Advances in early recognition, effective response, and high-quality resuscitation before, during, and after cardiac arrest have resulted in improved survival for infants and children over the past 10 years. This review addresses several key factors that can make a difference in survival outcomes, including the etiology of pediatric cardiac arrests in and out of hospital, mechanisms and techniques of circulation of blood flow during cardiopulmonary resuscitation (CPR), quality of CPR, meticulous postresuscitative care, and effective training. Monitoring and quality improvement of each element in the system of resuscitation care are increasingly recognized as key factors in saving lives.
Key points
- •
Advances in early recognition, effective response, and high-quality resuscitation before, during, and after cardiac arrest have resulted in improved survival for infants and children over the past 10 years.
- •
Several key factors can make a difference in survival outcomes, including the etiology of pediatric cardiac arrests in and out of hospital, mechanisms and techniques of circulation of blood flow during cardiopulmonary resuscitation (CPR), quality of CPR, meticulous postresuscitative care, and emphasis on effective training.
- •
Early warning scores with implementation of the rapid response team, and training strategies that include simulation and debriefing of events, have been shown to improve implementation of guidelines and protocols that improve outcome.
- •
Monitoring and quality improvement of each element in the system of resuscitation care are increasingly recognized as key factors in saving lives.
- •
By strategically focusing therapies on specific phases of cardiac arrest and resuscitation and on evolving pathophysiology, there is great promise that critical care interventions will lead the way to more successful cardiopulmonary and cerebral resuscitation in children.
Introduction
Advances in resuscitation science and the science of education and implementation have substantially improved survival outcomes for cardiac arrest over the past 10 years, particularly for pediatric in-hospital cardiac arrest. Cardiac arrests occur in 0.7% to 3% of pediatric hospital admissions and 1.8% to 5.5% of pediatric intensive care unit (PICU) admissions. Survival rates following in-hospital cardiac arrests have improved from 9% in 1987 to 16% to 30% over the past 10 years. Select inpatients, such as children who undergo cardiac surgery, are at greater risk for cardiac arrest but also have improved potential for good survival outcome. Unfortunately, good neurologic outcome and quality of life is not universal, especially following pediatric out-of-hospital cardiac arrest. This review addresses key factors that affect outcome: early recognition of warning signs before cardiac arrest, rapid response and initiation of resuscitation, adjusting the resuscitation interventions to the cause of the cardiac arrest, mechanisms of circulation of blood flow during cardiopulmonary resuscitation (CPR), focus on the quality of CPR, meticulous postresuscitative care, and emphasis on effective training techniques that can save lives and improve the quality of life for survivors.
Introduction
Advances in resuscitation science and the science of education and implementation have substantially improved survival outcomes for cardiac arrest over the past 10 years, particularly for pediatric in-hospital cardiac arrest. Cardiac arrests occur in 0.7% to 3% of pediatric hospital admissions and 1.8% to 5.5% of pediatric intensive care unit (PICU) admissions. Survival rates following in-hospital cardiac arrests have improved from 9% in 1987 to 16% to 30% over the past 10 years. Select inpatients, such as children who undergo cardiac surgery, are at greater risk for cardiac arrest but also have improved potential for good survival outcome. Unfortunately, good neurologic outcome and quality of life is not universal, especially following pediatric out-of-hospital cardiac arrest. This review addresses key factors that affect outcome: early recognition of warning signs before cardiac arrest, rapid response and initiation of resuscitation, adjusting the resuscitation interventions to the cause of the cardiac arrest, mechanisms of circulation of blood flow during cardiopulmonary resuscitation (CPR), focus on the quality of CPR, meticulous postresuscitative care, and emphasis on effective training techniques that can save lives and improve the quality of life for survivors.
