- •
The incidence of bronchopulmonary dysplasia (BPD) remains high, but the nature of the disease has evolved from the traditional form marked by extensive fibroproliferative changes to one of alveolar simplification and less scarring.
- •
There is a wide spectrum of severity of BPD; thus better definition of specific phenotypes is needed.
- •
BPD is associated with multiple co-morbidities, including poor growth, metabolic bone disease, aerodigestive disorders, pulmonary hypertension and neurodevelopmental impairment.
- •
The complex needs of these infants are quite different from those of the typical preterm infant are best served by a multidisciplinary team approach focused on BPD care.
- •
Many current therapies for BPD lack a solid evidence base; more research is urgently needed.
Bronchopulmonary dysplasia (BPD), the chronic lung disease of infancy that follows preterm birth, was first characterized by Northway and colleagues 50 years ago. In that era, prematurity-associated lung disease contributed to high mortality (60%) in relatively late-gestation preterm infants by today’s standards (32–34 weeks’ gestation). Currently survival for these late preterm infants is nearly 100%, with a 94% survival of preterm infants born even at 28 weeks. This remarkable success of modern care has increased survival of even the most extremely low gestational age newborns at the limits of viability (currently 22–23 weeks’ gestation), which likely accounts for the persistent rate of BPD at 43% of preterm infants born before 29 weeks’ gestation. As a result, BPD remains the most common morbidity of preterm birth, occurring in an estimated 10,000 to 15,000 infants per year in the United States alone. This has important health care implications as infants with BPD require prolonged neonatal intensive care unit (NICU) hospitalizations; are frequently readmitted during the first 2 years for respiratory infections, asthma, and related problems; and often have persistent lung function abnormalities and exercise intolerance as adolescents and young adults.
Although the overall incidence of BPD has not declined over the past decade, the nature of the disease and number of infants with severe BPD (sBPD) have changed with current clinical practice. Infants with chronic lung disease after premature birth have a different clinical course and pathology from that traditionally observed in infants dying with BPD during the pre-surfactant era. The classic progressive stages of disease, including prominent fibroproliferative changes, that first characterized BPD are often absent now, and the disease has changed to being predominantly defined as a disruption of distal lung growth, referred to as “the new BPD.” The new BPD often develops in preterm newborns who may have required minimal or even no ventilator support and relatively low inspired oxygen concentrations during the early postnatal days.
Although improved care has generally led to milder respiratory courses, infants with BPD can still develop severe lung disease, as reflected by chronic respiratory failure with high mortality and related morbidities ( Fig. 22.1 ). Ongoing clinical care and research has largely focused on issues regarding the prevention of BPD in preterm infants; however, strategies to improve care and outcomes of preterm infants in whom particularly severe disease develops remain a major challenge. The management of infants with sBPD has received less attention regarding clinical studies and interventions compared with preventive strategies, yet these infants constitute a critical population who remain at high risk for extensive morbidities and late mortality.

Therefore, the goal of this chapter is to characterize the epidemiology, pulmonary, and cardiovascular pathophysiology of sBPD, especially in ventilator-dependent infants, and to discuss therapeutic strategies for their management as based on the underlying physiology.
Definitions and Scope of Severe BPD
BPD is typically defined by the presence of chronic respiratory signs, a persistent requirement for supplemental oxygen, and an abnormal chest radiograph at 1 month of age or at 36 weeks postmenstrual age (PMA) in patients born before 32 weeks’ gestation. This definition lacks specificity and fails to account for important clinical distinctions related to the extremes of prematurity and wide variability in criteria for the use of prolonged oxygen therapy. The need for supplemental oxygen at 1 month in infants born at 24 or 25 weeks’ gestation may represent lung or respiratory control immaturity and not reflect the results of “lung injury,” and chronic respiratory disease may or may not develop in such infants. A National Institutes of Health (NIH)-sponsored conference in 2000 led to the current widely used definition of BPD that categorizes the severity of BPD according to the level of respiratory support required at 36 weeks PMA. An advantage of this classification is that BPD is defined as a spectrum of disease with early markers that may be predictive of long-term pulmonary morbidity. Past studies suggest that this grading of BPD severity is associated with the degree of abnormal lung function during infancy ; however, recent studies suggest that antenatal factors are key determinants for late respiratory disease independent of the diagnosis of BPD.
