Acidosis is a common finding in many childhood disease states, ranging from self-resolving diarrheal illnesses to progressively degenerative mitochondrial disorders to acute septicemia and shock. Given the broad range of underlying illnesses and their disparate clinical consequences, identifying the specific etiology of acidosis is often crucial to a child’s hospital care. In circumstances where there is relative diagnostic certainty, appropriate identification and characterization of acidosis can contribute important clinical evidence for or against a prevailing diagnostic theory or management strategy. For patients whose illnesses have not been fully characterized, a rational approach to the interpretation of acidosis can help direct more specific diagnostic testing and further clinical evaluation.
In this chapter, we discuss the pathophysiology, differential diagnoses, and workup strategies for acidosis, focusing primarily on metabolic acidosis. We include a section on respiratory acidosis in Special Considerations.
Acidosis is a metabolic state characterized by a relative excess of hydrogen ions (H+). This relative excess can result from a number of processes: (1) an increase in H+ generation, (2) a decrease in H+ elimination via the kidneys, (3) a decrease in basic buffer molecules, or (4) a combination of the three. These processes reflect imbalances in the various counter-regulatory systems involved with acid–base homeostasis.
A growing child generates a net of 1–3 mEq/kg/day of acid through normal metabolic processes. To maintain the optimal conditions for enzymatic activity (a pH between 7.35 and 7.45), the body must efficiently eliminate this and any additional acid load. Three primary mechanisms are responsible for maintaining this balance: the blood buffer system, respiratory ventilation, and renal excretion. Immediately upon exposure to excess acid, circulating buffers, including bicarbonate (HCO3–), bind excess H+, diminishing its concentration in the extracellular space. The excess H+ that binds to bicarbonate forms the “volatile” carbonic acid, which can then dissociate into carbon dioxide (CO2) and water. Assuming sufficient H+ excess and effective pulmonary ventilation, excess CO2 is then removed from the circulation through the pulmonary capillaries and alveoli. In response to persistently diminished pH, peripheral chemoreceptors and central respiratory centers increase minute ventilation to augment this process. Lastly, the proximal tubules in the kidney respond to excess H+ by increasing bicarbonate reabsorption and ammonia synthesis, the latter of which assists with renal hydrogen ion elimination in the form of ammonium ions (NH4+).
Acidemia, defined as an arterial pH of less than 7.35, occurs when the body’s numerous compensatory mechanisms to address an acid load are overwhelmed or compromised. The compensatory mechanisms can be overwhelmed in the setting of excess “non-volatile” acid production, as occurs acutely in lactic acidosis, diabetic ketoacidosis (DKA), or more indolently in the organic acidopathies. The body’s buffer system can be compromised when there is a reduction in circulating bicarbonate from gastrointestinal losses (e.g. prolonged diarrheal illness) or renal losses (e.g. proximal renal tubular acidosis). Compromised ventilation from a number of etiologies diminishes the body’s ability to readily eliminate volatile acid in the form of CO2, leading to retained H+. Defects in the kidney’s ability to appropriately acidify urine, as occurs with renal tubular acidosis (RTA), comprise the last set of conditions that lead to imbalances of this intricate homeostatic system.
DEFINITIONS
Acidosis: A state of relative excess of hydrogen ions.
Acidemia: Arterial pH less than 7.35.
Metabolic Acidosis: An acute or chronic state resulting from excess non-volatile H+ production, decreased H+ elimination via the kidneys, or diminished buffering capacity.
Respiratory Acidosis: An acute or chronic state of excess H+ resulting from inadequate removal of volatile acid in the form of CO2 via the lungs.
Generally, the symptoms of acidosis are non-specific. History should be targeted toward identifying the source of the acidosis. Common features of metabolic acidosis are vomiting and tachypnea. Other symptoms may include fatigue, lethargy, seizures, and alterations in mental status. Children with diarrheal illness may have acidosis but this is not a symptom of the acidosis, rather the precipitant. Analogously, children with respiratory acidosis may present with decreased respiratory rate or respiratory failure, also conditions which led to the acidosis. History of ingestion is particularly important, as is history of medical conditions that may predispose the patient to acidosis. Among others, these include diabetes mellitus, prior diagnosis of metabolic disorder, seizure disorder on a ketogenic diet, and systemic lupus erythematosus (which can be associated with a distal RTA). A number of medications are also associated with acidosis, including acetazolamide and other medications that can inhibit carbonic anhydrase (i.e. some anti-epileptic medications) and amphotericin B or amiloride, that may mimic RTAs.
Physical examination findings for children with acidosis are similarly nonspecific. Signs of hypovolemia (tachycardia, hypotension, delayed capillary refill, etc.) and tachypnea are often present in children with a diarrheal cause of excess bicarbonate loss or in those with lactic acidosis or DKA. Children presenting with DKA may present with fruity smelling breath from increased acetone ventilation. Patients with severe acidosis may present with alterations in level of consciousness or obtundation.