Early recognition and rapid response to warning signs before cardiac arrest
More than 4000 children in the United States receive in-hospital CPR each year, mostly in PICUs. Many of these events are a result of progressive respiratory failure and circulatory shock. There are at least 4 phases of cardiac arrest: (1) prearrest, (2) no flow (untreated cardiac arrest), (3) low flow (CPR), and (4) postresuscitation. The prearrest phase represents the greatest opportunity to affect patient survival by preventing pulseless cardiopulmonary arrest. Preventing common childhood accidents by using car restraint devices and pool safety can decrease the incidence of cardiac arrest. Patients often have abnormal physiologic parameters associated with acute respiratory insufficiency and circulatory shock in the hours before their event. In hospital, early warning and rapid response systems have affected early recognition, treatment, and transfer to PICUs. Several nonrandomized adult studies have shown improvement in outcomes with the presence of rapid response teams, although a cluster randomized trial in adults did not show a decrease in cardiac arrests or mortality. Prospective randomized trials confirming that rapid response teams truly improve patient outcome are lacking. However, several pediatric studies suggest that rapid response systems decrease the incidence of cardiac arrests in comparison with retrospective control periods. Reports from the American Heart Association’s Get With The Guidelines—Resuscitation national database show that 93% of all inpatient CPR events from 2000 to 2010 in reporting hospitals occurred in the PICU setting. The ratio of ICU-to-ward CPR has increased substantially over the past 10 years, with 9% to 13% occurring on wards from 2000 to 2003 and 4% to 6% occurring on wards from 2004 to 2010. The rates of successful initial resuscitation from these PICU and ward CPR events (ie, return of spontaneous circulation [ROSC]) increased in 2004 to 2010 compared with 2000 to 2003, concurrent with the increase in ratio of PICU-to-ward CPR events.
Rapid response systems can identify and transfer critically ill children to the PICU for intensive monitoring and aggressive interventions that might prevent cardiac arrest or provide prompt advanced life support by experienced providers. Many organizations, including the Institute for Healthcare Improvement ( www.ihi.org ) and the Child Healthcare Corporation of America ( www.chca.com ), consider pediatric CPR events on general wards as “never” events.
Outcomes and etiology of pediatric cardiac arrest
In most reports, 60% to 80% of in-hospital pediatric cardiac arrest patients achieve ROSC, approximately 20% to 38% survive to hospital discharge, and approximately 15% to 25% have a good neurologic outcome. Outcomes following pediatric out-of-hospital cardiac arrests are much worse, typically with approximately 5% to 10% survival to hospital discharge and approximately 2% to 5% with good neurologic outcome. Many out-of-hospital cardiac arrests are not witnessed, and only 30% of children are provided with bystander CPR. In all cardiac arrests, delayed CPR initiation, unwitnessed arrests, prolonged periods of “no flow,” and first documented electrocardiograph rhythm of asystole are associated with poor outcome.
Most pediatric cardiac arrests, whether in or out of hospital, are the result of respiratory failure leading to shock, bradycardia progressing to pulseless electrical activity, and loss of circulation. Interventions to improve outcome from pediatric cardiac arrest should ideally be targeted to address the presumed cause, duration, and “phase” of resuscitation. For cardiac arrests caused by asphyxia and/or ischemia, provision of adequate myocardial perfusion and myocardial oxygen delivery with ventilation titrated to blood flow is important. For ventricular fibrillation (VF) and pulseless ventricular tachycardia, rapid identification of shockable rhythms and prompt defibrillation are critical. In animal models of sudden VF cardiac arrest, acceptable partial pressures of arterial oxygen (Pa o 2 ) and carbon dioxide (Pa co 2 ) persist for 4 to 8 minutes during chest compressions without rescue breathing, because there is no blood flow and aortic oxygen consumption is minimal. The lungs serve as a reservoir for oxygen during the low-flow state of CPR. By contrast, animal studies of asphyxia-precipitated cardiac arrests have established that rescue breathing is a critical component of successful CPR. During asphyxia, blood continues to flow to tissues; therefore, arterial and venous oxygen saturations decrease while carbon dioxide and lactate increase. In addition, continued pulmonary blood flow before the cardiac arrest depletes the pulmonary oxygen reservoir. Therefore, asphyxia results in significant arterial hypoxemia and acidemia before resuscitation, in contrast to VF. In this circumstance, rescue breathing can be life-saving.
Interventions during the no-flow phase of pulseless cardiac arrest focus on early recognition of cardiac arrest and prompt initiation of CPR, restoring blood flow to the brain and heart while activating emergency response and accessing equipment. Effective CPR initiates a low-flow phase, with an effort to optimize coronary and cerebral perfusion, while matching blood flow with oxygenation and ventilation needs. Basic life support with near continuous effective chest compressions (eg, push hard, push fast, allow full chest recoil, minimize interruptions, and do not overventilate) is recommended.
