CHAPTER 43 Katherine R. Goetzinger1 and Anthony O. Odibo2 1Department of Obstetrics, Gynecology and Reproductive Sciences, University of Maryland School of Medicine, Baltimore, MD, USA 2Department of Obstetrics & Gynecology, University of South Florida, Tampa, FL, USA Disorders of fetal growth are commonly encountered in obstetrical practice. Although clinical estimation of fetal weight through symphysis‐fundal height measurements may provide the first sign of aberrant fetal growth, ultrasound estimation of fetal weight remains the gold standard for diagnosing fetal growth disorders in developed countries. Ultrasound estimation of fetal weight is typically derived using polynomial equations which combine measurements of biparietal diameter, head circumference, abdominal circumference, and femur length. These estimates of fetal weight are then plotted on population‐based growth curves by gestational age. Despite quality improvements in sonography over the years, there still remains a 6–15% margin of error in the sonographic prediction of fetal weight [1–3]. FGR has traditionally been defined as an EFW <10th percentile for gestational age; however, not all fetuses <10th percentile are pathologically small. In an attempt to identify fetuses who are pathologically growth‐restricted, others have proposed definitions such as EFW <5th percentile, EFW<3rd percentile, EFW two standard deviations below the mean for gestational age, abdominal circumference <10th percentile for gestational age and EFW <10th percentile with Doppler abnormalities. While these more stringent definitions may have high positive predictive values, they also may miss a significant proportion of truly growth‐restricted fetuses who do not necessarily meet such strict criteria [4]. Etiologies for FGR can be divided into extrinsic and intrinsic factors. The most common intrinsic etiologies include aneuploidy, congenital malformations, and congenital infection [5–7]. Extrinsic etiologies include chronic maternal hypoxia, maternal vascular disorders such as hypertension, diabetes, and pre‐eclampsia, poor maternal weight gain, and exposure to tobacco, illicit drugs, or teratogens [8–10]. The majority of these disorders cause relative placental hypo‐perfusion, thereby decreasing the flow of highly‐oxygenated blood to the fetus. In the absence of an identifiable cause for FGR, it becomes virtually impossible to distinguish pathologic FGR and constitutional “smallness” in utero. Large for gestational age (LGA) has traditionally been defined as EFW >90th percentile for gestational age; however, macrosomia is defined by absolute EFW >4000 g. Risk factors for macrosomia include maternal pre‐gestational or gestational diabetes, obesity, gestational age >40 weeks, prior child with macrosomia, excessive pregnancy weight gain, hydrops fetalis, and an elevated 50 g glucose challenge test with a normal 100 g glucose tolerance test [11, 12]. Macrosomia is most associated with adverse maternal and fetal events at the time of delivery. Macrosomic fetuses are at an increased risk for shoulder dystocia and its related fetal injuries such as clavicular fractures, humeral fractures, and brachial plexus injuries. Maternal adverse events associated with macrosomia include increased risk for Cesarean section, postpartum hemorrhage, and third and fourth degree lacerations [4]. Despite heightened awareness of these adverse risks, approximately 50% of macrosomic fetuses are not diagnosed until the time of birth [13–15]. In order to appropriately manage the patients in the above clinical scenarios, a critical appraisal of the literature was performed to address the following questions surrounding the management of pregnancies affected by fetal growth disorders. Using standardized population‐based growth curves, approximately 10% of pregnancies will be diagnosed with small‐for‐gestational age (SGA) fetuses; however, not all of these fetuses will be pathologically growth‐restricted. Additionally, some fetuses who measure greater than the 10th percentile for gestational age actually will be pathologically growth‐restricted and will be missed using population‐based growth curves. This has led to the concept of “individualized fetal growth potential.” Using this concept, a predicted “term optimal weight” is calculated for each fetus adjusting for maternal physiologic or constitutional variables such as height, weight, parity, and ethnicity. A customized fetal growth curve is then created which is used to follow fetal growth throughout pregnancy [16, 17]. In the