The surgical armamentarium of the pediatric urologist has changed greatly in the past 2 decades on account of new technology and careful adaptation of minimally invasive techniques in children. Conventional laparoscopy, robotic-assisted laparoscopy, laparoendoscopic single-site surgery, and endourologic surgery have, to varying degrees, provided new approaches to urologic surgery in the pediatric population. This article reviews the technology and adaptations behind these recent advances as well as their current applications in management of urologic disease in children.
- •
Due to recent technological advancements and careful adaptations in the pediatric patient, minimally invasive approaches now have routine applications in pediatric urology.
- •
Robotic surgery has the potential to make some minimally invasive techniques more accessible to pediatric urologists by simplifying complex reconstructive procedures.
- •
Miniaturization of endoscopic equipment has expanded the indications of endourologic surgery in the pediatric stone patient.
- •
Challenges for the foreseeable future include cost-containment and further improvement in patient comfort and cosmesis.
Introduction
The last 2 decades have seen great changes in the surgical management of urologic disease in children. Following the emergence of minimally invasive approaches in the adult population, urologists have successfully applied these techniques in pediatric patients. The most notable advancements have occurred in laparoscopy, robotics, and endourology. Improvements in imagery and miniaturization of laparoscopic equipment have expanded the indications of laparoscopy in children from simple orchiopexy and pyeloplasty to ureteral reimplantation. Even more complex urologic surgery such as bladder reconstruction is being performed more readily with the aid of robotics. The treatment of stone disease in children has also changed for the better because of the availability of smaller and more flexible endourologic equipment.
The technical difficulty and steep learning curve of conventional laparoscopy led to the application of robotic assistance, namely with the da Vinci Surgical System (Intuitive Surgical, Sunnyvale, CA, USA). Robotic assistance has the potential to make laparoscopic surgery more accessible to pediatric urologists by simplifying complex reconstructive procedures. Although still in its infancy, laparoendoscopic single-site surgery (LESS) is making the laparoscopic approach even less invasive.
Adaptations of laparoscopy for the pediatric patient
The foundation of laparoscopy in urology has its origins in the use of the cystoscope to inspect the peritoneal cavity of children with nonpalpable undescended testes. Despite this early use in the pediatric population, most of the equipment was developed for adults. Specific challenges of laparoscopy in children include a smaller working space, limited area for trocar insertion, delicacy of tissues, and manual dexterity required when working with smaller instruments and suture. This article first discusses recent technological advancements and considerations when applying them in children.
Insufflation
Abdominal insufflation is accomplished with carbon dioxide (CO 2 ) gas, which is noncombustible and highly soluble. Automated insufflation machines allow manual settings to regulate intra-abdominal pressure and CO 2 flow rates. Children are particularly sensitive to increases in intra-abdominal pressure, with potentially severe respiratory and hemodynamic changes, with greater risk of subcutaneous emphysema from dissection of insufflation gas. Subcutaneous emphysema is disturbing on examination, but not a clinical problem provided it is not associated with pneumothorax. Peritoneal insufflation has cardiovascular, pulmonary, vascular, neurologic, and somatic effects. A common complaint during recovery is shoulder pain, a referred pain from diaphragmatic distension. Hypercapnia from CO 2 insufflation causes hypertension, tachycardia, and increased cardiac output; however, the reduced venous return from compression of the inferior vena cava during pneumoperitoneum often negates this sympathetic effect. Ventilatory difficulty should prompt reduced abdominal insufflation, return to supine position, and a check of the endotracheal tube, which can migrate during surgery. Perhaps the most feared, but very rare, complication of laparoscopy is venous gas embolism; a mill-wheel cardiac murmur is diagnostic but rarely encountered. Gas embolism is immediately treated with desufflation of the abdomen; positioning in a left lateral, decubitus, head-down position (which moves the gas bubble to the apex of the right ventricle); 100% oxygen supplementation; and hyperventilation to help reabsorb the carbon dioxide gas bubble.
