CHAPTER 29 Sarah J. Kilpatrick Department of Obstetrics and Gynecology, Cedars‐Sinai Medical Center, Los Angeles, CA, USA Thyroid disease is the second most common endocrine disease encountered in pregnancy. Clinical hypothyroid disease, defined as elevated thyroid stimulating hormone (TSH) with suppressed free thyroid hormone (FT4) affects approximately 0.3–0.5% of pregnant women while it is likely that more than 1% of women come to pregnancy with a diagnosis of hypothyroid disease on T4 and are clinically euthyroid [1–3]. See Table 29.1 for the laboratory diagnostic criteria for thyroid diseases. The most common causes of hypothyroid disease in the US are Hashimoto’s thyroiditis and treated Graves’ disease. In contrast, worldwide the most common cause of hypothyroid disease is iodine deficiency. Subclinical hypothyroid disease, defined as an elevated TSH but normal FT4, is more prevalent in approximately 2% of pregnant women, and its impact on pregnancy is more controversial [1, 2]. Hyperthyroidism, largely Graves’ disease, affects approximately 0.2% of pregnant women [1, 2, 4]. Uncontrolled hyper‐ or hypothyroid disease in pregnancy is associated with multiple adverse perinatal outcomes such as low birth weight, pre‐eclampsia, and possibly fetal loss [5–7]. However, all of these are small, retrospective reports and certainly there will never be randomized trials comparing treated to untreated women with thyroid disease in pregnancy because it is well known that untreated clinical hyper‐ or hypothyroid disease in the nonpregnant individual leads to significant morbidity. Withholding appropriate treatment from pregnant women would be unacceptable. Hence clinical thyroid disease should be appropriately diagnosed and treated in pregnancy to achieve the best maternal and perinatal outcomes. Consistent with the concept that treatment of clinical thyroid disease optimizes outcome, women with treated clinical hypothyroid disease had no increase in adverse maternal or neonatal outcomes compared to women without thyroid disease in a large retrospective case‐control study [3]. Table 29.1 Thyroid dysfunction and TSH and FT4 Levels Understanding the effect of pregnancy on thyroid function is imperative to managing thyroid disease in the pregnant woman. Thyroid hormone production increases in pregnancy as does thyroid hormone binding globulin (TBG) beginning in early pregnancy because of the increase in estrogen and consequently the total (thyroxine (T4) and total triiodothyronine (T3) increase [8].Therefore unless one uses trimester specific norms, the typical laboratory norms are not relevant to pregnancy, although some have advocated to simply multiply the normal nonpregnant range of TT4 by 1.5 to adjust for pregnancy [9]. Free T4 and free T3 do not increase in pregnancy and may actually decrease slightly because of the increase in TBG. Traditionally, it has been thought that the normative range of TSH does not change in pregnancy. However, because human chorionic gonadotropin (HCG) is homologous to TSH with identical alpha subunits and similar beta subunits, as HCG rises in the first trimester it stimulates the TSH receptor which can result in transient increase in FT4 and suppression of TSH, particularly between 8 and 14 weeks’ gestation. This normal suppression of TSH has led experts to suggest that in the first trimester the upper limit of normal TSH should be lowered to as low as 2.5–3.5 mIU l−1 and several laboratories have adopted trimester specific norms for TSH [10–12]. See Table 29.2 for trimester specific norms for TSH and FT4. Suppression of TSH is more common in multiple gestations due to the increased placental mass, and occurred in 18% pregnancies in the first trimester; 10% of these women exhibited transient symptomatic thyrotoxicosis [13]. All of the women with symptomatic thyrotoxicosis had spontaneous remission within eight weeks [13]. Therefore, checking TSH in the first trimester should be reserved for the woman in whom one is concerned about clinical hyperthyroid or hypothyroid disease. Suppressed TSH in the first trimester is not diagnostic of hyperthyroidism. Table 29.2 TSH trimester specific norms a a De Groot et al. 2012 [10] and Stagnaro‐Green et al. 2011 [12]. Iodine clearance is significantly increased in pregnancy in part due to increased renal clearance from the increase in renal blood flow in pregnancy. This increase results in lower plasma iodide levels and in some women a small increase in thyroid gland size [14, 15]. In addition, there is significant transfer of maternal iodine to the fetus as the fetal thyroid gland becomes functional which makes maternal iodine supplementation important. The World Health Organization (WHO), The Institute of Medicine (IOM), the American Thyroid Association (ATA), and the Endocrine Society recommend that pregnant women receive a total of 220–250 μg of daily iodine [10, 16, 17]. Prenatal vitamins should have at least 150 μg per pill [18]. Potassium iodide is the preferred iodine source in pregnancy because it is a more consistent iodine source than kelp [17]. The fetal thyroid begins to use iodine by 10–12 weeks’ gestation with subsequent production of T4 by 12–14 weeks’ gestation [14]. T4 increases from approximately 18 week until 36 weeks when adult levels are reached [19]. Maternal T4 does cross the placenta in the first trimester and there is a correlation between fetal and maternal T4 levels [20]. Before 10–12 weeks’ gestation, maternal T4 is the only source of T4 for the fetus and is important for brain development [21–23]. The most dramatic evidence of this is the resulting cretinism in neonates of mothers with severe iodine deficiency and subsequent severe untreated hypothyroidism during pregnancy [22]. Data in rats suggest that reduced maternal T4 in early pregnancy was associated with permanent abnormal brain development in fetuses [21]. Clearly