Another approach to determine the severity of BPD is to assess chest radiographs, but for many infants with chronic supplemental oxygen dependency, the chest radiograph demonstrates only small volumes with hazy lung fields. Various scoring systems have been developed and may predict chronic oxygen dependency and troublesome respiratory symptoms at follow-up. Further work is clearly needed to identify early physiologic, structural, and genetic or biochemical markers of BPD, which are predictive of critical long-term endpoints, such as the presence of late respiratory disease evidenced by prolonged mechanical ventilation and oxygen therapy, recurrent hospitalizations, reactive airways disease, respiratory medications, and/or exercise intolerance during childhood.
The current NIH classification system defines “severe” BPD as the need for supplemental oxygen above a fraction of inspired oxygen (F io 2 ) of 0.30 with or without positive-pressure ventilation and/or continuous positive airway pressure (CPAP) at 36 weeks PMA in patients born before 32 weeks’ gestation. Currently data on the proportion of infants who meet these criteria are relatively limited. In addition, early respiratory deaths after the first week of life but before 36 weeks PMA may represent the most severe form of BPD, yet are not included in databases because they fail to reach the standard endpoint.
In addition, some of the most severely affected infants are those who require sustained mechanical ventilation. Although they are included in the severe classification of BPD according to NIH definitions, the proportion of infants with the most severe disease requiring high levels of respiratory support is uncertain and optimal approaches to their care are incompletely understood. This is an important issue as mortality increases with the duration of mechanical ventilation. Thus a subgroup of infants with sBPD exists that can be defined by a sustained need for mechanical ventilator support at 36 weeks PMA, which has recently been labeled as “type 2 sBPD” to differentiate infants with especially severe lung disease.
The limited incidence data for sBPD suggest that 16% to 30% of extremely low-birth-weight infants have sBPD. The original validation study of the NIH consensus definition of BPD found that 16% of infants born before 32 weeks and weighing less than 1000 g had sBPD ( Table 22.1 ). Two reports using birth cohorts from Scandinavia found that the incidence of sBPD was 20% to 25% in preterm infants born at or before 28 weeks. In 2013 the Children’s Hospitals Neonatal Consortium (CHNC) reported a 16% incidence of sBPD in patients born before 32 weeks, of whom the 91% who survived to discharge, 66% were discharged using supplemental oxygen, 4% required mechanical ventilation, and 5% received tracheostomy. The BPD Collaborative, a network of sites with multidisciplinary care programs for sBPD, recently reported a point prevalence of sBPD of 37%, ranging from 11% to 58% across centers. This same group reported that 41% of the patients born before 32 weeks’ gestation with sBPD had a persistent need for ventilator support at 36 weeks PMA, or type 2 sBPD. More recently the National Heart, Lung, and Blood Institute Prematurity and Respiratory Outcomes Program (PROP), involving a cohort of 724 infants born before 29 weeks’ gestational age from 6 academic U.S. centers, reported an incidence of sBPD of 30.6%; 37% of those followed through the first year had persistent respiratory disease.
Early (prevention) | Strategies to prevent acute lung injury:
|
Late (established BPD) | Strategies for effective gas exchange:
|
Few studies have examined the proportion of infants with type 2 sBPD who continue with high levels of respiratory support at and after 36 weeks PMA. In patients born before 27 weeks’ gestation admitted to a level IV NICU caring exclusively for outborn infants, 22% required mechanical ventilation at 36 weeks PMA and 16% had a tracheostomy. The variables associated with the need for prolonged mechanical ventilation have not been extensively examined, but in this cohort patients who required ventilation at 36 weeks were of lower birth weight and were more likely to need dopamine or insulin and to have had a fungal infection, but there was no difference in gestational age, Apgar scores at 1 and 5 minutes, age at NICU admission, indomethacin prophylaxis and/or treatment, need for patent ductus arteriosus ligation, intraventricular hemorrhage, severe intraventricular hemorrhage, or necrotizing enterocolitis between patients treated with intermittent positive-pressure ventilation at 36 weeks and those not requiring intermittent positive-pressure ventilation at 36 weeks. Thus the smaller and sicker extremely premature infants are at the highest risk for developing type 2 sBPD.