Mechanisms of blood flow during CPR
During CPR, cardiac and pulmonary blood flow is approximately 10% to 25% of that during normal sinus rhythm. The spontaneously beating heart receives the majority of its perfusion during diastole, with coronary artery blood flow linearly dependent on aortic diastolic pressure. During chest compressions, aortic pressure rises concurrently with right atrial pressure. During chest decompression, the right atrial pressure falls faster and lower than the aortic pressure, generating a pressure gradient that perfuses the heart. Thus coronary perfusion occurs during diastole or the “release” of chest compression. Coronary perfusion pressure that is lower than 15 mm Hg during CPR is associated with lack of ROSC, and perfusion pressure higher than 30 mm Hg during CPR is associated with successful return of spontaneous circulation.
To allow good venous return in the decompression phase of chest compressions, it is important to allow full chest recoil and to avoid overventilation. Negative intrathoracic pressure assists venous return to the heart during CPR, and improves blood flow. During the decompression phase, negative intrathoracic pressure can be enhanced by briefly impeding air flow to the lungs (eg, with an inspiratory impedance threshold device). Negative intrathoracic pressure promotes venous return, cardiac output, and mean aortic pressure. This approach improves organ perfusion pressures and myocardial blood flow in humans and animals. Despite success in animal studies and human hemodynamics, adult human studies have showed mixed results on survival outcomes, and specific studies in children are lacking. Further evidence that manipulating intrathoracic pressure during CPR can improve hemodynamics is suggested by studies of active compression-decompression devices. To date, no studies of mechanical or automated CPR have been shown to be superior to high-quality manual chest compressions in children.
Animal and adult data indicate that overventilation during CPR is common, and can substantially compromise venous return and cardiac output. Most concerning, these adverse hemodynamic effects during CPR, combined with the interruptions in chest compressions to provide airway management and rescue breathing, can contribute to worse survival outcomes.
Open-Chest CPR
Excellent open-chest CPR with direct cardiac compression can generate a cerebral blood flow that approaches normal. Although open-chest massage improves coronary perfusion pressure and increases the chance of successful defibrillation in animals and humans, surgical thoracotomy is impractical in many situations. Open-chest CPR may warrant consideration in selected special resuscitation circumstances, such as after sternotomy following cardiac surgery.
Ratio of Compressions to Ventilation
Ideal compression-ventilation ratios for pediatric patients are unknown. Physiologic models estimate that the amount of ventilation needed during CPR is much less than the amount needed during a normal perfusing rhythm, because the cardiac output during CPR is only 25% to 40% of that during normal sinus rhythm. The best ratio of compressions to ventilations depends on many factors including the compression rate, the tidal volume, the blood flow generated by compressions, and the time that compressions are interrupted to perform ventilations. A chest compression to ventilation ratio of 15:2 delivers the same minute ventilation as CPR with a chest compression to ventilation ratio of 5:1 in a mannequin model of pediatric CPR, but the number of chest compressions delivered was 48% higher with the 15:2 ratio. When chest compressions cease, the aortic pressure rapidly falls, thus dropping coronary perfusion pressure. Increasing the ratio of compressions to ventilations minimizes these interruptions, thus increasing coronary blood flow.
Medications for Cardiac Arrest
Although epinephrine and vasopressors consistently improve hemodynamics and initial ROSC following both asphyxial and VF cardiac arrests, no single medication has been shown to improve survival outcome after pediatric cardiac arrest. Medications commonly used for CPR in children are vasopressors (eg, epinephrine), antiarrhythmics (eg, amiodarone or lidocaine), sodium bicarbonate, and calcium chloride. Routine use of bicarbonate and calcium is not recommended, but may be beneficial for specific resuscitation circumstances (eg, severe acidosis, postbypass, hyperkalemia). Nonselective administration of these medications is associated with worse survival outcomes.
Extracorporeal Membrane Oxygenation–CPR
Extracorporeal membrane oxygenation (ECMO)–CPR can establish circulation and provide controlled reperfusion following cardiac arrest. Prospective, controlled outcome studies are lacking. Case series and registry reports suggest excellent outcomes when ECMO rescue therapy is applied to selected patients, with potentially reversible acute postoperative myocardial dysfunction or arrhythmias. It is important to emphasize that the children who survived with ECMO-CPR despite prolonged CPR had brief periods of no flow, excellent CPR during the low-flow period, and a well-controlled postresuscitation phase.
