Objective
The objective of the study was to characterize the vascular architecture at the placental-maternal interface in pregnancies complicated by placenta increta and normal pregnancies.
Study Design
Vessel numbers and cross-section area density and spatial and area distributions in 13 placenta-increta placental beds were compared with 9 normal placental beds using computer-assisted image analysis of whole-slide CD31 immunolabeled sections.
Results
The total areas occupied by vessels in normal and placenta-increta placental beds were comparable, but vessels were significantly sparser and larger in the latter. Moreover, placenta-increta–vessel distributions (area and distance from the placental–myometrial junction) were more heterogeneous.
Conclusion
Size and spatial organization of the placenta-increta vascular architecture at the placental-maternal interface differed from normal and might partially explain the severe hemorrhage observed during placenta-increta deliveries.
In accordance with the American Society of Maternal-Fetal Medicine recommendations, placenta accreta defines abnormally invasive placental implantation. This severe obstetrical complication, often responsible for life-threatening hemorrhage during delivery, affects 1 in 533 to 2510 deliveries. Its 10-fold increased incidence over the past 50 years has been associated with the rising cesarean section rate. Furthermore, the hysterectomy rate for accreta placentation increased by 23% between 1994 and 2007. A history of cesarean section remains the dominant maternal risk factor to develop placenta accreta and other complications in subsequent pregnancies. When placenta previa is present, the risk of placenta accreta increases from 3% for patients with a history of 1 cesarean section to 40-67% for those with 3 or more cesarean sections. A defect in the decidua, resulting in the direct adhesion of chorionic villi to the myometrium is suspected to be the main cause of placenta accreta.
We and others provided functional, echographic, and anatomical evidence of an extensive vascular anastomotic network in the subplacental myometrium during normal pregnancy. This network provides the placental intervillous blood supply and creates arteriovenous shunts in the subplacental myometrium. After delivery, passive uterine retraction and active myometrium contractions create a physiological tourniquet that stop the circulation in the terminal segments of this network, which open in the villous chamber, thereby preventing postpartum haemorrhage.
A precise definition of the vascular architecture at the placenta-maternal interface is lacking in women with placenta accreta. Only a few studies have characterized in vivo the uteroplacental vascularization for patients with placenta increta using 3-dimensional (3D) color power Doppler or 3D-angiography.
Because women with placenta accreta are at high risk of early postpartum hemorrhage, we analyzed in this study the vessel distribution in their placental bed in comparison with that of normal pregnancies. We combined high-resolution virtual imaging of whole-tissue sections and computer-assisted image analysis to avoid inter- and intraobserver variability of the conventional optical microscopy and hot spot analysis.
Materials and Methods
Tissue collection
Histopathologically, placenta accreta defines the direct attachment of placental villi to the myometrium. Depending on the depth of villous invasion into the myometrium (or beyond), 3 subtypes are differentiated: placenta accreta vera, increta, and percreta. In placenta accreta vera, chorionic villi attach directly to the myometrium in the absence of decidua. In placenta increta, placental villi invade deeper into the myometrium but do not extend to the outermost layer of the uterus. In placenta percreta, chorionic villi penetrate through the myometrium up to the serosa or beyond.
Placenta accreta vera is the histological description of a clinical entity, which often resolves by performing manual removal of a not spontaneously delivered placenta. The differential diagnosis with a retained placenta is very difficult for the clinician and often not in line with the pathological examination. Therefore, providing good quality samples of accreta vera placentation is challenging.
Therefore, we used hysterectomy specimens with the placenta in situ to reliably document a deep abnormal villous infiltration. Only areas in which myometrium was present were analyzed. These specimens were all classified as placenta increta independently by the 3 experienced pathologist involved in the study (1 from each center), using the criteria mentioned in the previous text. They were obtained from 13 patients with pregnancies complicated by clinically suspected and histologically confirmed placenta increta, who underwent cesarean hysterectomy.
Placental bed biopsies were obtained prospectively from 9 women presenting an uncomplicated pregnancy and delivered by elective cesarean section for breech presentation, fetopelvic disproportion or a history of 2 previous cesarean sections. All these biopsies were sampled under direct vision by the same operator (F.C.) after placental delivery from the central zone of the placental site applying the previously described knife technique. Adequacy of the samples was controlled by microscopy of haematoxylin-eosin–stained sections.
Thirteen paraffin-embedded tissues of increta cases were retrospectively obtained from 2 obstetrics and gynecology departments (J. J. Naon Morphological Institute, School of Medicine, University of Buenos Aires, Buenos Aires, Argentina, 7 cases; and Charité Campus Virchow, University Hospital, Berlin, Germany, 6 cases). Control samples (9 cases) were collected prospectively at the third Department of Obstetrics and Gynecology (University of Liege, Liege, Belgium). Here the local Ethics Committee approved also the study and patients’ informed consent was obtained.