The current norms used for working pressure in infants (0–2 years), children (2–10 years), and adolescents (>10 years) are 8 to 10 mm Hg, 10 to 12 mm Hg, and 15 mm Hg, respectively. Increasing the pressure beyond these levels does little to increase the working space in pediatric patients. Reduced abdominal wall laxity may require higher intra-abdominal insufflation pressures but directly increases risk of cardiopulmonary, vascular, or neurohumoral complications.
Trocars
Trocars enable the laparoscopic surgeon to introduce working instruments into the insufflated abdomen and provide a conduit for the carbon dioxide gas to maintain pneumoperitoneum. The typical trocar consists of an outer sheath (or port) that contains an inner obturator, which is removed following trocar insertion. In both infants and children, it is preferable to limit the trocar size because of the shorter body wall and limited working space. The authors have had success with a latex-free, radially dilated trocar system (Step system, Covidien, Mansfield, MA, USA) that incorporates an expandable sheath that accommodates 3-mm, 5-mm, and 10-mm ports. An advantage to the Step system is elimination of the need for fascial closure after removal of the port. Fascia is closed to prevent bowel or omental herniation through the abdominal wall; however, in practice, a child’s abdominal wall is so thin that fascia is easy to close regardless of trocar size. All nonradially dilating trocar sizes 10 mm and greater deserve proper fascial closure. Direct intra-abdominal visualization of port sites on trocar removal helps identify injury to abdominal wall vessels, typically the inferior epigastric artery or its superficial branches.
Laparoscopes and Camera Systems
Laparoscopes are typically rigid, contain a 0° or 30° lens, and range in size from 5 mm to 10 mm. Most pediatric urologic procedures can be completed with the use of a 30° lens, which allows the surgeon to see behind intra-abdominal structures. Aside from the camera, patient positioning is critical to successful laparoscopy. Renal surgery is performed in a lateral or flank position, allowing bowel to fall away. Bladder and ureteral surgery are often performed in dorsal lithotomy; Trendelenburg positioning lessens the need for bowel retraction. Diagnostic laparoscopy and orchiopexy are straightforward with children positioned supine. Careful padding of pressure points, securing of the endotracheal tube, and taping the patient to the bed are important, because patients can slide during frequent bed rotation or tilting during surgery ( Fig. 1 ).

Optics are not uniform between laparoscopes and the quality of visualization is directly proportional to scope diameter. For example, a 3-mm telescope has fused bundle fiberoptics that produce pixilated images with reduced resolution. Many 3-mm and current 5-mm and 10-mm telescopes contain rod lens systems that provide excellent quality and brightness. The authors think that 5-mm optics offer acceptable light transmission and visualization in almost all pediatric applications.
The quality of the laparoscopic camera systems have markedly improved with time. Digital equipment, as opposed to analog, offers high-fidelity imagery and picture recording, with better color, sharpness, contrast, and field depth. High-definition video towers with widescreen displays improve visualization. Two-dimensional (2D) images lack spatial depth information and require the laparoscopist to recognize this limitation and compensate. This disadvantage has led to recent advances in 3-dimensional (3D) video technology, which uses 2 optic channels in a single metallic sheath to mimic the binocular function of a surgeon’s eyes. Markedly improved images, digital input, and video recording capability have allowed surgeons to perfect and teach minimally invasive techniques, which is an asset not widely available in open surgery.
Laparoscopic Instruments
Although instrumentation for open surgery has seen little change in the last several decades, laparoscopic instrumentation is constantly adapting to meet the demands of expanded applications of minimally invasive surgery. The use of laparoscopy in pediatric urology has required miniaturization of standard adult laparoscopic instruments. The decreased diameter of these instruments affects their rigidity. Although 5-mm and 10-mm instruments are effectively rigid rods, 3-mm instruments flex to varying degrees based on manufacturer, length, and instrument type. However, 3-mm instruments are sufficiently rigid to prove immediately functional to most surgeons. One compromise in the use of smaller instruments is that the 3-mm arsenal is more limited than the 5-mm arsenal.