Using an estimated incidence of sBPD of 16% for infants born before 32 weeks suggests that sBPD develops in approximately 13,000 patients annually in the United States alone. Epidemiologic data are limited, but estimates suggest that roughly 8000 children in the United States receive mechanical ventilation at home.
An optimal ventilation mode has not yet emerged to prevent BPD, but it is clear from physiologic studies that tidal volumes and inspired oxygen concentrations should be reduced as low as possible to avoid hypocarbia, volutrauma, and oxygen toxicity, while applying strategies to optimize lung recruitment. Two meta-analyses suggest that volume-targeted ventilation reduces the duration of mechanical ventilation and significantly reduces the incidence of BPD. An alternative approach to reduce the risk of developing BPD has been to avoid intubation and mechanical ventilation by using early nCPAP. For example the Surfactant, Positive Pressure, and Oxygenation Randomized Trial (SUPPORT) found that patients who received early CPAP without intubation and surfactant therapy had decreased need for intubation or postnatal corticosteroids for BPD, required fewer days of mechanical ventilation, and were more likely to be alive and free from the need for mechanical ventilation by 7 days of age. Many centers now minimize their use of mechanical ventilation, preferring nCPAP with or without administration of exogenous surfactant, and report low incidences of BPD in high-risk infants. Because the risk for BPD is associated with the need for mechanical ventilation and centers that use less mechanical ventilation have a lower incidence of BPD, avoiding or minimizing mechanical ventilation during the early course of extreme prematurity may prevent BPD or lessen its severity. As discussed later, however, ventilator strategies during the early stages of respiratory distress are strikingly different from approaches needed to optimize gas exchange and treat chronic respiratory failure in the setting of established BPD.
Pathophysiology of Severe BPD
Respiratory Function
Multiple abnormalities of lung structure and function contribute to late respiratory disease in BPD. Chronic respiratory signs in children with moderate and severe BPD include tachypnea with shallow breathing, retractions, and paradoxical breathing pattern; coarse rhonchi, rales, and wheezes are typically heard on auscultation. The increased respiratory rate and shallow breathing increase dead-space ventilation. Nonuniform damage to the airways and distal lungs results in variable time constants for different areas of the lungs, and inspired gas may be distributed to relatively poorly perfused lung, thereby worsening ventilation-perfusion matching. Decreased lung compliance appears to correlate strongly with morphologic and radiographic changes in the lung. Dynamic lung compliance is markedly reduced in infants with established BPD, even in those who no longer require oxygen therapy. The reduction in dynamic compliance is due to small airway narrowing, interstitial fibrosis, edema, and atelectasis.
Newer mechanical ventilation strategies have resulted in less central airway damage in infants with the new BPD, but significant tracheomalacia and abnormalities of conducting airway structure persist in the current era. Increases in airway smooth muscle have been found within the first month of life in infants with BPD, and epithelial cell height was found to be greater than in controls. The combination of smooth muscle hypertrophy and thickened airway walls, together with fewer alveolar wall attachments supporting small airway patency, predispose BPD infants to increased airway resistance, which can be demonstrated even during the first week after birth in preterm neonates at risk for BPD. Although there is not complete agreement among various studies, most have demonstrated that pulmonary or respiratory system resistance is elevated within the first 2 weeks in those ventilator-dependent infants in whom BPD subsequently develops compared with those in whom BPD does not develop. This abnormality in lung mechanics persists: older infants with BPD have been found to have an increased total respiratory and expiratory resistance with severe flow limitation, especially at low lung volumes.
