The concept and definition of acute kidney injury (AKI) in adults and children has undergone significant change in recent years. Biomarker assessment is aiding in description, defining and understanding timing of AKI. AKI demonstrates unique characteristics in association with sepsis and septic shock, organ dysfunction, and fluid overload. Treatment remains problematic, but growing experience with pediatric continuous renal replacement therapies has improved the delivery of care in children. Increasingly, continuous renal replacement therapy is provided in combination with other extracorporeal technologies, and approaches are advancing to improve combined therapy use.
Key points
- •
The definitions and characterization of acute kidney injury (AKI) in children have advanced significantly over the past 2 decades.
- •
AKI is common in critically ill children and is associated with increased morbidity and mortality.
- •
AKI in association with sepsis, multiple organ involvement, and fluid overload carries heightened risk.
- •
Gene probes and urinary biomarkers represent intriguing tools for predicting and monitoring pediatric AKI, as well as potentially guiding treatment intervention.
- •
Treatment of AKI is problematic, but extracorporeal approaches in AKI and multiple organ system failure continue to grow in use and potential benefit.
“In the kidneys are seated reasonings, and there dwells in them the faculty of discernment; they distinguish truth from falsehood, and judge what is base and what is noble.” —Saint Ephraem (ca 306–373 CE)
Ancient authors viewed the kidneys to be both the seats of reason and of discernment. The kidneys, later termed “reins” in the English vernacular, were also seen as a source of divine punishment. Job described, among his many maladies, “my reins consume me.” Although our scientific understanding of the kidney has markedly evolved, we still find that the kidney does provide both a marker of outcome in critically ill adults and children, and a potential target for improving morbidity and mortality. This review focuses on updates in definition, epidemiology and outcomes, associated organ injury and sepsis, and treatment options for the critically ill child with acute kidney injury (AKI).
The profound clinical and translational research transformation observed in the AKI field over the past decade has been generated in large part by 3 major advancements: understanding that AKI is an independent risk factor for mortality, development and refinement of standardized multidimensional AKI definitions, and discovery and validation of novel urinary AKI biomarkers that reflect kidney damage before loss of kidney function. The concept that patients were dying “from” and not just “with” renal failure provided the impetus to change the terminology from acute renal failure (ARF) to AKI. The acceptance of this terminology change in the research literature was quite rapid, and reflected a controversial paradigm shift in how to define the AKI syndrome clinically. A seminal publication by Chertow and colleagues demonstrated increases in serum creatinine of as little as 0.3 mg/dL were independently associated with patient morbidity and mortality. These findings underscored the inadequacy of creatinine change as an early marker of disease. Price and colleagues observed a similar association between a rise of 0.3 mg/dL in serum creatinine and poor outcomes in children with acute decompensated heart failure, where this rise conferred an adjusted odds ratio (OR) of 10.2 (95% confidence interval [CI] 1.2–61.1) for the combined end point of mortality or need to go on to mechanical circulatory support. Reliance on serum creatinine emanated from its use to estimate glomerular filtration rate to define stages of chronic kidney disease, guide medical intervention, and direct the initiation of maintenance renal replacement therapy. Because serum creatinine change occurs only with more than 50% loss of functional nephron mass, it is not surprising that small changes in this functional marker can portend poor prognoses, leading to the need to standardize the AKI definition reflecting these associations.
In 2004, the Acute Dialysis Quality Initiative, a work group of nephrologists and critical care physicians, developed the first consensus multidimensional AKI definition, termed the RIFLE criteria. RIFLE has 3 AKI staging strata, Risk, Injury, Failure, and 2 outcome criteria, Loss and End-Stage Kidney Disease. AKI development by RIFLE (RIFLE-R) is defined as a 50% rise in serum creatinine (or 25% decrease in estimated creatinine clearance) over baseline or by urine output of less than 0.5 mL/kg per hour for 6 hours. RIFLE-I and RIFLE-F are each defined by greater changes in creatinine or urine output (UOP). Studies in adult patients in many different settings, including the intensive care unit (ICU), emergency center, and post cardiac surgery, have validated the RIFLE criteria. A recent literature review of these studies in more than 500,000 patients demonstrated increased independent mortality for each increasing RIFLE strata. In 2007, we developed and validated a pediatric modified version of the RIFLE criteria (pRIFLE) in 150 critically ill children receiving invasive mechanical ventilation. In this study, children who developed pRIFLE-I or pRIFLE-F had higher mortality or more prolonged AKI than patients with pRIFLE-R or no AKI. A recent systematic review of 11 pediatric studies comprising nearly 10,000 patients (ICU, emergency center, post cardiac surgery, and nephrotoxic medication–associated AKI) revealed similar associations, although the application of pRIFLE was inconsistently applied in these studies.