context of this conceptual model, a fetus who should measure in the 70th percentile for gestational age but is actually measuring in the 25th percentile may be at higher risk for perinatal morbidity and mortality than a fetus who measures in the 8th percentile who is born to constitutionally small parents. To date, there are no randomized controlled trials (RCTs) comparing customized growth curves to population‐based standards; however, there have been multiple, large observational studies evaluating the use of these customized growth curves in predicting perinatal morbidity in mortality. In 2009, Gardosi et al. retrospectively compared perinatal outcomes in pregnancies classified as SGA by both population‐based and customized growth curves. In this study, 17.4% of babies who were classified as SGA by population‐based standards were not SGA by customized standards. Additionally, 32.7% of babies who were classified as SGA by customized standards would not have met SGA criteria by population‐based standards. This group of patients who were identified as SGA using the customized growth curve only had the highest risk of adverse perinatal outcomes including pre‐eclampsia, stillbirth, and neonatal death [18]. Additionally, Odibo et al. have demonstrated that customized growth curves have a sensitivity and specificity of 32.7% and 95.1%, respectively, for predicting stillbirth compared to a sensitivity and specificity of 0.8% and 98.0% using population‐based standards [19]. These particular studies derived their customized growth curves using norms from particular United States populations; however, similar results have been replicated both in other samplings of the US population as well as in other European populations [20–24]. Other authors have argued that these increased risk estimates for intrauterine fetal demise and neonatal death may be artificially inflated due to the large proportion of preterm fetuses identified as growth‐restricted using customized growth curves. These authors argue that the differences in risk stem from the manner in which the two types of growth curves are developed. Whereas population‐based growth curves are constructed using actual birth weight (BW), customized growth curves are constructed using intrauterine fetal weight. Given that intrauterine fetal weight traditionally has been an underestimate of observed birth weight in preterm infants, more preterm infants will be classified as SGA using the customized growth curve. This could potentially explain the higher incidence of stillbirth and neonatal mortality that is observed in these SGA infants. Zhang et al. demonstrated that there was only a modest difference in perinatal death after adjusting for gestational age when comparing the two curves [25]. Furthermore, Hutcheon et al. demonstrated that the addition of maternal characteristics to gestational age and gender contributed little explanation for the variance observed in birth weight using the customized growth curve [26, 27]. While the use of customized growth curves is gaining popularity in European countries, this approach has yet to be widely accepted in the United States. Further trials to evaluate the antenatal detection rate of true pathologic FGR using both population‐based and customized growth curves are necessary. Additionally, the practical aspects of introducing customized growth curves into daily obstetric practice warrants further investigation. Quality of Evidence: Level B, Class II The well‐established association between FGR and stillbirth has lead physicians to adopt a strategy of increased antenatal surveillance in such patients. The BPP and NST are the two most commonly employed strategies of assessing fetal well‐being in women at high risk for stillbirth. The goal of these tests is to detect fetuses at risk for developing acidosis or hypoxemia in order for physicians to intervene prior to further deterioration in fetal status. Despite their widespread adoption into clinical medicine, RCTs demonstrating their efficacy in decreasing stillbirth are lacking. The NST incorporates baseline fetal heart rate as well as measures of fetal heart rate variability. A reactive NST after 32 weeks’ gestation is defined by the presence of at least two fetal heart rate accelerations of at least 15 beats per minute lasting for greater than 15 seconds over a 20‐minutes period [28]. A reactive NST reflects adequate oxygenation of the fetus and is representative of an intact fetal central nervous system. Advantages of the NST include its ease of administration