Quality of CPR
Despite evidence-based guidelines, extensive provider training, and provider credentialing in resuscitation courses, the quality of CPR is typically poor because of slow compression rates, inadequate depth of compression, frequent leaning on the chest with incomplete chest recoil, and substantial pauses in chest compressions. CPR guidelines recommend target values for key CPR parameters related to rate and depth of chest compressions and ventilations, avoidance of no-flow intervals (interruptions in chest compression), and complete release of sternal pressure between compressions. An approach that achieves “push hard, push fast, minimize interruptions, allow full chest recoil, and do not overventilate” can result in excellent myocardial, cerebral, and systemic perfusion, and will likely improve outcomes.
During the past decade, innovative technologies have extended the ability to monitor and provide real-time feedback on CPR quality during training on mannequins, and to apply this to use in actual cardiac arrests. Feedback on chest compression rate, depth, ventilation rate, no-flow interval (pauses), and incomplete chest wall recoil (leaning) can be tracked and fed back in real time. In the setting of real in-hospital pediatric cardiac arrest, several studies have confirmed the positive effect of feedback in improving CPR quality, compliance with recommended rates for chest compression depth and rate, and reduced leaning. Quality of postresuscitative management has also been demonstrated to be critically important in improving resuscitation survival outcomes.
Meticulous care after cardiac arrest
The goal of postresuscitation care is to diagnose and treat the underlying cause of the arrest, minimize secondary brain injury, and support end-organ perfusion and function. The postresuscitation phase is a high-risk period for reperfusion injuries, brain injury, and ventricular arrhythmias. Myocardial dysfunction and severe hypotension are common during the postresuscitation phase, particularly in the first 6 hours after restoration of spontaneous circulation. The postarrest phase may have the most potential for innovative advances in the understanding of cell injury and death, inflammation, and apoptosis, ultimately leading to novel interventions. Specific attention should be paid to oxygenation, ventilation, temperature control, seizure control, glucose and electrolytes, and hemodynamics, with the goal of matching appropriate substrate delivery to critical end-organ tissue demands.
Oxygenation
Following ROSC, both persistent hypoxemia (low Pa o 2 ) and hyperoxemia (elevated Pa o 2 ) have been associated with poor neurologic outcomes, with concerns that hyperoxia may worsen oxidative injury during reperfusion. Data in children are less clear, with some studies showing association with poor outcome and others showing no difference. Therefore, one goal of postresuscitative care is to deliver adequate oxygen to the patient while minimizing the risk of ongoing oxidative stress. Because many patients do not have arterial monitoring early after arrest, if a patient’s saturation is 100%, practitioners should wean the supplemental oxygen to a saturation goal of between 94% and 99%.
Ventilation
Arterial CO 2 levels directly affect cerebral vascular tone in healthy patients. Following cardiac arrest, cerebral edema may occur and titration of arterial CO 2 may affect blood flow. Hyperventilating patients can undergo cerebral vasoconstriction, with concerns of cerebral hypoperfusion of the already injured brain and increasing intrathoracic pressure, thus impairing cardiac output. Likewise, hypoventilation can lead to cerebral vasodilation and can increase already elevated intracranial hypertension.
Cardiovascular Support
Postarrest myocardial stunning and hypotensive shock occur commonly after successful resuscitation in children and adults, and are generally reversible among long-term survivors. Postarrest myocardial stunning is pathophysiologically similar to sepsis-related myocardial dysfunction and post–cardiopulmonary bypass myocardial dysfunction, including increases in production of inflammatory mediators and nitric oxide. Although the optimal management of post–cardiac arrest hypotension and myocardial dysfunction have not been established, adult data suggest that early hypotension in the 6 hours after ROSC is associated with increased mortality, therefore aggressive hemodynamic support may improve outcomes.
Initially fluid resuscitation should be provided for hypotension. If a central venous pressure is being monitored, volume should be titrated to increase the central venous pressures. Inotropic and/or vasopressor support should be provided for hypotension caused by myocardial dysfunction or vasodilation. Monitoring central venous pressure, central venous oxygen saturations, lactates, and urine output may help guide the effectiveness of therapies. General principles of critical care suggest that appropriate therapeutic goals are adequate blood pressure, adequate myocardial, cerebral, and systemic blood flows, and adequate oxygen delivery. Reasonable interventions for vasodilatory shock with low central venous pressure include fluid resuscitation and vasoactive infusions. Appropriate considerations for left ventricular myocardial dysfunction include euvolemia, inotropic infusions, and afterload reduction. Echocardiograms may contribute adjunctive data to guide therapies.