The presence of tracheobronchomalacia may also result in airflow limitation. It is important for the clinician to recognize this entity, because the airflow limitation is worsened by bronchodilator therapy. The presence of tracheobronchomalacia in BPD infants has been associated with longer courses of mechanical ventilation, longer NICU stays, and more complicated NICU courses. In the early stages the functional lung volume is often reduced as the result of atelectasis, but during the later stages of BPD there is gas trapping with hyperinflation. The use of pulmonary function testing to follow the progression of BPD and the response to therapeutic interventions has increased but is still not commonly applied in the clinical setting owing to the technical challenges, expense, and need for sedation.
Although the new BPD has been characterized as an arrest of distal lung and vascular growth, most of these observations were based on lung histology and evidence was lacking that provided direct physiologic data to support this finding. Balinotti and colleagues have demonstrated reduced lung surface area in infants with BPD by using novel methods of assessing diffusion capacity. Thus established BPD is primarily characterized by reduced surface area and heterogeneous lung units, in which regional variations in airway resistance and tissue compliance lead to highly variable time constants throughout the lung. As a result, mechanical ventilation of infants with sBPD requires strikingly different ventilator strategies from those commonly used early in infants with RDS to prevent BPD. Strategies for severe BPD generally favor longer inspiratory times, larger tidal volumes, higher positive end-expiratory pressure (PEEP), and lower rates to allow more effective gas exchange and respiratory function.
Lung Mechanics in Severe BPD
Diverse methods have been used to assess lung mechanics in infants with established BPD during tidal breathing. These include measurements of dynamic resistance and compliance of the lung with the use of esophageal pressure catheters; single- and multiple-breath occlusion for measuring respiratory system resistance and compliance; plethysmography measurement of airway resistance; interrupter and forced oscillation methods for measuring respiratory system resistance; and weighted spirometry. Airway obstruction has also been determined from respiratory inductive plethysmography measurements of phase-angle differences in chest wall and abdominal dimensions. These approaches have been reviewed in detail. Normalized measures of both static and dynamic lung compliance are reduced in established BPD. This may be due to fibrosis, atelectasis, changes in parenchyma and airway properties, or diminished coupling between lung parenchyma and airways owing to edema.
Past studies have reported increased airway resistance in infants with established BPD. Specific compliance and conductance generally improve over the first 2 to 3 years of life. However, concerns persist regarding limitations of infant pulmonary function testing, especially for routine clinical use. Measures of compliance and resistance can be variable as these values are generally determined over a limited tidal volume range and are dependent on the lung volume at which these measurements are made; thus hyperinflation will be associated with lower lung or respiratory system compliance. Additionally, in BPD patients with airway obstruction, measurements of dynamic compliance are markedly affected by respiratory rate (e.g., “frequency dependence”) because of the heterogeneity of time constants of regional lung units. This heterogeneity is reflected in the substantial curvilinearity of the passive expiratory flow-volume curve obtained by the single-breath occlusion method in infants with BPD. Jarriel et al. have pointed out that respiratory system mechanics in these patients are much better characterized by a “two-compartment” rather than a linear “one-compartment” model (see below). The abnormalities in compliance and especially resistance in BPD infants significantly alter how the lung fills and empties; the respiratory system time constant of 24 BPD infants mechanically ventilated for 38 ± 4 days increased from 0.14 ± 0.01 seconds at 10 to 20 days of life to 0.33 ± 0.02 seconds at 6 months, 0.48 ± 0.03 seconds at 1 year, and 0.50 ± 0.03 seconds at 2 years ( p < .0001).