The RIFLE was modified by the Acute Kidney Injury Network (AKIN) in 2007 to include a 0.3 mg/dL serum creatinine rise in less than 48 hours for the AKI definition ( Table 1 ). The pRIFLE and AKIN definitions have been used in pediatric studies, and yield similar results; RIFLE-I (AKIN Stage II) or worse is associated with increased patient morbidity. In 2012, an international guideline developed by the Kidney Disease Improving Global Outcomes (KDIGO) Acute Kidney Injury Work Group harmonized RIFLE, AKIN, and pRIFLE into a single standardized definition (see Table 1 ). The KDIGO AKI definition and staging criteria should be used at the current time unless further modifications are warranted by prospective study.
pRIFLE Criteria | AKIN Criteria | KDIGO Criteria | ||||||
---|---|---|---|---|---|---|---|---|
Stage | SCr-Based | Urine Output | Stage | SCr-Based | Urine Output | Stage | SCr-Based | Urine Output |
Risk | >25% eCCl decrease | <0.5 mL/kg/h for 8 h | I | SCr increase ≥0.3 mg/dL OR 150%–200% in ≤48 h | <0.5 mL/kg/h for 8 h | I | SCr increase ≥0.3 mg/dL in 48 h OR 1.5–1.9 times | <0.5 mL/kg/h for 6–12 h |
Injury | >50% eCCl decrease | <0.5 mL/kg/h for 16 h | II | SCr increase 200%–300% | <0.5 mL/kg/h for 16 h | II | SCr increase 2.0–2.9 times | <0.5 mL/kg/h for 12 h |
Failure | >75% eCCl decrease OR eCCl <35 mL/min/1.73 m 2 | <0.5 mL/kg/h for 24 h OR <0.3 mL/kg/h for 12 h | III | SCr increase 200%–300% OR SCr >4.0 mg/dL | <0.5 mL/kg/h for 24 h OR <0.3 mL/kg/h for 12 h | III | SCr ≥3.0 increase OR SCr > 4.0 mg/dL OR if <18 y of age then eCCl <35 mL/min/1.73 m 2 | <0.5 mL/kg/h for 24 h OR <0.3 mL/kg/h for 12 h |
The role of excessive fluid accumulation and its association with poor outcome in critically ill children is discussed later in this article; however, the potential for fluid accumulation to dilute serum creatinine concentration and alter the ascertainment of AKI based on serum creatinine change has been examined recently. In a post hoc analysis of the Fluid and Catheter Treatment trial, Liu and colleagues corrected serum creatinine concentration based on fluid status. In this analysis, patients who fulfilled AKI by serum creatinine–based criteria after, but not before, fluid overload (FO) correction had significantly higher mortality than patients who did not meet AKI criteria before or after FO correction. In a study of infants after arterial switch operation, we found correction of serum creatinine for fluid balance increased the number of patients with severe AKI and strengthened the association of AKI with mortality.
The repeated demonstration of serum creatinine as a late and unmodifiable functional AKI marker has led to a more than decade-long research effort to identify novel urinary AKI biomarkers that reflect kidney injury development and severity earlier than serum creatinine. Numerous biomarkers discovered from animal models of AKI have been validated in humans and include neutrophil gelatinase–associated lipocalin (NGAL), kidney injury molecule-1 (KIM-1), interleukin-18 (IL-18), and liver type fatty acid binding protein, all of which are upregulated in the setting of ischemic kidney injury. Additional biomarkers that leak from tubular cells, such as alpha-glutathione-S-transferase (GST) and pi-GST, are also being investigated. Although it is beyond the scope of this update to review the entire AKI biomarker literature (please see Devarajan for the most recent review), these markers hold promise to identify patients at risk for acute functional kidney decline, much as the troponins have revolutionized identification and directed early management of the acute coronary syndrome to prevent myocardial infarction in adults. In fact, a recent meta-analysis of NGAL across 10 adult and pediatric studies demonstrate similarly poor outcomes for patients with NGAL (+)/serum creatinine (–) and serum creatinine (+)/NGAL (–) AKI. In the future, once these biomarkers have been used widely in clinical practice and linked to clinical outcomes, it is likely that AKI will become defined by biomarker elevations and not by serum creatinine.