Manual dexterity and finesse are required when working with laparoscopic needle drivers, especially in the infant or child. A fine needle driver (ie, 3 mm or 5 mm) is required when working with the fine 5-0 or 6-0 suture that is commonly used for laparoscopic pyeloplasty in children. Needles can get caught within the sheath of a 5-mm trocar, and, at that small size, are not easily visualized on radiograph. Therefore, carefully watching needles as they enter the abdomen or placing them through a larger 8-mm to 10-mm trocar minimizes this risk. A lost needle means removal of the trocar to cut open its sheath, and, if still not found, then open surgical conversion. The EndoStitch suturing device (Covidien, Mansfield, MA, USA) is a disposable, 1-handed, 10-mm suturing instrument that shuttles its needle from one jaw through tissue, then to the other jaw; Although it makes tissue closure easier, its application in children is limited because of large instrument size.
Electrosurgical Instruments
Electrosurgical devices combine laparoscopic instruments with an energy source, providing a means to cauterize vessels during dissection. Monopolar energy can be added to most laparoscopic dissectors and scissors and is useful in the cauterization of small vessels. The monopolar hook electrode is particularly useful for dissection around tubular structures. Monopolar cautery must be used with great caution because of the pattern of current spread across tissue as well as the risk of current arcing through a gap in the instrument’s insulation. These risks are increased in the limited working space of pediatric laparoscopy. Monopolar coagulation runs the highest risk of electrosurgical injury outside the surgeon’s visual field via the mechanisms of insulation failure, direct coupling (conduction of current from direct touching of an energized instrument and other metal device), and capacitative coupling (induction of current in nearby conductors despite normal insulation).
Bipolar electrocautery involves flow of radiofrequency-based current from one jaw to the other, which reduces thermal spread and capacitive coupling. Simple bipolar instruments do not cut tissue and are generally powered by the standard electrosurgical unit (Bovie). One example of a more advanced so-called smart bipolar device is the LigaSure vessel sealing system (Valleylab, Boulder, CO, USA), which generates more current with only a percentage of the voltage required by a simple bipolar instrument. The LigaSure actively monitors changes in tissue impedance, provides real-time adjustment of the energy output, and can coagulate vessels of up to 7 mm. A 5-mm diameter device is available for pediatric applications.
The Harmonic scalpel (Ethicon Endosurgery, Cincinnati, OH, USA) is a disposable ultrasonic cutting and coagulating surgical device. The handpiece of the device transforms electrical energy into mechanical energy by the use of a piezoelectric crystal system. The rapid mechanical vibrations significantly increase the temperature of the active blade, denaturing proteins by breaking hydrogen bonds and creating a coagulum that seals vessels of up to 5 mm. The intracorporeal portion of the instrument dissipates heat (and steam), so care must be taken to avoid inadvertent thermal injury to adjacent structures. In comparison, the Harmonic scalpel coagulates and cuts tissues at lower temperatures (50–150°C) than its radiofrequency-based counterparts (150–400°C) and may therefore be safer in tight spaces or near important structures.
da Vinci Surgical System
The da Vinci Surgical System consists of a surgeon console, patient-side cart with interactive robotic arms, and a vision cart. It was approved for clinical use by the United States Food and Drug Administration in 2000. Although the system allows for the use of 4 working arms, the fourth arm is seldom used in the pediatric population because of the limited working space. The camera arm contains 2 optic channels in a single metallic sheath, which mimics the surgeon’s binocular vision, and transmits a high-definition 3D image at the surgeon console ( Fig. 2 ). Although smaller cameras are available in 8.5 mm (3D) and 5 mm (2D), the best image is achieved through the 12-mm camera, giving the surgeon a 10× magnified, 3D picture. The surgeon sits comfortably at the surgeon console, from where the robotic controls including arm position, focus, zoom, camera position, and instrument movement can be manipulated. Among the principal advantages for the use of robotic-assisted surgery are tremor filtration and motion scaling. Tremor filtration results in steadier and smoother movements even with longer laparoscopic instruments that would otherwise magnify unintentional movement. Computerized motion scaling allows large movements by the surgeon to be translated into delicate manipulations in the surgical field.