The differential diagnosis for acidosis is broad. As with other clinical scenarios, a basic understanding of epidemiology helps to generate an ordered differential diagnosis by assessing the prior probability of a particular cause with respect to the individual characteristics, symptoms, and signs of the child before you. Etiologies such as diarrheal illness and lactic acidosis are most probable from the general population perspective. However, certain groups require special consideration, including toddlers, where ingestions are particularly common, and newborns, where undiagnosed inherited disorders of metabolism are more prevalent. While Bayesian logic helps to order the possible diagnostic options, a number of well-established and relatively formulaic strategies to characterize a child’s acidosis are valuable in guiding further evaluation. One such strategy is detailed in Figure 18-1.
The initial step in categorization is to determine whether acidemia (pH <7.35) is present. In the non-emergent, non-intensive care setting, serum bicarbonate concentration is often used as a proxy for acidemia. While low bicarbonate concentration is reflective of acidosis, this is not equivalent to acidemia, which is important when considering whether there is appropriate compensation or a mixed disorder. An important rule of thumb is that the body’s normal compensatory mechanisms will never overcome the primary process.
The second consideration is the primary source of the acidosis, which can usually be determined by assessing [HCO3–] and pCO2. [HCO3–] of <22 mEq/L in the setting of acidemia indicates a metabolic acidosis, whereas a pCO2 of >45 mmHg on an appropriately collected and processed arterial blood gas indicates a respiratory acidosis.
Upon identification of a metabolic acidosis, the third consideration is to determine whether there is an anion gap. Based on the concept of electrochemical neutrality, total serum cation concentration must equal total serum anion concentration, so in normal metabolic states a fixed number should be derived when subtracting the sum of commonly measured serum cations from the sum of commonly measured anions. The most frequently used equation for anion gap is:
While there is never a “real” anion gap, a calculated gap of >14 mEq/L provides the clinician with evidence of both the presence and quantity of unmeasured anions, although gaps of up to 16 mEq/L may be normal in newborns.1 These unmeasured anions are generated through the buffering action of HCO3– in the presence of excess acid, as depicted in the following equilibrium:
In this equilibrium, H+anion– represents the excess acid, such as keto-acids, lactic acid, and organic acids. Through this reaction, HCO3– is metabolized, and the anion salt of the excess acid remains and serves to maintain serum electrochemical neutrality. When HCO3– is metabolized at a greater rate than the acid salt is eliminated and new HCO3– generated, an unmeasured anion will be detectable when calculating the anion gap. Etiologies of anion gap and non–anion gap acidosis are presented in Table 18-1, and the diagnostic evaluation of these categories is discussed in the next section. Of note, the calculated anion gap may be inappropriately low in the presence of unmeasured cations, as with lithium toxicity and hypermagnesemia, or in the setting of hypoalbuminemia, which is a potent contributor to the normal anion gap. In the setting of low albumin, an adjusted AG can be calculated using the following formula:
A non–anion gap acidosis indicates either the loss of HCO3– through the kidney or gastrointestinal tract, with the subsequent retention of Cl–, or the addition of an acid, with the subsequent consumption of HCO3– and a rapid renal excretion of the accompanying anion and replacement with Cl–. In both cases, HCO3– is effectively replaced by Cl–, which maintains a normal anion gap. Because of this tendency for Cl– to replace HCO3–, non–anion gap acidosis is also known as hyperchloremic metabolic acidosis.