AKI epidemiology and incidence
The epidemiology of AKI has likewise changed over the past 2 decades, transitioning from primary renal disease to a syndrome secondary to other systemic illness or its treatment. Advances in medical management for other organ illnesses, including solid organ and stem cell transplantation, corrective congenital heart surgery, sepsis, and septic shock, have depended on medicinal and mechanical therapeutic interventions that have nephrotoxic side effects. For example, numerous studies demonstrate cardiopulmonary bypass (CPB) to be associated with a 20% to 40% AKI rate. Nephrotoxic medications are a commonly reported cause of AKI in critically ill and non–critically ill children, which is independently associated with morbidity and mortality in children. Finally, nonrenal disease accounts for 80% of the underlying illnesses associated with the continuous renal replacement therapy provision in children. Thus, this epidemiologic shift requires non-nephrologists to be on the lookout for factors that place children at risk for AKI and early signs of AKI to optimize management to prevent or mitigate the effects of AKI. The KDIGO AKI guidelines provide a stage-based management scheme for AKI that incorporates risk assessment in the model.
AKI is quite common in critically ill children, with incidence ranging from 1% to 82% of all ICU or postoperative CPB admissions, as categorized in an excellent review. AKI as defined by AKIN criteria was found in 17.9% of 2106 admissions in a large Canadian pediatric ICU (PICU) cohort and in 10% of 3396 PICU patients by RIFLE criteria. AKI is associated with worsened outcomes in children. AKI in the Canadian study was an independent risk factor for mortality (OR 3.7), increased length of stay, and prolonged mechanical ventilation ; ICU length of stay was increased fourfold. Reported mortality in children with AKI receiving continuous renal replacement therapies (CRRTs) has ranged from 32.1% to 58.9% in studies since 2000. AKI synergistically increases morbidity and mortality in children with multiple organ failure, hematopoietic stem cell transplantation, trauma, and extracorporeal membrane oxygenation, independent of severity of illness scoring. Effects of AKI are also long lasting in survivors, with chronic renal insufficiency in almost half of patients at 3-year to 5-year follow-up, suggesting permanent alteration of the renal parenchyma.
AKI epidemiology and incidence
The epidemiology of AKI has likewise changed over the past 2 decades, transitioning from primary renal disease to a syndrome secondary to other systemic illness or its treatment. Advances in medical management for other organ illnesses, including solid organ and stem cell transplantation, corrective congenital heart surgery, sepsis, and septic shock, have depended on medicinal and mechanical therapeutic interventions that have nephrotoxic side effects. For example, numerous studies demonstrate cardiopulmonary bypass (CPB) to be associated with a 20% to 40% AKI rate. Nephrotoxic medications are a commonly reported cause of AKI in critically ill and non–critically ill children, which is independently associated with morbidity and mortality in children. Finally, nonrenal disease accounts for 80% of the underlying illnesses associated with the continuous renal replacement therapy provision in children. Thus, this epidemiologic shift requires non-nephrologists to be on the lookout for factors that place children at risk for AKI and early signs of AKI to optimize management to prevent or mitigate the effects of AKI. The KDIGO AKI guidelines provide a stage-based management scheme for AKI that incorporates risk assessment in the model.