Lung Volumes in Severe BPD
FRC has been measured in infants with BPD by body plethysmography and with nitrogen washout and gas dilution methods. In contrast to plethysmography, washout and gas dilution methods measure only gas that communicates with the conducting airways during tidal breathing. These measurements may underestimate the actual lung volume at FRC because they do not measure volumes of gas trapped behind closed airways and can underestimate volumes in severely obstructed poorly ventilated areas. Measurements before 1 year of age using gas dilution and washout methods have consistently reported reduced FRC in infants with both old and new BPD. In contrast, plethysmography studies have demonstrated normal or elevated FRC values. Reductions in gas dilution and nitrogen washout measurements of FRC probably reflect the amount of noncommunicating trapped gas not measured in infants with obstructive disease rather than being indicative of a true restrictive defect. Reduction in the difference between the two methods is probably indicative of improvements in airway function, less gas trapping, and better gas exchange. Thus functional abnormalities in infants with severe BPD are primarily obstructive rather than restrictive, but precise measurements are especially complicated in severe disease because of heterogeneity of airway and lung parenchymal abnormalities.
Recently the use of the raised volume rapid thoracic compression method for performing spirometry in sedated infants has provided an alternative approach to measure fractional lung volumes, including total lung capacity (TLC) and residual volume (RV). Robin et al. have reported the results of fractional lung volume measurements in 28 patients with new BPD. Mean RV and RV/TLC ratio were found to be significantly elevated in infants with BPD compared with normal control infants while mean TLC was in the normal range. In contrast, FRC as measured by plethysmography was found to be only marginally elevated compared to the normal control infants. In addition, TLC continued to increase over the second year of life in infants with BPD, yet the severity of air trapping, as reflected by the RV/TLC ratio, remained unchanged. Thus infants with the new BPD have obstructive airway disease with gas trapping that persists over time and is strikingly abnormal in severe BPD.
Forced Flows in Severe BPD
Measurements of forced flows have been made in infants with BPD using the rapid thoracic compression (RTC) method to produce partial flow-volume curves over the tidal range and the forced deflation and raised volume rapid thoracic compression techniques to produce forced expiratory flows over the full vital capacity range. The use of these tests in infants with BPD has been reviewed and guidelines for the two RTC methods have been published. The RTC technique to produce partial expiratory flow volume curves was applied to infants with BPD, demonstrating that average maximal flows measured at FRC (V′max FRC ) were reduced by approximately 50% when compared to normal infants. A reduction in V′max FRC in infants with BPD has been a consistent finding in subsequent studies, and longitudinal measurements over the first 2 years of life demonstrated very modest increases in absolute flows in individual infants with BPD who often do not keep pace with the expected rate of increase with growth. On average, the rate of increase for infants with BPD was substantially below that measured in normal infants over the same interval. Thus at follow-up, measurements of V′max FRC in the infants with BPD had fallen even farther below those measured in normal infants. Reduction in forced flow can also reflect severity of the underlying disease: toddlers with BPD still dependent on supplemental oxygen use after 2 years of age had significantly lower volume-corrected forced flows (V′max FRC/FRC ) than those BPD toddlers who were weaned from supplemental oxygen.
Lung Imaging in Severe BPD
The chest radiographic characteristics of infants with BPD have changed substantially since the original description by Northway et al. Although the chest radiograph of BPD as classically described is still seen in infants with severe disease, radiographic changes in smaller, less mature infants with new BPD are much more variable and are often characterized by irregularly distributed areas of fine infiltrates and mild hyperlucency. Chest radiographs often underestimate and correlate poorly with the extent of the pathologic changes in infants with established BPD. High-resolution computed tomography (HRCT) is a more sensitive technique for detecting structural abnormalities in the lungs of patients with established BPD than plain chest radiography ( Fig. 22.2 ). Correlations between abnormalities seen on HRCT and measures of lung function and clinical severity suggest that HRCT may be useful in clinical management and as an outcome measure in this population. CT imaging is helpful for identifying unsuspected abnormalities in the lungs of individual patients with BPD, but its ultimate utility as a tool for clinical management and as an outcome measure for research investigations is not yet clear. Radiation exposure from CT imaging is substantially greater than that received from standard chest radiographs. Through the development of novel scanning algorithms, however, the radiation exposure from CT imaging has been greatly reduced, such that in cystic fibrosis diagnostic HRCT can be done at a radiation dose similar to that of a chest radiograph, and similar algorithm development is ongoing for patients with BPD.

