect position, does not appear to influence the measurement of the AFV. However, as demonstrated by Fok and coworkers, fetal positioning can influence the calculation of the AFI, although it does not appear to influence the DVP measurement ( Fig. 20-8 ). Fetal movements do not appear to alter the assessment of AFV, nor does the choice of the transducer, whether curvilinear or sector.

Excessive pressure with the transducer while scanning can negatively influence the estimation of AFV. Flack and associates demonstrated that excess pressure on the maternal abdomen resulted in a 21% decrease in calculated AFI measurement. It is important to remember that maternal adipose tissue tends to scatter the ultrasound beam, which may result in artificial echoes suggested within pockets of amniotic fluid. Thus, obese women may appear to have reduced volume of amniotic fluid as a result of these artifactual echoes ( Fig. 20-9 ). It may help to use a lower frequency transducer when assessing the AFV in these patients. Also, measurements of amniotic fluid pockets may be made in varying locations by different examiners; this too can lead to variation in estimates of AFV ( Fig. 20-10 ).


Normal Amniotic Fluid Volume in Singleton Pregnancies
In order to establish what constitutes an abnormal AFV, methods for assessing normal amniotic fluid for singleton pregnancies must be determined. Based on published studies in the literature, there are multiple accepted definitions of an abnormally low or high AFV. An abnormally low AFV (referred to as oligohydramnios ) has been defined as any one of the following: a total volume less than 200 mL or less than 500 mL, a value below the 5th percentile for gestational age, an SDP less than 2 cm, an AFI less than 5 cm, or a subjectively low AFV. An increased AFV (referred to as polyhydramnios ) can be defined as any one of the following: a total volume greater than 2000 mL, a value above the 95th or 97th percentiles for gestational age, an SDP greater than or equal to 8 cm, an AFI greater than or equal to 24 cm or greater than 25 cm, or a subjectively increased AFV.
Several studies have attempted to define normal AFVs across gestation. The AFV deemed normal is to a certain degree dependent upon the gestational age at which it was measured. There are multiple published reference curves demonstrating changes in AFV across gestation and a few notable studies evaluating either dye-determined or directly measured AFVs. Queenan and colleagues, in 1972, used dye-determined methods to assess the AFVs of 187 patients across gestation from 15 to 16 weeks to 41 to 42 weeks. They demonstrated an increase in AFV as pregnancy advanced from the 15th to the 20th week, and then AFV remained relatively constant from the 20th through the 41st week. Their study showed that the AFV peaked at 33 to 34 weeks’ gestation, then gradually decreased until term, decreasing at a greater rate after 41 weeks. Brace and Wolf in 1989 derived normal values from a compilation of 705 pregnancies from 12 previously published studies with gestational ages ranging from 8 to 43.2 weeks. They reported a peak AFV of 931 mL at 33.8 weeks with a decrease in the AFV thereafter ( Fig. 20-11 ). Using polynomial regression and log transformation of the data, the authors found no statistically significant change in the AFV from 22 to 39 weeks’ gestation, with an average volume of 777 mL and a range of 630 to 817 mL. They showed a decrease in the AFV after 40 weeks by 8% per week. Magann and coworkers, in 1997, measured the AFVs of 144 singleton pregnancies between 15 and 40 weeks also using a single dye-dilution technique with analysis performed in the same laboratory; the AFV was found to increase across gestation with a peak at 40 weeks. In 2014, Sandlin and associates modeled AFVs across gestation in normal singleton pregnancies using the statistical method quantile regression (QR). In their study, 379 women with singleton pregnancies were evaluated and their amniotic volumes were calculated by either dye-dilution technique or direct measurement at the time of cesarean delivery between 16 and 41 weeks’ gestation. These authors were then able to derive a normative chart for AFVs across each week of gestation (16-41 weeks) at the 5th, 25th, 50th, 75th, and 95th percentiles ( Table 20-1 ). The authors point out in their study that the use of quantile regression enabled them to relax the stringent assumptions of standard regression models and overcome several of those limitations, thereby allowing them to more appropriately estimate values at the extremes of a distribution (e.g., below the 5th and above the 95th percentiles). Because those values are labeled as abnormal, this is where clinical interest and concern lie—the relationship of abnormal amniotic fluid values and their subsequent association with perinatal outcome.