as well as its high negative predictive value of >99.8%; however, limitations include its high false positive rate of >50%, especially at early gestational ages, as well as its relatively high interobserver and intraobserver variability [28–30]. While NSTs have traditionally been performed twice weekly, there remains a lack of evidence to support this practice. In 2000, a RCT was performed comparing a regimen of twice weekly NSTs to fortnightly NSTs in a population of women with SGA fetuses with normal UA Doppler studies. There was no difference in neonatal outcomes between the two groups; however, there was an increased incidence of induction of labor in the twice weekly NST group [31]. The BPP is another method of antenatal surveillance which incorporates evaluation of AFI, fetal movement, fetal tone, and fetal breathing with or without a NST. When normal, each parameter receives two points, for a maximum of 10 points. The NST portion of the exam may be omitted, leaving a score of 8/8 as a maximum [28, 32, 33]. As fetal status deteriorates, there is progressive loss in fetal breathing and AFI followed by loss of fetal heart rate reactivity. Fetal movement and tone are typically the last parameters to become abnormal. Overall, abnormal BPP scores have been associated with worsening acid–base status at the time of delivery [34, 35]. Similar to the NST, the BPP also has been associated with a high false positive rate, and there is lack of high quality evidence from RCTs to support its use in the evaluation of high‐risk pregnancies [36]. Kaur et al. evaluated the use of daily BPPs in preterm fetuses with severe FGR (<1000 g with abnormal UA Doppler indices). Results from this study demonstrated that the BPP was not reliable in the evaluation of preterm severe FGR given both its high false positive and false negative rates [37]. The BPP also gives important information regarding AFI. Amniotic fluid index has been shown to progressively decrease in cases of FGR [38, 39]. A normal AFI is typically associated with adequate placental perfusion; whereas, a low AFI may be a sign of worsening placental dysfunction. Low AFI may also be found in cases of membrane rupture or congenital fetal abnormalities. A 1999 meta‐analysis demonstrated that oligohydramnios (AFI < 5 cm) was associated with an increased rate of Cesarean section for fetal distress as well as low 5‐minutes Apgar scores; however, oligohydramnios was not associated with fetal acidosis [40]. Alternatively, in a retrospective study of preterm FGR cases only, Scrifres et al. identified oligohydramnios as an independent predictor of perinatal mortality [41]. Regardless, the finding of oligohydramnios on ultrasound always warrants further fetal evaluation in an attempt to establish an etiology. Quality of Evidence: Level B, Class IIa More recent data has suggested that changes in fetal parameters as reflected by NSTs or BPPs are rather late occurrences in the series of events leading to fetal demise [42]. In fact, fetal acidosis may already be present in a proportion of fetuses displaying abnormalities in these antenatal tests. This has led to the investigation of other parameters which can be used in the evaluation of the growth‐restricted fetus. Doppler interrogation of fetal vessels has become a common strategy in the evaluation and surveillance of FGR. The most common vessels which are evaluated include the UA, middle cerebral artery (MCA), and ductus venosus (DV). In normal gestations, placental resistance declines with advancing gestation leading to an increased amount of forward diastolic flow in the UA. With progressive uteroplacental insufficiency, there is elevated placental resistance leading to decreased forward diastolic flow which can progress to absent or even reversed diastolic flow in the most severe cases. UA Doppler is reported as a systolic to diastolic ratio (S/D) or as a PI [systolic – diastolic flow/mean]. The fetal MCA is typically a high impedance vascular bed with low end‐diastolic velocity. In cases of progressive FGR, there is thought to be preferential shunting of blood toward vital organs such as the brain and heart at the expense of visceral organs, resulting in a lower MCA S/D ratio or PI. This phenomenon is known as the “brain‐sparing effect.” Finally, evaluation of the fetal venous system is an indirect measure of fetal cardiac compliance. Doppler interrogation of the DV produces a tri‐phasic waveform comprised of S, D, and a waves. The S and D waves occur with ventricular contraction and then passive