AKI is quite common in critically ill children, with incidence ranging from 1% to 82% of all ICU or postoperative CPB admissions, as categorized in an excellent review. AKI as defined by AKIN criteria was found in 17.9% of 2106 admissions in a large Canadian pediatric ICU (PICU) cohort and in 10% of 3396 PICU patients by RIFLE criteria. AKI is associated with worsened outcomes in children. AKI in the Canadian study was an independent risk factor for mortality (OR 3.7), increased length of stay, and prolonged mechanical ventilation ; ICU length of stay was increased fourfold. Reported mortality in children with AKI receiving continuous renal replacement therapies (CRRTs) has ranged from 32.1% to 58.9% in studies since 2000. AKI synergistically increases morbidity and mortality in children with multiple organ failure, hematopoietic stem cell transplantation, trauma, and extracorporeal membrane oxygenation, independent of severity of illness scoring. Effects of AKI are also long lasting in survivors, with chronic renal insufficiency in almost half of patients at 3-year to 5-year follow-up, suggesting permanent alteration of the renal parenchyma.
AKI and associated conditions
Sepsis-Associated AKI
AKI is a common finding in sepsis, both as a cause and as an effect, and often in association with multiple organ dysfunction. Septic AKI is defined as the simultaneous presence both of RIFLE criteria for AKI and consensus criteria for sepsis, with the absence of other clear and established nonsepsis-related causes of AKI (eg, radiocontrast, other nephrotoxins). Sepsis has been noted as the primary cause of up to 50% of adult AKI. In pediatric AKI series, incidence of sepsis-associated AKI has ranged from 9% to 34%. Sepsis-associated AKI is also associated with lower survival. The pathogenic mechanisms of AKI in sepsis are complex and multifactorial, but are most likely related to combination of blood flow alterations and cytokine-mediated injury ( Fig. 1 ). Resultant injury produces both necrotic and apoptotic renal cell death. Regional or global hypoperfusion has long been thought to be responsible for primary injury, with an associated proinflammatory state adding injury. Likely the answer is more complex. For instance, sepsis-associated AKI can occur even in the face of hyperdynamic renal blood flow. The “peak concentration hypothesis” has been proposed to suggest that elevations and imbalances of both proinflammatory and anti-inflammatory mediators coupled with endothelial dysfunction and altered coagulation cascade can all function synergistically to induce AKI. The importance of microthrombosis in AKI has been demonstrated by findings that deficiency of ADAMTS-13 (formerly known as von Willebrand factor–cleaving protease), a cardinal finding in thrombotic thrombocytopenic purpura (TTP), is found in septic AKI patients. ADAMTS-13 deficiency in this setting is associated with increased mortality. Specific cytokine gene promoter polymorphisms have also been suggested to predispose some patients with sepsis to develop AKI, and to increase likelihood of mortality in sepsis-associated AKI. Specific gene probes for matrix metalloproteinase-8 and elastase-2 were upregulated in pediatric septic shock–associated AKI with high sensitivity, albeit low specificity, suggesting the potential for serum biomarkers in predicting AKI.

AKI and Other Organ Involvement
Although AKI manifests itself most commonly as single organ failure, it frequently occurs in concert with other organ failure in critically ill children. Children with multiple organ system failure (MOSF) have decreasing survival with increasing number of organ failures, and AKI is a synergistic contributor to this mortality.
The complex pathophysiologic interaction of heart and kidney dysfunction in children has been increasingly recognized, described as cardiorenal syndrome (CRS). CRS may occur as numerous subtypes, but is primarily caused by acute cardiac disease with secondary acute renal impact (type 1), or vice versa (type 3). Systemic disease can also result in secondary heart and kidney involvement. In children, type 1 CRS is seen most commonly in the perioperative setting with acute decompensated heart failure. AKI was seen in 27% of patients in a postoperative pediatric CPB cohort. Diagnosis of severe AKI after pediatric CPB was also associated with worsened left ventricular function 30 days after CPB. Recent studies have identified several urinary biomarkers, including NGAL, IL-18, and KIM-1, as well as serum biomarkers, such as cystatin C, that can detect AKI children after CPB. They also correlate with AKI severity and outcome. Thus, early diagnosis of CRS and/or AKI through use of biomarkers could help guide use of peritoneal dialysis or ultrafiltration immediately in the postoperative period. Adult studies are ongoing and pediatric trials may be warranted. Excellent pediatric recommendations regarding CRS are found in the review by Jefferies and Goldstein. The interaction of liver and kidney in hepatorenal syndrome is well recognized, although pathophysiologic mechanisms remain elusive.
