Acute Renal Failure and Oligoanuria
ARF, usually defined as an acute deterioration in the ability of the kidneys to maintain the homeostasis of body fluids (449), is associated with an acute decrease in the rate of glomerular filtration, as opposed to isolated acute tubular dysfunction. Because the placenta fulfills that role in utero, congenital malformations associated with limitation of renal function will not lead to renal failure until birth. ARF in newborn infants is suggested by a progressive increase in the infant’s serum creatinine above normal values for age in the context of normal maternal renal function (Fig. 42-5) (450,451).
Incidence
Diagnosis
Intrauterine oligoanuria can be suspected when oligohydramnios develops in the absence of amniotic fluid leakage or rupture of the amniotic membranes; it can be as a result of a congenital urinary tract anomaly (e.g., urinary tract obstruction, renal agenesis, dysgenesis), to toxins (e.g., ACE inhibitors, indomethacin) (235,453,454), or to intrauterine asphyxia. If urine output alone is used to assess renal function, ARF often will be either overlooked or over diagnosed. Indeed, normal urine output is found in approximately one-third of neonates with ARF (450, 455), although low urine output may occur in the absence of ARF. Infants who have been subjected to perinatal as-phyxia, shock, hypoxemia, or various nephrotoxins are at risk of development of ARF. Clinical signs of ARF include syndromes or signs suggestive of renal or urogenital malformations, oligoanuria, polyuria, hematuria, proteinuria, fluid overload, dehydration, cardiac arrhythmia, and systemic hypertension. Other signs include decreased activity, seizures, anemia, vomiting, and anorexia. Sometimes ARF is suspected on the basis of electrolyte abnormalities or elevated plasma levels of medications (e.g., aminoglycosides).
ARF is characterized by decreased GFR and renal tubular function compared to normal values for either postconceptional age or GA and postnatal age. Although the diagnosis of ARF is strongly suggested by a Pcr that is above the upper limit of normal, during the first days of life such an elevation also may result from abnormal maternal renal function. Thus, measuring GFR or following Pcr over time is required to diagnose ARF in a newborn infant during the first few days of life. Because renal tubular function changes with maturation, it is important to use criteria appropriate for age; indices developed for more mature subjects are not indicative of intrinsic renal failure in VLBW infants.
Etiology
Pathophysiology of Acute Tubular Necrosis
The evolution of acute tubular necrosis (ATN) is characterized by three successive phases: initiation, maintenance, and recovery. Although ATN may be precipitated by a single event, its development is multifactorial and involves vascular, i.e., hemodynamic, nephronal, and cellular, i.e., metabolic factors (449).
Vasoconstriction of the afferent arterioles plays a major role in the initiation phase of ATN (463,464 and 465). Increased renal vascular resistance results from changes in the relative concentrations of vasoconstrictive agents such as PRA, adenosine, thromboxane, endothelin, and platelet activating factor, and vasodilator agents such as natriuretic peptides, NO, PGs, and prostacyclin. The increase in PRA, which results from the stimulation of the juxtaglomerular apparatus by the high solute content of damaged tubules, helps maintain glomerular filtration by vasoconstricting the efferent arteriole. During the maintenance phase of ATN, the main factors are nephronal and cellular. The main cellular injury comes in the form of programmed cell death (apoptosis). Renal cellular injury is accompanied by release of free oxygen radicals, changes in intracellular calcium metabolism, phospholipid breakdown, altered cell polarity, and secondarily, loss of tight cell junctions between cells, loss of the cell brush border, and disruption of the cytoskeleton, leading to disruption of major cellular functions, e.g., Na+/K+-ATPase, and cell swelling. All these processes, except damage caused by free oxygen radicals, are accompanied by cellular ATP depletion, thereby limiting cellular restoration with reperfusion, an energy consuming process. When cellular ATP decreases, the concentration of intracellular heat shock transcription factor rises. Proximal tubular cells loaded with heat shock protein and then exposed to ischemic damage had significantly less ATP release after ischemia than nonheat shock protein-loaded cells and a marked reduction in apoptosis. Generation of heat shock protein and lactic dehydrogenase after anoxic injury in neonatal kidneys provides a better defense mechanism against ischemic renal injury compared to adult kidneys.