Temperature Management
Hyperthermia following cardiac arrest is common in children. Animal and human data show that fever following brain injury is associated with worse outcomes, therefore fever should be aggressively treated following cardiac arrest. Induced hypothermia (32° to 34°C) following witnessed adult VF cardiac arrest improves survival and neurologic outcomes. Induced hypothermia is the most clinically promising recent goal-directed postresuscitation therapy for adults. Interpretation and extrapolation of these studies to children is difficult, and potential benefits and risks are currently under study. Induced hypothermia may be considered for children who remain comatose after pediatric cardiac arrest; however, to date there are no prospective pediatric data proving efficacy.
Following ROSC, clinicians should continuously measure the patient’s core temperature (rectal, bladder, or esophageal). It is not uncommon for small children who have had a prolonged resuscitation to be hypothermic. Clinicians should discuss temperature goals with a tertiary care center. At a minimum, hyperthermia (>38°C) should be prevented with antipyretics and a cooling blanket. Similarly, hypothermia (<32°C) should be corrected, as deep hypothermia can lead to arrhythmias and repeat cardiac arrest.
Glucose Control
Hyperglycemia following adult cardiac arrest is associated with worse neurologic outcomes. However, hypoglycemia is associated with worse outcomes as well. Data on evidence-based titration of specific end points in children are not available, therefore clinicians should monitor frequent blood glucose levels and treat hypoglycemia.
Seizures
Seizures following cardiac arrest are common, and often are undetectable without electroencephalography (EEG). Postarrest seizures (clinical and subclinical) are associated with worse neurologic outcomes. Clinical convulsions should be aggressively treated and evaluated for correctable causes, and should be sought (eg, hypoglycemia, hyponatremia, hypocalcemia, hypomagnesemia). Many patients exhibit electrographic seizures that are undetectable to the clinician, even when not paralyzed. Therefore, clinicians should consider EEG monitoring of the comatose postarrest patient for seizure detection. If the patient is iatrogenically paralyzed, EEG monitoring will be the only means to detect postischemic seizure.
Prediction of neurologic outcome after both adult and pediatric cardiac arrests is limited. The predictive value of clinical neurologic examinations, neurophysiologic diagnostic studies (eg, EEG or somatosensory evoked potentials), biomarkers, or imaging (computed tomography, magnetic resonance imaging, or positron emission tomography) on eventual outcome following cardiac arrest is largely unknown. A burst-suppression pattern on postarrest EEG can be sensitive and specific for poor neurologic outcome. However, many children who suffer a cardiac arrest have substantial preexisting neurologic problems. Thus, comparison with prearrest neurologic function of a child is difficult, and adds another dimension/barrier to the assessment and prediction of postarrest neurologic status.
Emphasis on effective education and implementation programs
Current training is not adequate enough to maintain basic and advanced life-support skills and behaviors for critically ill children. Pediatric advanced life-support knowledge and skills decay rapidly after training, usually within 6 months. Frequent skill refresher training is needed to address knowledge and skill decay. Teamwork and behavioral skills are now recognized as key components of clinical skill performance. Highly realistic in situ simulation training has been used as an effective educational tool. However, the current translation of advanced life-support training to effective basic and advanced life-support clinical practice in real events is often less than desired.
In selected settings, simulation-based advanced life-support educational programs have improved compliance with national resuscitation guidelines for cardiac arrest in adults. Implementation of in situ simulation-based interdisciplinary pediatric mock code training has been associated with improvement of real patient survival-to-discharge following in-hospital cardiac arrest. In addition, simulation-based education has translated to improved patient process of care and patient outcomes in selected clinical settings. Such simulations are likely to drive resuscitation implementation in the future.