AKI and Fluid Overload
Development of AKI would be expected to contribute inherently to FO from decreased renal function and oliguria; however, evidence also suggests that FO itself may contribute to AKI. A number of pediatric and adult studies have supported the association of degree of FO and worsening outcome. Goldstein and colleagues first reported this association. In children receiving continuous venovenous hemofiltration (CVVH) (either alone or with dialysis), percent FO at initiation of hemofiltration was significantly lower in survivors (16.4% ± 13.8%) compared with nonsurvivors (34.0% ± 21.0%), even when controlled for severity of illness. These findings were supported in analysis by Foland and colleagues of a larger population of 113 critically ill children receiving CVVH for a variety of conditions associated with both primary and secondary renal insufficiency. Multiple organ dysfunction syndrome (MODS) was seen in 103 of these patients. Median FO was significantly lower in survivors compared with nonsurvivors (7.8% vs 15.1%, P = .02). Percent FO was independently associated with survival in patients with 3 or more organ MODS, adjusting for severity of illness by PRISM III score ( Fig. 2 ). Children with high FO (>10%) at CVVH initiation were at 3 times greater risk of mortality ( P = .002). Another single-center study also found that pediatric CRRT survival outcomes progressively worsened with increasing percent FO ( Fig. 3 ). Subsequently, prospective observation data from the 13 centers of the Prospective Pediatric Continuous Renal Replacement Therapy Registry confirmed the association between FO and outcomes in pediatric patients with AKI selected to receive CRRT. In these studies, mortality increased with increasing deciles of percent FO, even when adjusted for intergroup differences in severity of illness scores. Children with greater than 20% FO had an adjusted mortality OR of 8.5 times those less than 20%.
Several prospective AKI studies later confirmed pediatric findings more broadly in adolescents and adults with AKI; fluid balance was an independent risk factor for mortality, and early institution of renal replacement therapy was associated with better outcome. The Program to Improve Care in Acute Renal Disease (PICARD), a 618-patient adult AKI observational study, found that FO was associated with mortality and nonrecovery of kidney function (adjusted OR of 2.07 for death associated with FO at time of dialysis initiation), persisting even when adjusted for Acute Physiology and Chronic Health Evaluation (APACHE) and Sequential Organ Failure Assessment (SOFA) severity of illness scores. The data also suggested that aggressive use of diuretics and early initiation of hemofiltration might be associated with improved outcomes. Although adjusting for severity of illness, all these AKI studies do not test the effects of treating FO on outcome and are unable to determine causation. Randomized studies would be important to further evaluate therapeutic benefit in children with AKI.
Treatment of AKI in children
Although diagnostic classification and characterization of AKI has significantly improved, treatment remains problematic. In part this is a result of the broad etiologies of AKI and multifactorial causes. Potential therapies, without conclusive data in adults, are even less well defined for use in children.
Medical Therapies
Numerous medical approaches have been attempted for prevention and treatment of AKI. Trials assessing such AKI treatments are hampered on the reliance of serum creatinine as the marker of AKI. Because, as noted previously, serum creatinine is a late functional marker of AKI, it is unlikely that any treatment is likely to be effective once more than 50% of the functional nephron mass is affected. The most common therapeutic agent used and studied in the setting of AKI has been furosemide, a loop diuretic that causes diuresis, as well as natriuresis and kaliuresis. Bagshaw and colleagues performed a comprehensive meta-analysis of furosemide trials in AKI, and determined that whereas most patients with AKI receive diuretics, they led to no improvement in outcomes.
AKI preventive therapies and AKI prevention trials depend on knowing the precise timing of AKI or detection of an early biomarker of injury to allow for early and optimized directed therapy. The 2 settings most conducive to such study are radiocontrast administration and CPB. Indeed, the CPB setting has been the proving ground for most AKI biomarker studies. Medications tested in this setting have included fluid administration, sodium bicarbonate, diuretics, n-acetylcysteine (NAC), statins, low-dose dopamine, fenoldopam, and theophylline. Although some initial success was seen with NAC in preventing contrast-induced nephropathy, subsequent large randomized trials have yielded negative or equivocal results. Similar disappointing patterns of early success for AKI treatment or prevention followed by lack of substantiation have been observed with almost all the medications listed previously.