Robotic Instrumentation
The robotic instruments have proprietary EndoWrist technology (Intuitive Surgical, Sunnyvale, CA, USA) that allows the surgeon to move the instrument with the 7 degrees of freedom present in the human wrist. The instrument’s movement is more similar to the surgeon’s hand and wrist motion than conventional laparoscopy in which the surgeon’s movements are opposite to those of the internal instruments.
Most EndoWrist instruments, which require special 8-mm robotic trocars, were created for use in adults. Five-millimeter instruments have been created for use in children and infants and rely on a 3-joint wrist. However, the arsenal of 5-mm robotic instruments is limited. Notably absent are the Hot Shears (Intuitive Surgical, Sunnyvale, CA, USA), curved scissors that are electrocautery capable and one of the more common instruments used in robotic surgery; for these reasons, most surgeons still use 8-mm instruments in pediatric urologic surgery. Bipolar electrocautery can be used through 8-mm Maryland bipolar forceps, which function as a dissector, grasper, and retractor. PK Dissecting Forceps (Intuitive Surgical, Sunnyvale, CA, USA) require a proprietary generator that provides audio feedback that signifies adequate coagulation of tissue. Although robotic instrumentation is still limited, all 5-mm laparoscopic instruments, including the suction/irrigator, can be used through a separate port or 8-mm robotic port by a skilled bedside assistant.
Adaptations of laparoscopy for the pediatric patient
The foundation of laparoscopy in urology has its origins in the use of the cystoscope to inspect the peritoneal cavity of children with nonpalpable undescended testes. Despite this early use in the pediatric population, most of the equipment was developed for adults. Specific challenges of laparoscopy in children include a smaller working space, limited area for trocar insertion, delicacy of tissues, and manual dexterity required when working with smaller instruments and suture. This article first discusses recent technological advancements and considerations when applying them in children.
Insufflation
Abdominal insufflation is accomplished with carbon dioxide (CO 2 ) gas, which is noncombustible and highly soluble. Automated insufflation machines allow manual settings to regulate intra-abdominal pressure and CO 2 flow rates. Children are particularly sensitive to increases in intra-abdominal pressure, with potentially severe respiratory and hemodynamic changes, with greater risk of subcutaneous emphysema from dissection of insufflation gas. Subcutaneous emphysema is disturbing on examination, but not a clinical problem provided it is not associated with pneumothorax. Peritoneal insufflation has cardiovascular, pulmonary, vascular, neurologic, and somatic effects. A common complaint during recovery is shoulder pain, a referred pain from diaphragmatic distension. Hypercapnia from CO 2 insufflation causes hypertension, tachycardia, and increased cardiac output; however, the reduced venous return from compression of the inferior vena cava during pneumoperitoneum often negates this sympathetic effect. Ventilatory difficulty should prompt reduced abdominal insufflation, return to supine position, and a check of the endotracheal tube, which can migrate during surgery. Perhaps the most feared, but very rare, complication of laparoscopy is venous gas embolism; a mill-wheel cardiac murmur is diagnostic but rarely encountered. Gas embolism is immediately treated with desufflation of the abdomen; positioning in a left lateral, decubitus, head-down position (which moves the gas bubble to the apex of the right ventricle); 100% oxygen supplementation; and hyperventilation to help reabsorb the carbon dioxide gas bubble.
The current norms used for working pressure in infants (0–2 years), children (2–10 years), and adolescents (>10 years) are 8 to 10 mm Hg, 10 to 12 mm Hg, and 15 mm Hg, respectively. Increasing the pressure beyond these levels does little to increase the working space in pediatric patients. Reduced abdominal wall laxity may require higher intra-abdominal insufflation pressures but directly increases risk of cardiopulmonary, vascular, or neurohumoral complications.