Histology and immunohistochemistry
All biopsies were fixed in 4% formaldehyde in phosphate-buffered saline (PBS) and embedded in paraffin. Immunohistochemistry for cytokeratin-7 (CK7; an epithelial marker expressed by trophoblast cells), insulin-like growth factor-binding protein-1 (IGFBP-1; a marker for decidualized stromal cells), and CD31 (a vascular endothelial cell marker) was performed on paraffin sections that were 4 μm thick mounted on aminopropyltriethoxysilane (Tespa)-coated glass slides. The sections were dewaxed in xylene and rehydrated. Antigen retrieval was achieved with Target Retrieval Solution (S2031; DakoCytomation, Glostrup, Denmark).
Endogenous peroxidases were blocked by incubation in 3% H 2 O 2 for 20 minutes, and nonspecific binding was prevented by incubation in PBS/normal goat serum. The following primary mouse antibodies and dilutions were used: monoclonal antihuman CK7 (M7018, 1:50; DakoCytomation), monoclonal antihuman IGFBP-1 (6303, 1:750; Medix Biochemica, Kaunieainen, Finland), or monoclonal anti-CD31 (M0823, 1:40; DakoCytomation). Sections were incubated with primary antibodies for 60 minutes at 37°C and then biotinylated goat antimouse IgG (E0433, 1:400; DakoCytomation), followed by streptavidin/horseradish peroxidase (P0397, 1:500; DakoCytomation). Labeling was visualized with 3-3′-diaminobenzidine hydrochloride (K3468; DakoCytomation) as the chromogen and hematoxylin as the counterstain. Nonspecific binding of primary antibody was evaluated using mouse IgG1 at the same immunoglobulin concentration than the primary antibody (X0931; DakoCytomation).
Image acquisition, processing, and measurements
Virtual images of the whole tissue sections were acquired with the fully automated digital microscopy system dotSlide (BX51TF; Olympus, Aartselaar, Belgium) coupled with a Peltier-cooled, high-resolution digital color camera (XC10; Olympus) at a resolution of 1376 × 1032 pixels. Whole-tissue sections at high magnification (×100) were scanned, yielding virtual images with a 0.65 μm pixel size.
The vessel parameters were characterized in the myometrium up to a depth of 2.25 mm from the placental-myometrial junction. This value represents the maximal depth that can be measured in all tissue samples.
Binary images were decimated before quantification, according to a previously described procedure because the original virtual images exceed several gigabytes.
CD31-indentified endothelial cells allowed an automatic computerized acquisition of blood vessels characteristics. Vessel area density was defined as the number of pixels belonging to all vessels (endothelium and encompassed lumen, ie, the total area occupied by vessels) divided by the total number of pixels of the 2.25 mm myometrial depth. The number of vessel cross-sections per unit area was defined as the number of vessels divided by the area of myometrium. The area of each vessel cross-section was measured.
The vessel cross-section area distribution was drawn as a histogram. The Euclidean distance from the center of gravity (CG) of each vessel to the (manually drawn) placental-myometrial junction was measured using a previsouly described methodology. Distance distribution was drawn as a histogram. Finally, vessel sizes were plotted as a function of their distance to the placental-myometrial junction. Image processing and measurements used MATLAB version 9.2 software (MathWorks, Natick, MA).
Statistical analysis
Results are expressed as means ± SEM of individually tested parameters (vessel area density, number of vessels/unit area, area of individual vessel sections, vessel cross-section area distribution, and distance from each vessel to the placental-myometrial junction). For each parameter, a large number of measurements were performed in each individual (mean number of vessels per tissue section 2242 for increta and 3185 for control cases). The total vessel count numbers are 25074 for the increta group and 28662 for the control group.
Tests of homogeneity performed in each group (increta and control) confirmed the homogeneity of both populations for the evaluated parameters. It can therefore be concluded that for the tested parameters, the increta, and the control group displayed homogenous characteristics. The statistical differences between the groups were assessed using the nonparametric Mann-Whitney U test. Furthermore, we used the nonparametric Kolmogorov-Smirnov test for distributions and analysis of covariance for linear regressions. P < .05 defined significance. Statistical analyses were performed with MATLAB version 9.2 software.
Results
The demographic and obstetrical characteristics of the increta and control groups are summarized in the Table . As expected, women with placenta increta were significantly older, had more cesarean sections, and delivered at earlier gestational age. We verified that no biopsy was sampled in the uterine scar of a previous hysterotomy. It may therefore be anticipated that the demographic and obstetrical differences would not affect vessels distribution in the placental bed.