Given the potential role of ischemia in AKI, efforts to limit or improve renal blood flow have always been attractive; however, pharmacologic approaches have not proven successful in clinical studies. The classic use of “renal dose” dopamine has been convincingly shown to be ineffective in decreasing need for renal replacement therapy or mortality, and is not recommended. Maintenance of appropriate arterial blood pressure should still be considered essential to optimize renal perfusion.
It is important to note that both low-dose dopamine and loop diuretics may be harmful for AKI prevention, and their use in this context has been largely abandoned. On a positive note, Ricci and colleagues demonstrated that high-dose fenoldopam given during cardiac bypass decreases urinary NGAL and cystatin C levels, as well as serum creatinine–based AKI severity by pRIFLE. This novel trial design, using novel biomarkers as the end point and incorporated in the definition of AKI, should inform future studies going forward.
Metabolic Control
Efforts to maintain euglycemia have received much attention in light of a seminal randomized trial demonstrating reduced mortality in surgical patients achieving tight glycemic control. The suggested mechanism of benefit of insulin is induction of anti-inflammatory and antiapoptotic effects, in line with the suggested mechanisms of septic AKI. Reduction of AKI has accordingly been suggested to benefit from tight control in critical illness hyperglycemia. In the study by van den Berghe and colleagues, severe acute renal failure requiring dialysis or CRRT was reduced (8.2% vs 4.8%; P = .04), as well as reduced numbers of patients with elevated creatinine, in patients receiving tight control. Subsequent studies in medical patients have been less encouraging in mortality benefit, although AKI was again reduced, as based on I or F criteria of RIFLE (8.9% vs 5.9%). Subsequent trials in both adults and children have not found significant effects on reduction of AKI, or need for dialysis.
CRRT
CRRT has become recognized as the preferred modality for management of the child with severe AKI with electrolyte abnormalities and FO. Several excellent reviews provide much information on technical details, timing, and dosing. Benefits of CRRT over intermittent hemodialysis and peritoneal dialysis remain the technique’s ability to provide gradual but constant removal of solutes and independent adjustment of composition and volume of extracellular fluid, minimizing the hemodynamic impact in critically ill patients. An additional theoretical benefit of CRRT has been the potential immunomodulatory effect of inflammatory cytokine removal, related to the classic “peak concentration” hypothesis. Although a multitude of studies have identified decrease in cytokines with CRRT, related both to filtration and adsorption to the membrane, no studies have correlated degree of cytokine removal with outcome in septic patients.
While biologically plausible and intuitively advantageous, randomized trials have unfortunately not demonstrated outcome efficacy of CRRT compared with intermittent hemodialysis therapy in critically ill adults. Prospective randomized studies of CRRT compared with standard approaches are lacking in children; however, data from the 13-center Prospective Pediatric Continuous Renal Replacement Therapy (ppCRRT) registry have provided an extensive window into indications for and use of CRRT in children. More than 90% of patients in the registry have received CRRT to treat metabolic or fluid disturbances directly related to AKI. Reports from the ppCRRT registry in patients receiving CRRT for AKI and MOSF have supported the association of FO and outcome and the early initiation of CRRT. Anecdotally, mortality in these CRRT series is lower than might be expected from historical series of children with MOSF not treated with CRRT.
Dosing of CRRT has been postulated to accordingly improve outcome. Indeed, an early landmark randomized trial by Ronco and colleagues found significant improvement in survival with high-flow ultrafiltration (35 mL/kg/h) compared with a lower dose (20 mL/kg/h); however, subsequent single-center and multicenter trials found no difference by intensity. In particular, the Randomized Evaluation of Normal versus Augmented Level Replacement Therapy (RENAL) trial of 1508 adults exclusively receiving continuous venovenous diahemofiltration (CVVDHF) found no difference in survival or renal replacement need at 60 days between the high-intensity (33.4 ± 12.8 mL/kg/h) group and the low-intensity group (22 ± 17.8 mL/kg/h). The cumulative evidence thus leads to the recommendation that provision of an effectively delivered standard dose of renal replacement therapy should be sought over seeking to increase intensity of therapy. Some single-center experience has suggested outcome benefit of convective hemofiltration as a mode compared with diahemofiltration, perhaps because of enhanced cytokine removal in sepsis. However, ppCRRT results did not find comparative benefit in nonrandomized review. Timing of CRRT initiation is also still not well delineated. Although evidence favors earlier initiation to minimize FO, no study has been able to successfully assess best-practice cutoff points. Use of urinary biomarkers in combination with FO categories could provide a mechanism for identifying timing; further studies are in progress.