Trocars
Trocars enable the laparoscopic surgeon to introduce working instruments into the insufflated abdomen and provide a conduit for the carbon dioxide gas to maintain pneumoperitoneum. The typical trocar consists of an outer sheath (or port) that contains an inner obturator, which is removed following trocar insertion. In both infants and children, it is preferable to limit the trocar size because of the shorter body wall and limited working space. The authors have had success with a latex-free, radially dilated trocar system (Step system, Covidien, Mansfield, MA, USA) that incorporates an expandable sheath that accommodates 3-mm, 5-mm, and 10-mm ports. An advantage to the Step system is elimination of the need for fascial closure after removal of the port. Fascia is closed to prevent bowel or omental herniation through the abdominal wall; however, in practice, a child’s abdominal wall is so thin that fascia is easy to close regardless of trocar size. All nonradially dilating trocar sizes 10 mm and greater deserve proper fascial closure. Direct intra-abdominal visualization of port sites on trocar removal helps identify injury to abdominal wall vessels, typically the inferior epigastric artery or its superficial branches.
Laparoscopes and Camera Systems
Laparoscopes are typically rigid, contain a 0° or 30° lens, and range in size from 5 mm to 10 mm. Most pediatric urologic procedures can be completed with the use of a 30° lens, which allows the surgeon to see behind intra-abdominal structures. Aside from the camera, patient positioning is critical to successful laparoscopy. Renal surgery is performed in a lateral or flank position, allowing bowel to fall away. Bladder and ureteral surgery are often performed in dorsal lithotomy; Trendelenburg positioning lessens the need for bowel retraction. Diagnostic laparoscopy and orchiopexy are straightforward with children positioned supine. Careful padding of pressure points, securing of the endotracheal tube, and taping the patient to the bed are important, because patients can slide during frequent bed rotation or tilting during surgery ( Fig. 1 ).
Optics are not uniform between laparoscopes and the quality of visualization is directly proportional to scope diameter. For example, a 3-mm telescope has fused bundle fiberoptics that produce pixilated images with reduced resolution. Many 3-mm and current 5-mm and 10-mm telescopes contain rod lens systems that provide excellent quality and brightness. The authors think that 5-mm optics offer acceptable light transmission and visualization in almost all pediatric applications.
The quality of the laparoscopic camera systems have markedly improved with time. Digital equipment, as opposed to analog, offers high-fidelity imagery and picture recording, with better color, sharpness, contrast, and field depth. High-definition video towers with widescreen displays improve visualization. Two-dimensional (2D) images lack spatial depth information and require the laparoscopist to recognize this limitation and compensate. This disadvantage has led to recent advances in 3-dimensional (3D) video technology, which uses 2 optic channels in a single metallic sheath to mimic the binocular function of a surgeon’s eyes. Markedly improved images, digital input, and video recording capability have allowed surgeons to perfect and teach minimally invasive techniques, which is an asset not widely available in open surgery.
Laparoscopic Instruments
Although instrumentation for open surgery has seen little change in the last several decades, laparoscopic instrumentation is constantly adapting to meet the demands of expanded applications of minimally invasive surgery. The use of laparoscopy in pediatric urology has required miniaturization of standard adult laparoscopic instruments. The decreased diameter of these instruments affects their rigidity. Although 5-mm and 10-mm instruments are effectively rigid rods, 3-mm instruments flex to varying degrees based on manufacturer, length, and instrument type. However, 3-mm instruments are sufficiently rigid to prove immediately functional to most surgeons. One compromise in the use of smaller instruments is that the 3-mm arsenal is more limited than the 5-mm arsenal.
Manual dexterity and finesse are required when working with laparoscopic needle drivers, especially in the infant or child. A fine needle driver (ie, 3 mm or 5 mm) is required when working with the fine 5-0 or 6-0 suture that is commonly used for laparoscopic pyeloplasty in children. Needles can get caught within the sheath of a 5-mm trocar, and, at that small size, are not easily visualized on radiograph. Therefore, carefully watching needles as they enter the abdomen or placing them through a larger 8-mm to 10-mm trocar minimizes this risk. A lost needle means removal of the trocar to cut open its sheath, and, if still not found, then open surgical conversion. The EndoStitch suturing device (Covidien, Mansfield, MA, USA) is a disposable, 1-handed, 10-mm suturing instrument that shuttles its needle from one jaw through tissue, then to the other jaw; Although it makes tissue closure easier, its application in children is limited because of large instrument size.