diastolic filling, respectively. The a wave is a reflection of ventricular filling which occurs during atrial systole or “atrial kick.” With worsening right ventricular dysfunction, the a wave will become decreased or even reversed. This reflects decreased or reversed forward flow during atrial systole [4]. Examples of normal waveforms for each of these vessels are shown in Figure 43.1. Figure 43.1 Normal Doppler Waveforms in (a) the umbilical artery, (b) the middle cerebral artery, and (c) the ductus venous. Multiple studies have evaluated the association between abnormal UA Doppler parameters and adverse perinatal outcomes. Studies evaluating fetal blood samples from cordocentesis specimens have shown an increased incidence of both hypoxia and acidosis with worsening UA Doppler studies [43, 44]. Abnormal UA Doppler studies have also been associated with other adverse perinatal outcomes such as neonatal intensive care unit (NICU) admission, low Apgar scores, and fetal distress, with absent or reversed end diastolic flow being the most ominous finding and associated with the highest risk of perinatal mortality [45–47]. Recently, Vergani et al. evaluated predictors of adverse neonatal outcomes in both late preterm and term growth‐restricted neonates. Results from this study demonstrated that gestational age was the most important predictor of adverse outcome in term infants; however, in preterm infants, UA PI was found to be an independent predictor of adverse outcome [48]. There have also been multiple RCTs evaluating the efficacy of UA Doppler surveillance in improving perinatal outcome. When including only those studies which were performed in high‐risk populations, improved outcomes with the use of Doppler surveillance has been demonstrated in the majority of studies; however, there are limitations in sample size, methodology, and randomization. In order to surpass these issues, results from these trials have been synthesized in meta‐analyses in order to determine a more accurate effect size. Table 43.1 shows a summary of these results in the current published literature [49–52]. Of note, each of these studies shows a decrease in perinatal mortality with the use of Doppler surveillance in high‐risk pregnancies such as those with FGR. Additionally, these meta‐analyses also showed a decreased risk of antenatal admission, inductions of labor, and Cesarean section in the groups monitored with UA Doppler. Quality of Evidence: Level A, Class I Table 43.1 Summary results of currently published meta‐analyses comparing routine umbilical artery Doppler evaluation versus no umbilical artery Doppler evaluation in high risk pregnancies OR, odds ratio and CI, confidence interval. a Finding not statistically significant. The use of MCA Doppler studies in the routine surveillance of FGR has not been as widely accepted in clinical practice. In an observational study, Mari et al. demonstrated a lower risk of adverse outcomes in growth‐restricted fetuses with a normal MCA PI compared to those with an abnormal MCA PI [53]. Nanthakomon et al. showed that growth‐restricted fetuses with a normal UA PI but abnormal MCA PI had worse outcomes compared to those with normal UA and MCA PIs [54]. Furthermore, a longitudinal study by Hecher et al. has demonstrated progressive abnormality in the MCA PI as early‐onset FGR worsens [39]. Unfortunately, this finding has not been consistently replicated in other studies [42, 55]. More recently, it has been suggested that the MCA peak systolic velocity (PSV) may also be an informative marker in evaluating FGR, and trends in both the MCA PI and MCA PSV may provide more useful clinical information than single measurements [56]. Additionally, the cerebroplacental ratio (CPR) has been shown to be predictive of adverse perinatal outcome [57, 58]. This parameter is calculated by dividing the MCA PI value by the UA PI value and, therefore, incorporates information regarding placental status and subsequent fetal response. Finally, it has also been suggested that MCA Doppler studies also may have utility when performed in the third trimester in pregnancies complicated by FGR; however, there remain no RCTs available to guide the use of MCA Doppler in the clinical management of FGR [59, 60]. Quality of Evidence: Level B, Class IIb Interrogation of the fetal venous circulation provides indirect information regarding the status of the fetal cardiovascular system in response to FGR. Baschat et al. has shown that growth‐restricted fetuses with abnormal venous flow have a higher rate of