Weeks of Gestation | AMNIOTIC FLUID VOLUME PERCENTILE | ||||
---|---|---|---|---|---|
5th | 25th | 50th | 75th | 95th | |
16 | 134.0 | 334.5 | 377.1 | 503.2 | 694.7 |
17 | 132.3 | 322.0 | 389.6 | 552.2 | 937.2 |
18 | 130.9 | 311.1 | 401.9 | 602.0 | 1233.7 |
19 | 129.9 | 301.7 | 414.0 | 652.1 | 1584.8 |
20 | 129.2 | 293.7 | 425.8 | 701.8 | 1986.6 |
21 | 128.9 | 286.9 | 437.2 | 750.4 | 2430.0 |
22 | 128.9 | 281.4 | 448.3 | 797.2 | 2900.5 |
23 | 129.2 | 277.0 | 459.0 | 841.5 | 3378.4 |
24 | 129.8 | 273.7 | 469.2 | 882.5 | 3839.9 |
25 | 130.8 | 271.4 | 478.9 | 919.5 | 4258.8 |
26 | 132.1 | 270.2 | 488.1 | 951.9 | 4609.3 |
27 | 133.8 | 270.0 | 496.7 | 979.1 | 4868.0 |
28 | 135.8 | 270.8 | 504.7 | 1000.5 | 5016.9 |
29 | 138.3 | 272.6 | 512.1 | 1015.9 | 5045.3 |
30 | 141.1 | 275.4 | 518.8 | 1024.8 | 4951.1 |
31 | 144.4 | 279.3 | 524.8 | 1027.1 | 4741.3 |
32 | 148.1 | 284.4 | 530.0 | 1022.8 | 4430.5 |
33 | 152.3 | 290.6 | 534.5 | 1012.0 | 4040.0 |
34 | 157.0 | 298.0 | 538.2 | 994.8 | 3594.8 |
35 | 162.3 | 306.8 | 541.1 | 971.6 | 3121.4 |
36 | 168.2 | 317.0 | 543.2 | 942.8 | 2644.7 |
37 | 174.7 | 328.8 | 544.5 | 909.0 | 2186.7 |
38 | 182.0 | 342.3 | 545.0 | 870.7 | 1764.2 |
39 | 190.0 | 357.7 | 544.7 | 828.7 | 1389.0 |
40 | 198.2 | 375.2 | 543.5 | 783.6 | 1067.1 |
41 | 207.9 | 395.0 | 541.5 | 736.2 | 800.0 |
Given that the actual AFV can only be directly calculated by amniocentesis with dye-dilution techniques or directly measured at the time of cesarean delivery, in clinical practice, the AFV is estimated by ultrasound evaluation using the AFI, the DVP, or subjective assessment. An often-cited reference for normal ultrasound-estimated AFV is an article by Moore and Cayle, published in 1990, in which the authors attempted to define the normal AFI in pregnancy. They evaluated the AFI, as described by Rutherford and colleagues, in 791 patients with normal pregnancies between 16 and 44 weeks. Using polynomial regression and logarithmic transformation, they found the mean AFI curve to rise beginning at 16 weeks, peak at 27 weeks, plateau until 33 weeks, and then decline until 42 weeks. They observed that the AFI decreased by 12% per week after 40 weeks ( Table 20-2 ). In 2000, Magann and coworkers performed a prospective study to determine normal values for sonographically measured AFI, SDP, and two-diameter pocket in normal human pregnancy. They recruited 50 patients at every gestational age between 14 and 41 weeks, for a total of 1400 patients. The data were analyzed using linear regression and logarithmic transformation, and they found that when AFI is used to define fluid status across gestation, it increases from 14 to 31 weeks and then declines thereafter; when SDP or two-diameter pocket is used, the AFV increases from 14 to 20 weeks’ gestation, plateaus between 20 and 37 weeks, and then gradually declines thereafter through the 41st week. If one were to consider the individual cases in the study by Magann and coworkers but use the curve reported by Moore and Cayle, one would find that 36% of the patients in the study by Magann and coworkers who had normal outcomes would have been labeled as having abnormally low or high AFVs. In 2007, Machado and associates evaluated the AFIs of 2868 pregnant women. Using multiple linear regression and quadratic polynomial adjustments, they found the AFIs across gestation to be essentially constant between 20 and 33 weeks’ gestation, decreasing after 33 weeks, with the most significant decrease after the 38th week of gestation. Lei and Wen constructed an AFI curve by gestational week based on data from 5496 Chinese women. The 5th, 50th, and 95th percentiles of this study were different from the curves of both Moore and Cayle and Magann and coworkers across gestation. The curves of both Lei and Wen and Magann and coworkers reported AFIs less at each gestational age than in the curve of Moore and Cayle.