Electrosurgical Instruments
Electrosurgical devices combine laparoscopic instruments with an energy source, providing a means to cauterize vessels during dissection. Monopolar energy can be added to most laparoscopic dissectors and scissors and is useful in the cauterization of small vessels. The monopolar hook electrode is particularly useful for dissection around tubular structures. Monopolar cautery must be used with great caution because of the pattern of current spread across tissue as well as the risk of current arcing through a gap in the instrument’s insulation. These risks are increased in the limited working space of pediatric laparoscopy. Monopolar coagulation runs the highest risk of electrosurgical injury outside the surgeon’s visual field via the mechanisms of insulation failure, direct coupling (conduction of current from direct touching of an energized instrument and other metal device), and capacitative coupling (induction of current in nearby conductors despite normal insulation).
Bipolar electrocautery involves flow of radiofrequency-based current from one jaw to the other, which reduces thermal spread and capacitive coupling. Simple bipolar instruments do not cut tissue and are generally powered by the standard electrosurgical unit (Bovie). One example of a more advanced so-called smart bipolar device is the LigaSure vessel sealing system (Valleylab, Boulder, CO, USA), which generates more current with only a percentage of the voltage required by a simple bipolar instrument. The LigaSure actively monitors changes in tissue impedance, provides real-time adjustment of the energy output, and can coagulate vessels of up to 7 mm. A 5-mm diameter device is available for pediatric applications.
The Harmonic scalpel (Ethicon Endosurgery, Cincinnati, OH, USA) is a disposable ultrasonic cutting and coagulating surgical device. The handpiece of the device transforms electrical energy into mechanical energy by the use of a piezoelectric crystal system. The rapid mechanical vibrations significantly increase the temperature of the active blade, denaturing proteins by breaking hydrogen bonds and creating a coagulum that seals vessels of up to 5 mm. The intracorporeal portion of the instrument dissipates heat (and steam), so care must be taken to avoid inadvertent thermal injury to adjacent structures. In comparison, the Harmonic scalpel coagulates and cuts tissues at lower temperatures (50–150°C) than its radiofrequency-based counterparts (150–400°C) and may therefore be safer in tight spaces or near important structures.
da Vinci Surgical System
The da Vinci Surgical System consists of a surgeon console, patient-side cart with interactive robotic arms, and a vision cart. It was approved for clinical use by the United States Food and Drug Administration in 2000. Although the system allows for the use of 4 working arms, the fourth arm is seldom used in the pediatric population because of the limited working space. The camera arm contains 2 optic channels in a single metallic sheath, which mimics the surgeon’s binocular vision, and transmits a high-definition 3D image at the surgeon console ( Fig. 2 ). Although smaller cameras are available in 8.5 mm (3D) and 5 mm (2D), the best image is achieved through the 12-mm camera, giving the surgeon a 10× magnified, 3D picture. The surgeon sits comfortably at the surgeon console, from where the robotic controls including arm position, focus, zoom, camera position, and instrument movement can be manipulated. Among the principal advantages for the use of robotic-assisted surgery are tremor filtration and motion scaling. Tremor filtration results in steadier and smoother movements even with longer laparoscopic instruments that would otherwise magnify unintentional movement. Computerized motion scaling allows large movements by the surgeon to be translated into delicate manipulations in the surgical field.

Full access? Get Clinical Tree
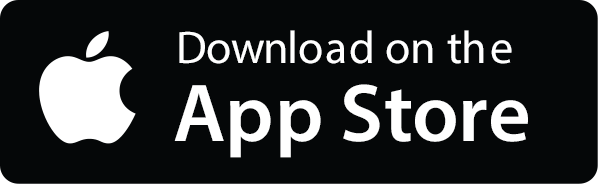
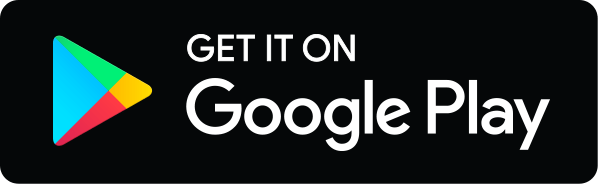