Anticoagulation is a key element of effective CRRT. Although heparin had been a mainstay of therapy, use of sodium citrate anticoagulation with calcium infusion has achieved widespread and majority acceptance because of the ability to provide regional anticoagulation of the circuit, decrease bleeding risk, and minimize heparin exposure. The protocol of Bunchman and colleagues is the most commonly used citrate approach. Patients on protocol must be monitored closely for evidence of citrate overload, particularly in patients with impaired hepatic function.
Plasma Exchange in AKI and MOSF
The use of plasma exchange has markedly improved survival and AKI outcomes of TTP. Findings of a TTP-like subtype of patients, most with sepsis, thrombocytopenia, and multiple organ failure (TAMOF) have led to efforts to use plasma exchange in children. A small randomized trial of pediatric patients with TAMOF found benefit in reduction of organ dysfunction scores. Preliminary results from a more recent multicenter prospective observational trial of children with TAMOF demonstrated reduced ADAMTS-13 levels, a high incidence of AKI, and need for CRRT. Plasma exchange was associated with a more rapid reduction in organ dysfunction scores and with decreased mortality in preliminary studies; further prospective randomized trials are necessary to determine outcome benefit on survival and AKI compared with standard therapies.
Use of CRRT With Extracorporeal Life Support
Treatment of AKI and multiple organ failure is common in the PICU, and often requires multiple extracorporeal devices, including extracorporeal life support (ECLS) to provide temporary cardiopulmonary support for patients with severe, potentially reversible heart or lung failure when traditional medical managements have failed. Renal findings of oliguria/anuria, FO, transient impairment of renal function, and combined renal hypoperfusion and hypoxemia all are manifestations of AKI in this population. AKI rates in patients receiving ECLS include 25% in neonates, 18% to 30% in pediatric patients, and 50% in adult patients. Specific subpopulations, such as those receiving CPB for repair of congenital heart disease, have much higher reported AKI rates, up to 71% in one series.
Optimal management of these renal complications in patients receiving ECLS has not been definitively determined. Traditionally, management of FO on ECLS has focused on increasing urine output by using diuretics and restricting fluid intake to achieve a net negative daily fluid balance; however, this approach has recently been called into question. Aggressive management of FO can be performed via high diuretic dosages in critically ill patients with AKI, but is associated with increased risk of death, hearing loss, nonrecovery of renal function, and lowered caloric intake due to fluid restriction with poorer outcome. Although impact on survival is unknown, early intervention with hemofiltration in neonatal and pediatric ECLS has been shown to improve fluid balance, increase caloric intake, and reduce total dose of diuretics.
Much variation currently exists in prevention and management of these ECLS complications. In a recent survey of Extracorporeal Life Support Organization (ELSO) centers providing ECLS, Fleming and colleagues found that 23% of centers did not routinely use any renal supportive therapies (intermittent hemodialysis, sustained low-efficiency dialysis, CRRT, slow continuous ultrafiltration) for their patients receiving ECLS, whereas another 26% included a hemofilter to provide these therapies in every ECLS circuit. The most common indications for use of renal supportive therapies were treatment of FO (43%), acute kidney injury (35%), prevention of FO (16%), and electrolyte disturbances (4%). Of centers using a renal supportive therapy, most used convective therapies (61.4%). Differences between devices used, connection points into the ECLS circuit, and techniques used to provide these therapies were seen as well. Nephrology consultation in the management of these patients varied widely (94.0% consultation rates in the United States compared with 5.6% in all other countries). The survey provided evidence of the variability in the diagnosis and treatment of renal complications in patients receiving ECLS and informs future studies aimed at identifying optimal therapy.