adverse perinatal outcomes compared to those with Doppler abnormalities in only the UA or MCA [61]. Turan et al. showed that an absent/reversed a wave in the DV lasting >seven days had 100% sensitivity and 80% specificity for detecting stillbirth; however, this finding was unrelated to neonatal morbidity and mortality [62]. A systematic review and meta‐analyses demonstrated a modest predictive ability of abnormal DV Doppler for the prediction of perinatal mortality with a positive likelihood ratio of 4.21 (95% confidence interval (CI) 1.98–8.96) and a negative likelihood ratio of 0.43 (95% CI 0.30–0.61) [63]. The optimal strategy of incorporating Doppler studies and fetal well‐being assessment with BPP and NST is still under investigation. Cosmi et al. evaluated a group of 145 growth‐restricted fetuses with abnormal UA Doppler studies and divided them into two groups: Group 1: all indices (MCA, DV, and AFI) became abnormal preceding a non‐reassuring BPP or NST; Group 2 : 1 or more indices were normal at the time of delivery. Although there was no statistically significant difference in perinatal morbidity and mortality between the two groups, UA reversed flow and DV absent/reversed flow were independently associated with adverse outcome [38]. In another study comparing NST, computerized fetal heart rate analysis, BPP, and arterial and venous Doppler, venous Doppler was found to be the most predictive of acidemia with a sensitivity of 73% and specificity of 90% [64]. Furthermore, Baschat et al. has shown that both multivessel Doppler assessment and BPP evaluation can effectively risk‐stratify growth‐restricted fetuses; however, their results do not consistently correlate with each other [65]. This finding would suggest that complementary use of these two modalities would be most effective. Prior studies have shown that multivessel Doppler may only identify fetuses at high risk for demise 24 hours earlier than BPP [42, 66]. A 2004 decision analysis evaluated four strategies of antepartum FGR assessment including Doppler + BPP, BPP only, Doppler only, and no testing. This analytic model actually demonstrated that BPP only was the best strategy to guide physicians on the timing of delivery in preterm growth‐restricted fetuses [67]. Most recently, the Trial of Umbilical and Fetal Flow in Europe (TRUFFLE) was undertaken to evaluate the role of venous Doppler evaluation as a trigger for delivery in preterm FGR. This multicenter RCT randomized women with singleton gestations with preterm FGR (26–32 weeks) and elevated UA Doppler studies to one of three groups as a trigger for delivery: (i) reduced short‐short term variation on cardiotocography monitoring; (ii) early DV changes of elevated PI; or (iii) late DV changes of absent or reversed a‐wave. There was no significant difference in the primary outcome of survival without neurodevelopmental impairment at age two among the three groups. There was a significant increase in intact survival for infants randomized to delivery on the basis of late DV changes compared to cardiotocography monitoring; however, this was at the expense of an increased, but non‐significant, risk of mortality [68]. Based on the available data, the role of multi‐vessel fetal Doppler interrogation in the clinical management of the growth‐restricted infant remains uncertain. Quality of Evidence: Level B, Class IIb Based on the above data, the optimal surveillance strategy to prevent adverse perinatal outcome in growth‐restricted fetuses remains uncertain [36]. This produces a clinical dilemma in determining optimal timing of delivery for these fetuses. In cases of maternal distress or imminent fetal compromise, the decision to deliver is straightforward; however, the majority of cases of FGR require the clinician to weigh the risk of stillbirth with the risks of prematurity and neonatal death in determining optimal delivery timing. To date, the only RCT to evaluate timing of delivery for preterm FGR is the Growth Restriction Intervention Trial (GRIT) [69]
Disorders of fetal growth
Background
Clinical questions
Critical appraisal of the literature
Authors
Year
Number of trials included
OR (95% CI)
Improvement in perinatal outcome
Alfirevic & Neilson
1995
12
0.62 (0.15–0.55)
Yes
Maulik et al.
1998
3
0.19 (0.06–0.63)
Yes
Westergaard et al.
2001
6
0.66 (0.36–1.22)
Yes a
Cochrane Review
2010
18
0.71 (0.53–0.98)
Yes
Stay updated, free articles. Join our Telegram channel

Full access? Get Clinical Tree
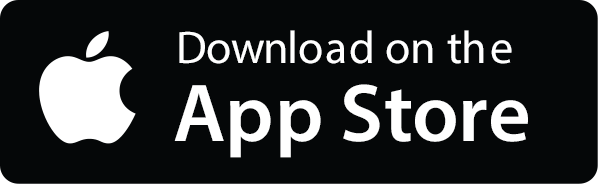
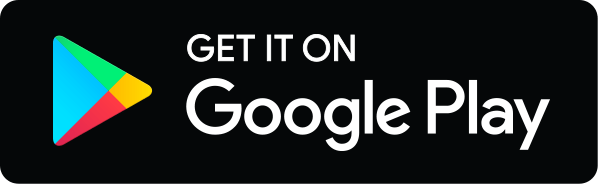