The production of local growth factors decreases during the early phase of ATN; the tubular epithelium only regenerates during the recovery phase. GFR initially increases in parallel with a decrease in intratubular pressure and casts; GFR then increases in parallel with RPF and urine output (452). Unless enough fluid is provided to compensate for the large amounts of urine lost during the polyuric phase, hypovolemia may develop and slow the recovery or even cause a secondary deterioration of renal function. Random-ized trials are needed to determine strategies to reduce the severity of ARF with the least side effects (466). Although many candidate drugs have been studied, none have proven effective in clinical trials.
TABLE 42-5 ETIOLOGIC CLASSIFICATION OF ACUTE NEONATAL RENAL FAILURE | ||
---|---|---|
|
Renal Blood Flow
Decreased RBF may result from multiple mechanisms such as reverse diastolic flow, hypotension, heart failure, and vasoconstriction (Table 42-5). In mothers with preeclampsia, absent or retrograde diastolic blood flow in the fetal aorta and UA predicts several perinatal complications, including renal failure (467).
RBF velocity remains low for at least 1 week in VLBW infants with intrauterine growth retardation (468). Prevention of prerenal failure includes correction of any abnormality in and maintenance of adequate oxygenation, ventilation, blood pressure, cardiac output, hydration (see Chapter 21), blood glucose, and hematocrit, and prevention and early treatment of sepsis. Preliminary data suggest that indomethacin-mediated renal vasoconstriction and ARF may be reduced by using prior volume expansion and by using a low dose and a slow infusion of indomethacin (469). Although furosemide increases urine output in patients receiving indomethacin for treating a PDA, the routine use of furosemide in these patients cannot be recommended (470,471).
Urine Output
In asymptomatic low-risk full-term infants receiving routine care, low or even absent urine output (detected by checking the number of wet diapers) during the first 24 hours of life is a normal event, assuming adequate fluid intake. If spontaneous urination is not observed within an additional 12 hours, i.e., at 36 hours of life, the baby should be reexamined for possible urinary retention, and the bladder should be catheterized.
In contrast, in premature infants and in patients with suggestive history, symptoms, or abnormal physical examination, urine output should be measured from birth. If there is no urine by 24 hours of life or if subsequent output is less than 1 mL/kg/h, the bladder should be catheterized; suprapubic pressure (i.e., the Credé maneuver) should be avoided, because of the risk of VUR, and rarely for bladder or renal rupture (472). Patients at high risk for renal failure (e.g., shock, extracorporeal membrane oxygenation [ECMO], or major surgery) should have their bladder catheterized to measure the hourly urine output.
If polyuria develops, possible diagnoses include hypervolemia, tubular dysfunction, diabetes insipidus, glucose overload, the polyuric phase of ATN, and the polyuric phase of postrenal failure (e.g., after decompression of the bladder by catheterization).
Perinatal Asphyxia
The risk for and severity of ARF increases with the severity of asphyxia (473,474 and 475). ARF associated with asphyxia is predominantly nonoliguric (474). Thus, unless Pcr is monitored daily in the severely asphyxiated neonate, renal failure will be easily missed. Early diagnosis of ARF secondary to perinatal asphyxia is suggested by the finding of increased tubular LMW proteinuria immediately after birth (476,477 and 478). Asphyxiated infants with a urine output less than 1 mL/kg/h for 36 hours usually have a high urinary concentration of beta2-microglobulin and clinical signs of hypoxic-ischemic encephalopathy. A dipstick may help detect hematuria, myoglobinuria, and proteinuria. Oligoanuria in these infants may result from prerenal, i.e., functional-mediated failure mediated in part by endothelin (479), intrinsic renal failure (e.g., ATN), or syndrome of inappropriate secretion of ADH (SIADH).
Prevention and Early Diagnosis of Acute Renal Failure
The first step is to identify infants at high risk for renal failure or oligoanuria immediately after birth or after predisposing events (Figs. 42-5 and 42-6). In these infants, urine collection should be initiated right away, and vital signs, electrolytes, and renal function should be followed serially. If urinary tract anomalies are suspected, a complete urologic workup should be considered (see Chapter 43). Decompression of the urinary tract by bladder catheterization or surgical intervention, or both, may be indicated. Oliguric renal failure develops in 22% of patients on venoarterial ECMO and may develop in patients on venovenous ECMO in the absence of changes in cardiac output, blood pressure, or hypoalbuminemia; the exact pathophysiology of the renal dysfunction in such infants remains to be elucidated (480,481).
General Approach in Oligoanuria
The diagnosis of oligoanuria, i.e., urinary output less than 0.5 to 1.0 mL/kg/h should be confirmed by demonstrating the absence of urine by bladder catheterization. Delayed micturition has been described in association with transient bladder distention attributed to severe perinatal asphyxia (482). Bladder puncture should be avoided because of the high likelihood of causing trauma.