ELSO provides patient care guidelines addressing the management of fluid balance and kidney injury in these patients. Because of edema and FO caused by critical illness and iatrogenic crystalloid infusion worsening lung/heart failure, ELSO recommends a management goal “to return the extracellular fluid volume to normal (dry weight) and maintain it there.” To achieve this, guidelines suggest institution of diuretics once the patient is hemodynamically stable and if this effect “is not sufficient to achieve negative fluid balance or if the patient is in overt renal failure, [then] continuous hemofiltration is added to the extracorporeal circuit to maintain fluid and electrolyte balance.” ELSO guidelines also address the misperception that use of renal replacement therapies concomitantly with ECLS causes both acute and chronic kidney failure, commenting that renal replacement therapies are “often used” on ECLS, can be useful in helping provide full caloric and protein nutritional support, and do “not cause renal failure.” Development of chronic renal failure after concomitant CRRT and ECLS therapy is rare outside of a primary kidney disease and resolution of kidney injury occurs “in >90% of patients.” Balancing the risks of FO and use of renal replacement therapy in patients receiving ECLS is important because both are associated with death in ELSO registry and individual center patient reviews.
Several approaches are currently in use for providing renal replacement therapy on ECLS. A brief review of the 2 most common methods, in-line hemofiltration and connection of a dedicated CRRT device to the ECLS circuit, is provided, with more detailed information available elsewhere. No studies have been performed to evaluate comparison devices, although single-center experience has suggested improved outcomes in the time period of dedicated CRRT device use. Of note, no currently available CRRT device approved by the Food and Drug Administration exists to date in the United States for use in neonatal or pediatric populations, nor any device approved for use in conjunction with ECLS. Regardless of technique used, the use of commercially prepared replacement/dialysis fluids and use of standardized protocols are critical to providing safe and effective care.
One common method for CRRT in ECLS used in many institutions is a simplified in-line hemofilter device that does not require addition of a dialysis machine to the circuit. Renal replacement circuit flow is driven by ECLS pump flow rather than requiring an additional blood pump. In this standardized procedure ( Fig. 4 ), a primed renal replacement circuit is inserted in-line and parallel with the existing ECLS circuit. The renal replacement circuit is constructed using a hemofilter/hemodialyzer with CRRT tubing. The filter inlet is connected after the ECLS roller pump, the outlet is connected before the pump, and, where available, to the ECLS bladder. Monitoring of actual delivered flow with an ultrasonic flow probe on the arterial limb after all shunts is recommended to ensure adequate oxygen delivery. The blood path circuit is altered with both inlet and outlet to the renal replacement circuit being post-pump but pre-oxygenator when using a centrifugal pump owing to negative pressures seen on the venous pre-pump limb of the circuit. Regardless of pump type used, the outlet blood should be returned before the oxygenator so that it serves as a trap to avoid sending unanticipated air or clot from the hemofiltration limb to the patient. Existing anticoagulation provided for the ECLS circuit is usually sufficient, and no additional anticoagulation of the renal replacement circuit is needed. Ultrafiltrate from the hemofilter is removed using intravenous (IV) fluid infusion tubing and an IV pump, which is intended to control the hourly fluid removal rate and is measured by a urometer when the device is set up to provide slow continuous ultrafiltration (SCUF). To provide CVVH, a second IV pump is used to deliver replacement fluids to the blood before returning to the ECLS circuit. To provide continuous venovenous hemodialysis mode, the replacement fluids are removed and an appropriate dialysate is connected to the hemofilter. Both convection and diffusive therapies, such as CVVHDF, can also be provided.

Full access? Get Clinical Tree
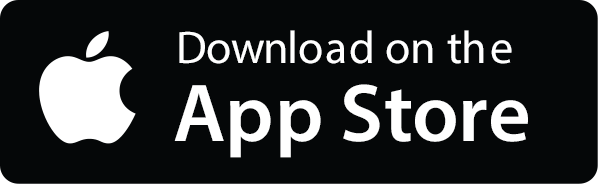
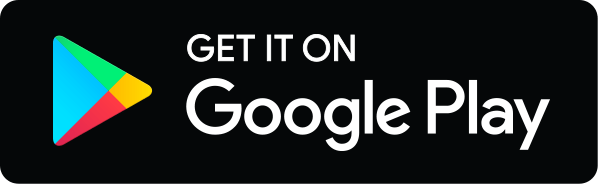