The Cardiovascular System in Severe BPD
Acute lung injury impairs growth, structure, and function of the developing pulmonary circulation after premature birth. Endothelial cells are particularly susceptible to oxidant injury owing to hyperoxia or inflammation. The media of small pulmonary arteries may also undergo striking changes, including smooth muscle cell proliferation, precocious maturation of immature pericytes into mature smooth muscle cells, and incorporation of fibroblasts into the vessel wall and surrounding adventitia. Structural changes in the lung vasculature contribute to high pulmonary vascular resistance (PVR) caused by narrowing of the vessel diameter and decreased vascular compliance. Decreased angiogenesis may limit vascular surface area, causing further elevations of PVR, especially in response to high cardiac output with exercise or stress. The pulmonary circulation in BPD patients is further characterized by abnormal vasoreactivity, which also increases PVR. Abnormal pulmonary vasoreactivity is evidenced by a marked vasoconstrictor response to acute hypoxia. Cardiac catheterization studies have shown that even mild hypoxia causes marked elevations in pulmonary artery pressure, even in infants with modest basal levels of pulmonary hypertension (PH). Maintaining oxygen saturation levels above 92% to 94% effectively lowers the pulmonary artery pressure. Strategies to lower pulmonary artery pressure or to minimize lung injury to the pulmonary vasculature may limit the subsequent development of PH in BPD.
Early injury to the lung circulation leads to the rapid development of PH, which contributes significantly to the morbidity and mortality of severe BPD. Even in early reports of BPD, PH, and cor pulmonale were recognized as being associated with high mortality. Persistent echocardiographic evidence of PH beyond the first few months has been associated with up to 40% mortality in infants with BPD. High mortality rates have also been reported in infants with BPD and severe PH, especially in those who require prolonged ventilator support. In addition to the adverse effects of PH on the clinical course of infants with BPD, the lung circulation is further characterized by persistence of abnormal or “dysmorphic” growth of the pulmonary circulation, including a relative paucity of small pulmonary arteries with an altered pattern of distribution within the interstitium of the distal lung. In infants with severe BPD, decreased vascular growth occurs in conjunction with marked reductions in alveoli, suggesting that the new BPD is primarily characterized by growth arrest of the developing lung. This reduction of alveolar-capillary surface area impairs gas exchange—thereby increasing the need for prolonged supplemental oxygen and ventilator therapy, causing marked hypoxemia with acute respiratory infections and late exercise intolerance—and further increases the risk for developing severe PH. Experimental studies have further shown that early injury to the developing lung can impair angiogenesis, which further contributes to decreased alveolarization and simplification of distal lung air space (the “vascular hypothesis” ). Thus abnormalities of the lung circulation in BPD are not only related to the presence or absence of PH, but more broadly, pulmonary vascular disease after premature birth as manifested by decreased vascular growth and structure also contributes to the pathogenesis and abnormal cardiopulmonary physiology of BPD.
A recently emerging cause of PH in patients with BPD is pulmonary vein stenosis (PVS). Mahgoub et al.