Once oligoanuria is confirmed, the history should be reviewed for severe asphyxia, respiratory failure, hypotension, shock, and renal toxins. Intake and output over the last few days should be reviewed. The physical examination should seek evidence of weight change, dehydration, malformations, respiratory failure, CHF, shock, hypotension, and central nervous system disease. General care should be optimized, including measures to support ventilation, oxygenation, cardiac output, functional circulatory volume, and ECF volume.
Urine and Blood Analysis
The differential diagnosis of oligoanuria includes not only renal failure but also SIADH and decreased urine production secondary to the administration of ADH or its analogs (Table 42-6). The differentiation between prerenal and true renal ARF is presented in Table 42-7.
Urine should be examined promptly for protein, cellular elements and crystals, electrolytes, creatinine, and SG or osmolality. A blood sample should be obtained for hematocrit, electrolytes, creatinine, BUN, and glucose; other tests (e.g., serum concentration of xanthine or uric acid, oxaluria) may be indicated based on history or initial results. Plasma osmolality can be either measured or calculated from the following formula:
TABLE 42-6 DIFFERENTIAL DIAGNOSIS OF OLIGOANURIA | |
---|---|
|
TABLE 42-7 DIFFERENTIAL DIAGNOSIS: PRERENAL VS. INTRINSIC OLIGURIC RENAL FAILUREa | ||||||||||||||||||||||||||||||||||||
---|---|---|---|---|---|---|---|---|---|---|---|---|---|---|---|---|---|---|---|---|---|---|---|---|---|---|---|---|---|---|---|---|---|---|---|---|
|

Use of this formula is not recommended for infants with a BW less than 1,000 g, in whom it may substantially underestimate true osmolality (483).
While waiting for the results of these tests and ordering a renal US, and in some instances a renal scan, an initial therapeutic decision often can be made. This is especially important in patients in whom no urine can be obtained for analysis. Prerenal failure is a reasonable presumptive diagnosis in the presence of respiratory failure, CHF, dehydration, or shock, if osmolality or SG is high in the absence of casts in the urine. Rapid correction of the problem may be the only therapeutic intervention needed. The SIADH can be suspected in cases of severe neurologic dysfunction, pneumothorax, or pleural effusion, with weight gain, oligoanuria, high urinary SG and hyponatremia.
The urine obtained initially, i.e., before any diuretic or fluid challenge should be sent for urinalysis and urine chemistry for the assessment of tubular function. Various indices and tests have been proposed to differentiate ATN from prerenal or functional failure, by the presence of tubular dysfunction in ATN (484,485). FENa is the preferred index. A FENa greater than 3% (>6 % in preterm <32 weeks) (485) suggests a diagnosis of intrinsic failure and a value less than 2.5% a prerenal failure; however, several exceptions exist (Table 42-7). Renal failure index (RFI) is sometimes used as an alternative to FENa:

Cutoff points for diagnosis of ATN are RFI more than 4 in term and more than 8 in preterm infants less than 32 weeks.
Asphyxiated newborn infants may manifest transient tubular dysfunction associated with a normal or mildly decreased GFR and a normal or decreased urine output. Their tubular dysfunction is characterized by a positive dipstick test for blood, moderate tubular proteinuria, i.e., LMW proteins and N-acetyl-β-D-glucosaminidase [NAG], and a FENa that is either normal or mildly increased (476,477,486,487 and 488). In contrast, ATN is associated with marked proteinuria, markedly decreased GFR, and often oligoanuria. Urinary concentration of myoglobin and retinol-binding protein, but not NAG, can be used to differentiate between normal transient tubular dysfunction and ATN (476,477). Renal and bladder US may establish a diagnosis of urinary tract obstruction, uro-ascites, or renal cystic disease (see Appendix G-4), suggest renal vein or artery thrombosis, adrenal hemorrhage, or show abnormal cortical, medullary, or pyelocalicial echogenicity (see Table 42-4) (435,436,437,438,489,490,491). One common finding is increased echogenicity of the pyramids (494,495), which may correspond to the precipitation of Tamm-Horsfall protein in patients with ATN.