Variable | Increta (n = 13) | Controls (n = 9) | P value |
---|---|---|---|
Maternal age at delivery, y a | 37 (30–42) | 24 (20–39) | .0029 |
Gestational ages at delivery, wks a | 34 (30–38) | 39 (38–39) | .0021 |
Gravidity a | 5 (3–6) | 3 (1–8) | .0121 |
Parity a | 3 (1–4) | 2 (0–7) | NS |
Number of prior cesarean sections, n | |||
0 | 1 | 4 | |
1 | 5 | 0 | |
≥2 | 7 | 5 | |
Number of prior curettages, n | |||
0 | 4 | 9 | |
1 | 5 | 0 | |
≥2 | 4 | 0 |
a Data presented are expressed in medians (interquartile range) and analyzed with the Mann–Whitney U test.
Figure 1 illustrates the strategy used to correctly orientate placental bed biopsies. Serial sections from both groups were labeled with anti-CK7 antibodies to identify trophoblast cells and anti-IGFBP-1 antibodies to identify decidualized stromal cells. The vascular architecture was studied after CD31 immunolabeling because the detection of this specific marker provides the optimal contrast between the vessels and background tissue. Indeed, in digitized color (red, green, blue) images, a brownish color distinguishes CD31-labeled blood vessels. The placental-maternal tissue interface is easily identified and manually drawn.

Figure 2 illustrates the computer-assisted–image algorithm for a control and an increta case. On the whole-slide section, most vessels were automatically detected, whereas all missed vessels, the placental-myometrial junction, and the limits of tissue section were manually drawn ( Figure 2 , B and E). Finally, Figure 2 , C and F, shows binarized images, subsequently used for vascular architecture characterization.

Placental bed-vessel characteristics
First, we determined the number and area of blood vessels. Their respective total cross-sectional vessel areas did not differ (increta 14% ± 2 vs control 12% ± 2, P = .48; and Figure 3 , A). The mean number of vessels per tissue section was 2242 for increta and 3185 for control cases, respectively. However, the increta group had fewer vessels (30.84 ± 2.75 vessels/mm 2 vs 76.77 ± 14.28 vessels/mm 2 , P = .008; Figure 3 , B), implying that placenta increta vessels are larger than in normal placentas. Figure 2 allows a simple visual comparison between a typical increta case and a control; the placenta increta vessels are 1.5 times larger, as confirmed by computation (respectively, 0.0042 ± 0.0006 mm 2 vs 0.0028 ± 0.0006 mm 2 , Figure 3 , C).

Vessel cross-section areas varied widely between 1 μm 2 and 3 mm 2 . The log 10 -transformed vessel-size distribution ( Figure 3 , D) was analyzed. This normalized histogram mainly highlights the excess of smaller vessels in increta placentas but masks the presence of larger vessels in them, which contributes to the higher mean vessel area in these patients ( Figure 3 C).
Therefore, the bin-by-bin difference between the increta and control histograms was calculated ( Figure 3 , D, inset ). In this graph, positive values show the excess of increta vessels versus controls. Vessels less than 0.0003 mm 2 and greater than 0.06 mm 2 were more abundant in the increta placentas, whereas the intermediate-size vessels were more plentiful in the controls.
Spatial distribution of vessels
Visual examination of whole-slide sections suggested a difference in vessel spatial distributions ( Figure 2 , A and D), which seems more obvious after adding each vessel’s CG ( Figure 2 , C and F). Quantification of this observation, by measuring the distance between each vessel’s CG and the placental-myometrial junction, showed more placenta-increta vessels closer to that junction ( Figure 4 ). Most placenta-increta vessels were located less than 0.20 mm from this interface, thereby accounting for the more uneven vessel-size distribution in them ( P KS = .01).

Full access? Get Clinical Tree
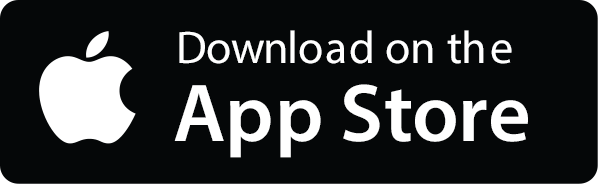
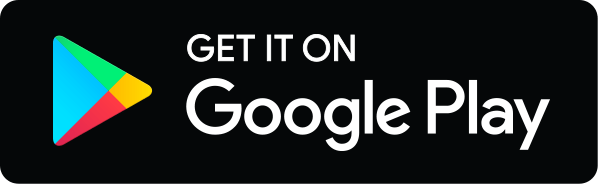