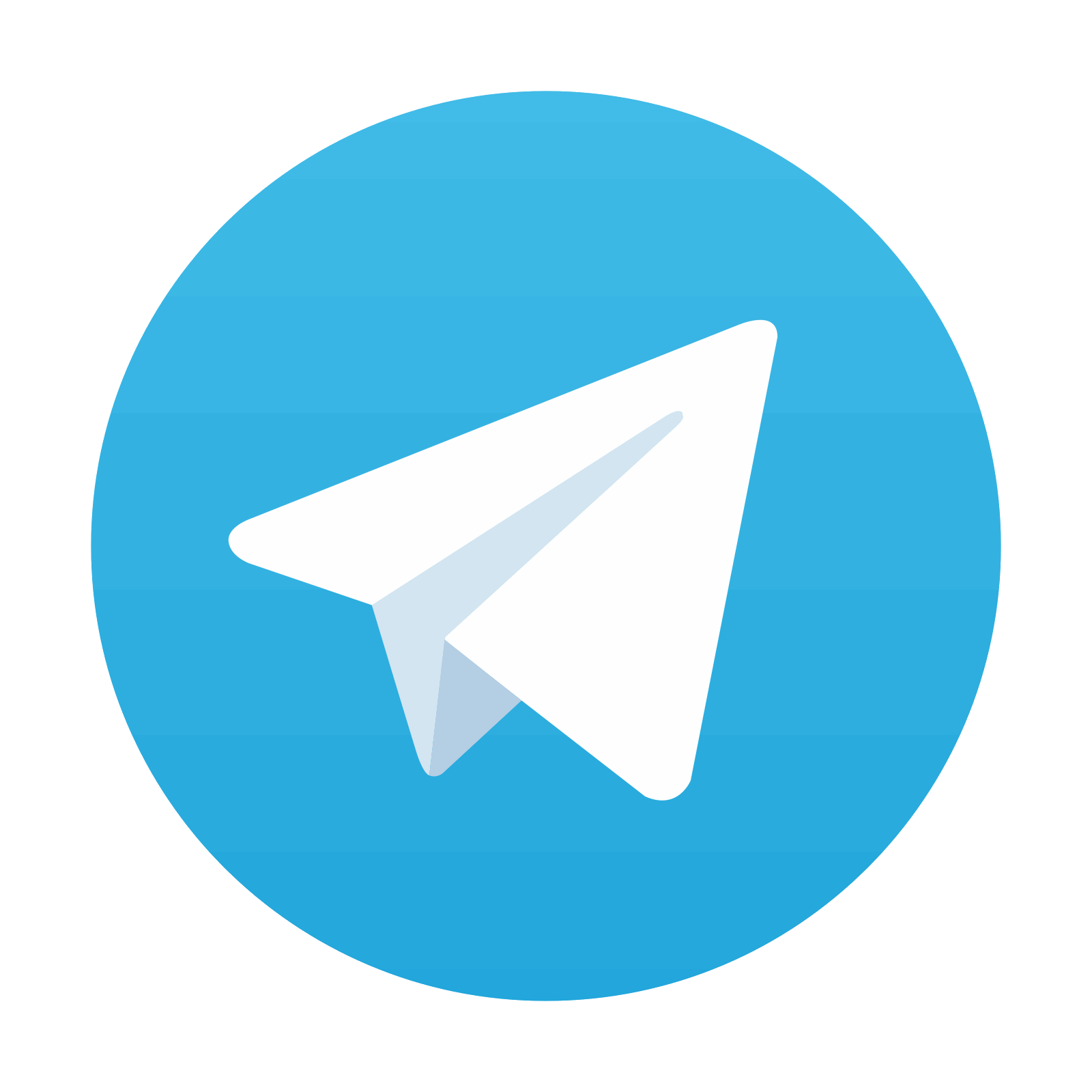
Stay updated, free articles. Join our Telegram channel

Full access? Get Clinical Tree
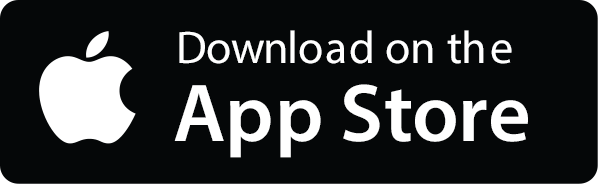
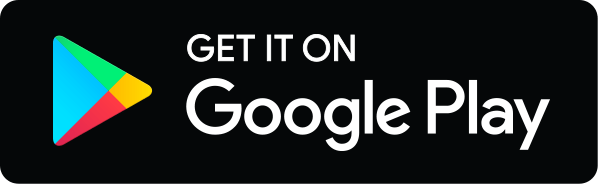