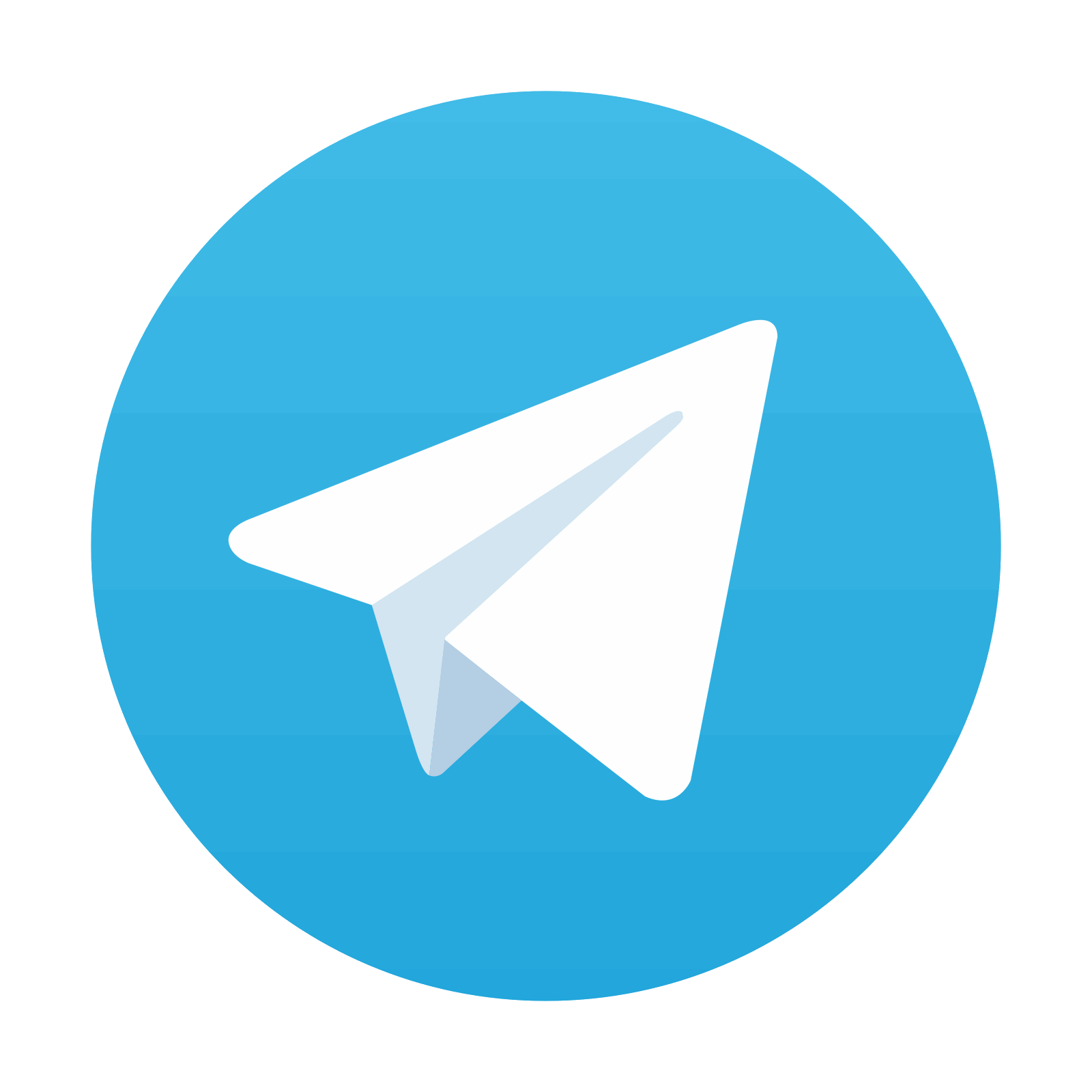
Stay updated, free articles. Join our Telegram channel

Full access? Get Clinical Tree
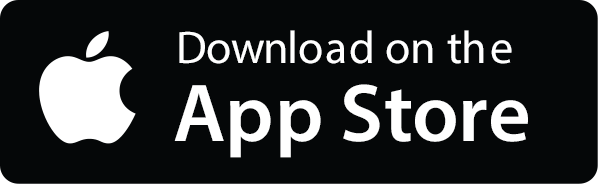
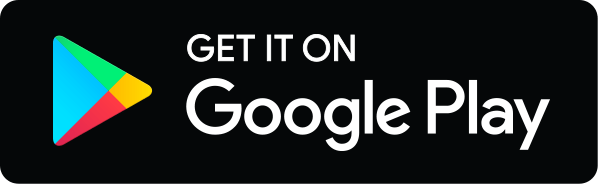