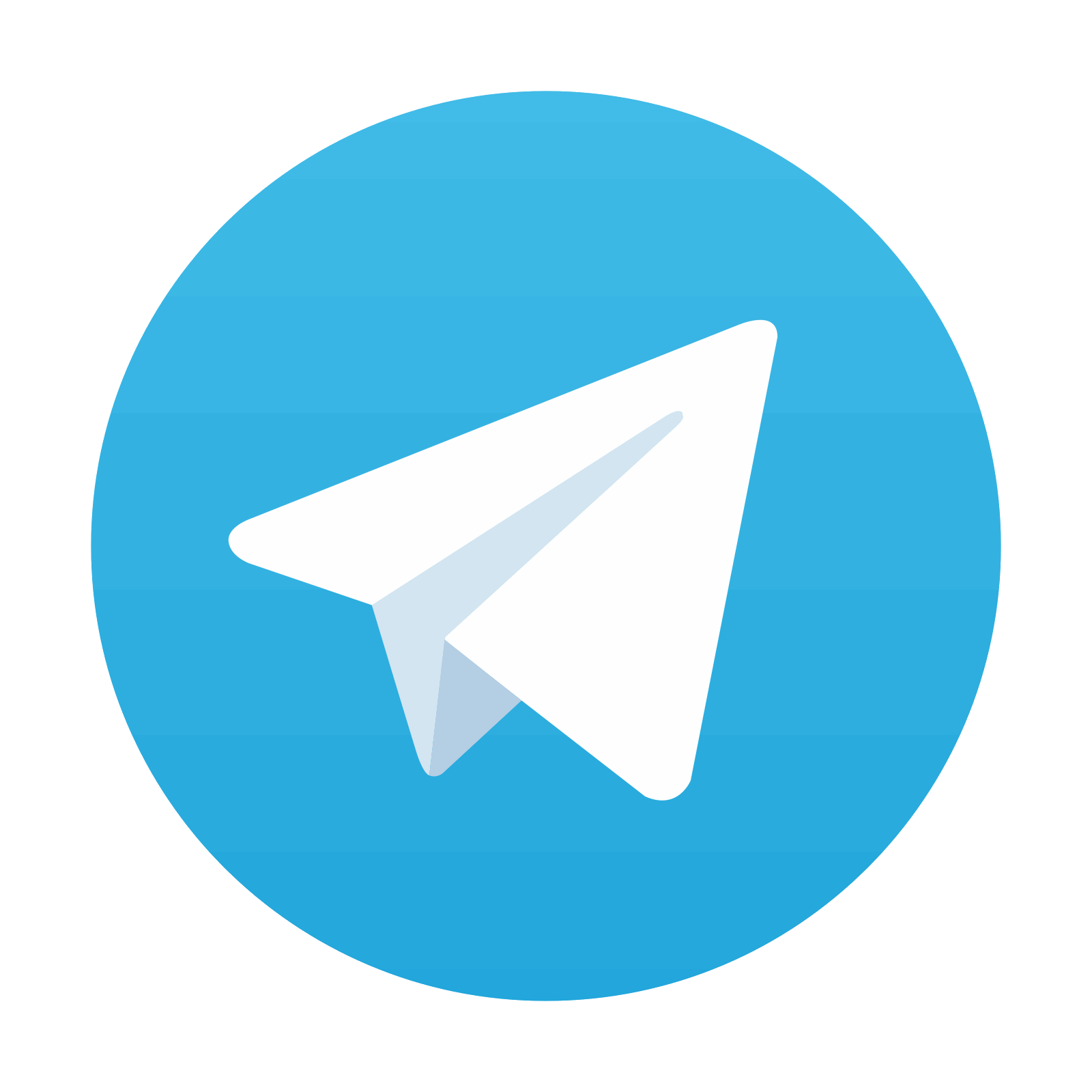
Stay updated, free articles. Join our Telegram channel

Full access? Get Clinical Tree
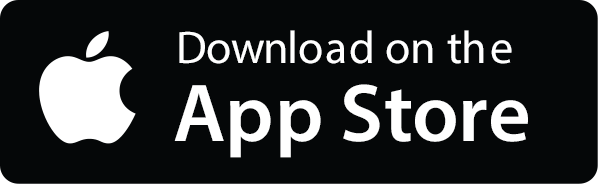
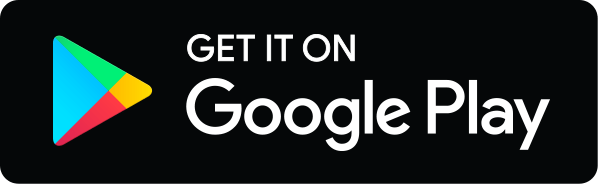