Anion Gap Acidosis |
Ketoacidosis |
Diabetic ketoacidosis |
Alcoholic ketoacidosis |
Starvation |
Lactic acidosis |
Tissue hypoxia |
Shock |
Dehydration |
Hypoxia |
Carbon monoxide poisoning |
Increased production or decreased clearance of lactate |
Leukemia, lymphoma, large tumors |
Liver or renal failure |
Renal failure (especially if GFR <15 mL/min/1.73 m2; uremia and lactic acidosis) |
Toxins or drugs |
High osmolal gap |
Ethylene glycol |
Ethanol |
Methanol |
Normal osmolal gap |
Salicylate |
Ibuprofen |
Toluene inhalation |
Isoniazid |
Iron |
Metformin |
Inborn errors of metabolism |
Non–Anion Gap Acidosis |
Gastrointestinal loss of HCO3– |
Diarrhea |
Enteric fistulas (e.g. pancreatic) or enterostomies |
Ureterosigmoidostomy (Cl–/HCO3– exchanger) |
Drugs (e.g. calcium chloride, cholestyramine, magnesium sulfate) |
Renal acidosis |
Loss of HCO3– |
Proximal RTA (type 2) |
Drugs (e.g. acetazolamide) |
Failure to excrete H+ |
Distal RTA (type I) |
Drugs (e.g. potassium-sparing diuretics, amphotericin B) |
Decreased ammoniagenesis |
Generalized distal nephron dysfunction (type 4 RTA) |
Early and moderate renal insufficiency (GFR 20–50 mL/min/1.73 m2) |
Acid load |
Hyperalimentation |
Dilutional |
Rapid saline administration |
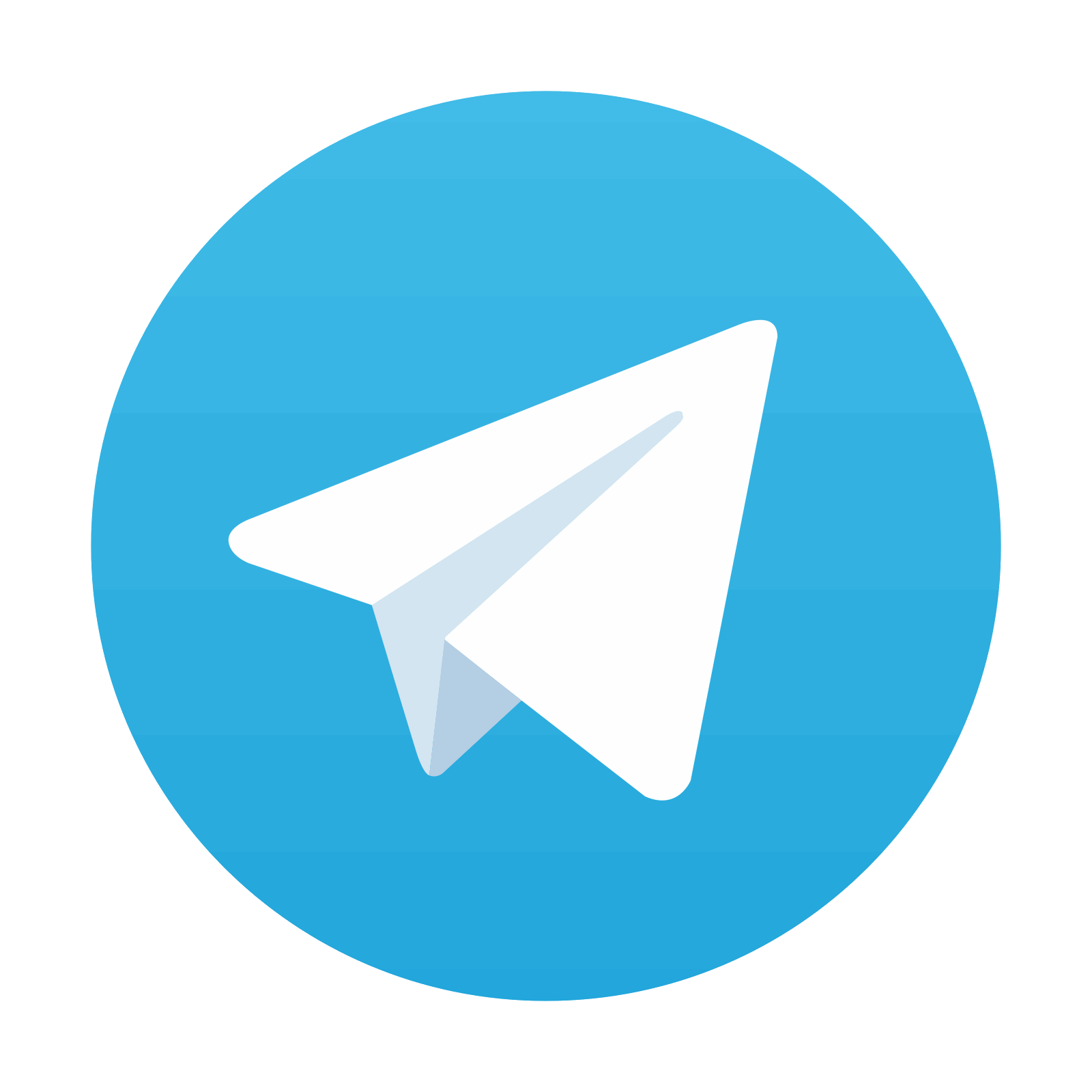
Stay updated, free articles. Join our Telegram channel

Full access? Get Clinical Tree
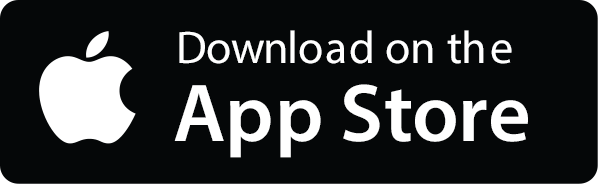
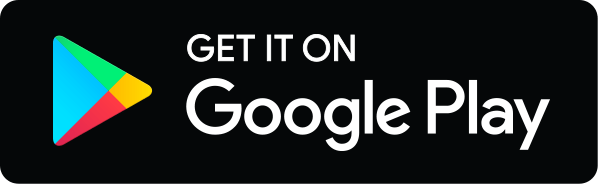