Weeks of Gestation | AMNIOTIC FLUID INDEX PERCENTILE | n | ||||
---|---|---|---|---|---|---|
2.5th | 5th | 50th | 95th | 97.5th | ||
16 | 73 | 79 | 121 | 185 | 201 | 32 |
17 | 77 | 83 | 127 | 194 | 211 | 26 |
18 | 80 | 87 | 133 | 202 | 220 | 17 |
19 | 83 | 90 | 137 | 207 | 225 | 14 |
20 | 86 | 93 | 141 | 212 | 230 | 25 |
21 | 88 | 95 | 143 | 214 | 233 | 14 |
22 | 89 | 97 | 145 | 216 | 235 | 14 |
23 | 90 | 98 | 146 | 218 | 237 | 14 |
24 | 90 | 98 | 147 | 219 | 238 | 23 |
25 | 89 | 97 | 147 | 221 | 240 | 12 |
26 | 89 | 97 | 147 | 223 | 242 | 11 |
27 | 85 | 95 | 146 | 226 | 245 | 17 |
28 | 86 | 94 | 146 | 228 | 249 | 25 |
29 | 84 | 92 | 145 | 231 | 254 | 12 |
30 | 82 | 90 | 145 | 234 | 258 | 17 |
31 | 79 | 88 | 144 | 238 | 263 | 26 |
32 | 77 | 86 | 144 | 242 | 269 | 25 |
33 | 74 | 83 | 143 | 245 | 274 | 30 |
34 | 72 | 81 | 142 | 248 | 278 | 31 |
35 | 70 | 79 | 140 | 249 | 279 | 27 |
36 | 68 | 77 | 138 | 249 | 279 | 39 |
37 | 66 | 75 | 135 | 244 | 275 | 36 |
38 | 65 | 73 | 132 | 239 | 269 | 27 |
39 | 64 | 72 | 127 | 226 | 255 | 12 |
40 | 63 | 71 | 123 | 214 | 240 | 64 |
41 | 63 | 70 | 116 | 194 | 216 | 162 |
42 | 63 | 69 | 110 | 175 | 192 | 30 |
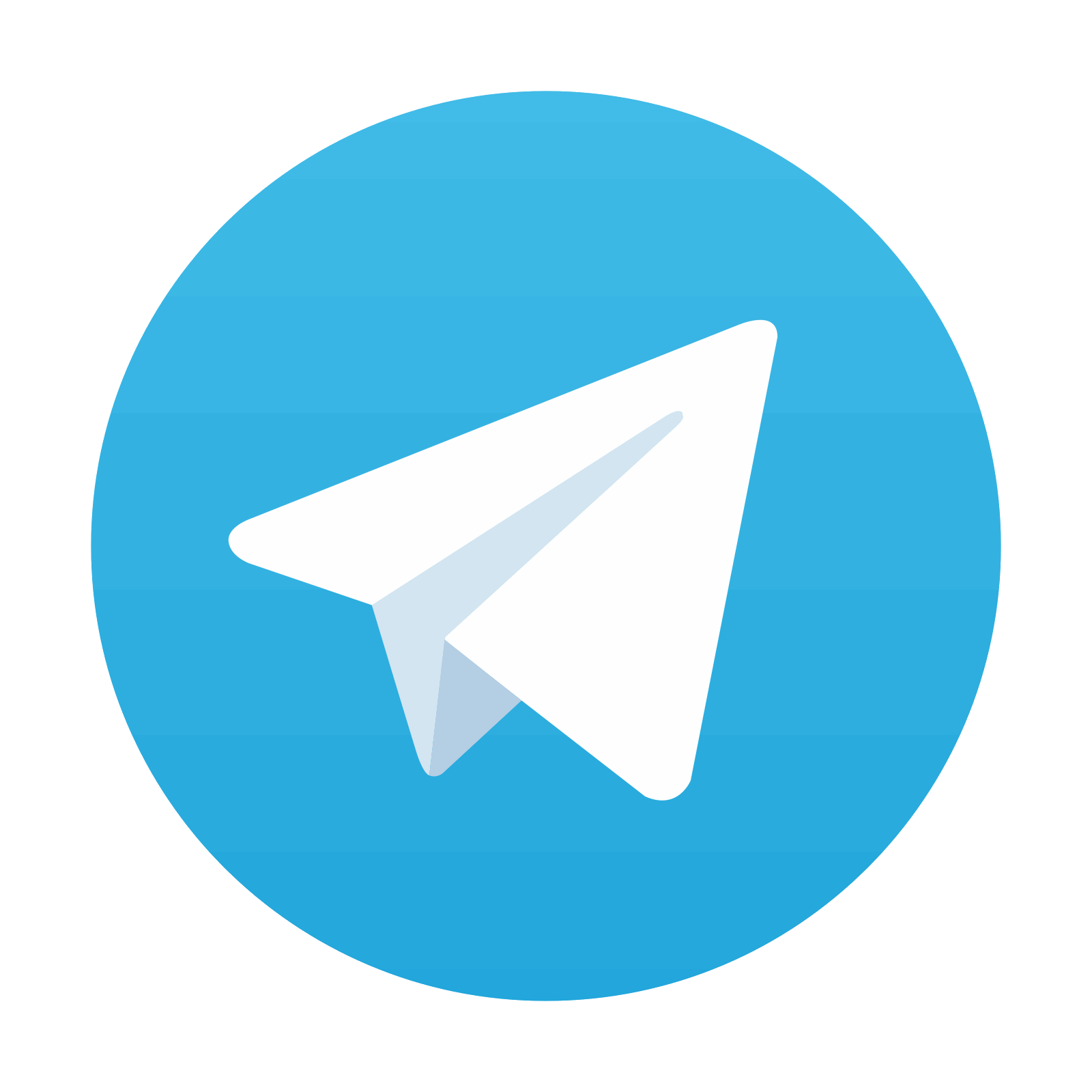
Stay updated, free articles. Join our Telegram channel

Full access? Get Clinical Tree
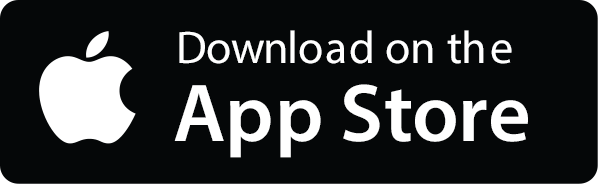
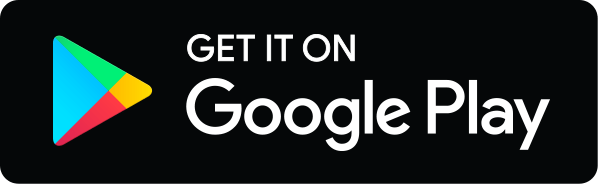