clinical hypothyroidism should be avoided in pregnancy. Although TSH does not cross the placenta, thyrotropin‐releasing hormone and TSH receptor immunoglobulins (thyroid stimulating immunoglobulin (TSI), thyroid‐stimulating hormone‐binding inhibitory immunoglobulin (TBII)) do cross the placenta. In a prospective series of women with primary hypothyroidism planning pregnancy, thyroid function was measured before and during pregnancy [24]. In 85% of the women it was necessary to increase the levothyroxine dose to offset the rise in TSH observed as early as five weeks’ gestation. The authors recommended increasing the T4 dose by 30% by eight weeks gestation corresponding to their findings. The increased T4 requirement peaked at approximately 16 weeks’ gestation with a mean 47% increase by that time. In another paper, only 38% of women with hypothyroid disease before pregnancy required an increase in their thyroid replacement. The only difference between the women requiring an increase and those not requiring one is that those women requiring an increase in dose had had a recent change in their medication dose before pregnancy [25]. Based on these two papers it seems reasonable to check a TSH in the first prenatal visit in women with hypothyroid disease and treat them appropriately. Alternatively, raising the replacement dose by 20–30% and repeating TSH in four weeks is acceptable. In general, it is sufficient to measure only TSH in women who come to pregnancy with a diagnosis of clinical hypothyroid disease. The goal during pregnancy is to maintain the TSH in the normal or pregnancy specific range throughout the entire pregnancy. As noted above many women will need an increase in their T4 replacement early in pregnancy. Once a change in dose has occurred it takes four to six weeks to see the maximum effect of the dose change. Therefore, there is no need to repeat the TSH until four weeks after a dose change. Once the TSH is in the desired range, then most obstetric experts recommend checking the TSH every trimester although endocrinologists recommend more frequent testing at every four to six weeks [11]. It is important to remember to reduce the dose of T4 postpartum if it was increased during pregnancy. Approximately 5–10% of women are TPOAb positive in pregnancy [26–28]. The presence of TPOAb in pregnancy is associated with the development of postpartum thyroid dysfunction and the progression years later to hypothyroid disease [28, 29]. By seven years postpartum 46% of women positive for TPOAb in pregnancy developed thyroid dysfunction compared to only 1% of women without TPOAb during pregnancy [29]. However, how this should be managed in the pregnant patient is still unclear [28, 30]. For example, in a randomized controlled trial 55% of TPOAb positive women developed postpartum thyroid dysfunction, but that incidence was not significantly affected by prenatal iodine supplementation [28]. The relationship of prenatal TPOAb positivity and acute perinatal outcomes is not clear with multiple conflicting findings. A study from Finland reported a weak association between first trimester maternal TPOAb positivity and low birth weight (OR 1.7 (1.01–3.0)) but no association with preterm delivery (PTD) or small for gestational age [31]. They also found a significant increase in perinatal mortality with TPOAb positivity but no association between maternal clinical hypothyroid or subclinical hypothyroid disease and perinatal outcomes. Another study found no difference in perinatal outcomes between TPOAb positive, whether treated or not with T4, and TPOAb negative women [32]. TPOAb positivity in pregnancy has been associated with an increased risk in abruption [26, 33]; TPOAb positivity in the second but not first trimester of pregnancy was significantly associated with an increased risk for abruption, (OR 2.14; 0.18–3.89) although the percentage abruption was only 1.78% and 0.82% in the TPOAb positive and TPOAb negative women, respectively [33]. However, there are no reports evaluating the effect of any treatment to reduce this increased risk of abruption in TPOAb positive women. In other studies, no difference in PTD, hypertensive disorders, diabetes or preterm rupture of membranes was noted between TPOAb positive and negative women [26, 32, 34]. Although TPOAb cross the placenta there is no evidence that these antibodies affect fetal thyroid development [35]. TPOAb positivity has also been associated with a significant increase in the risk of miscarriage [36]. In a meta‐analysis of case‐control and cohort studies performed between 1991 and 2011, the OR was 3.90 (2.48–6.12) in cohort studies, and 1.80 (1.25–2.60) in case‐control studies for miscarriage [36]. There are only two randomized controlled trials comparing treatment with thyroid hormone replacement to placebo in women with TPOAb [37, 38]. In one study over 900 pregnant women were screened for TPOAb, and the 115 women (11.7%) that were found to be positive for TPOAb were randomized to either supplemental T4 or no treatment [37]. Women were excluded for overt thyroid disease, so all women were euthyroid at the time of randomization. These two groups of women were then compared to TPOAb negative women. Untreated TPOAb positive women had significantly higher TSH and lower T4 by delivery than treated TPOAb positive or TPOAb negative women. Untreated TPOAb positive women had a significantly higher miscarriage rate than untreated or control women (13.8%, 3.5%, and 2.4%, respectively; p < 0.05). However, the Cochrane review disputes that this effect was significant (risk ratio of 0.25 (0.05, 1.15)) [39]. Preterm deliveries were significantly higher in untreated compared to treated and control women (22%, 7%, and 8% respectively; p < 0.05). Although 19% of the untreated women had an abnormally high TSH by the time of delivery, it is not clear how many developed clinical hypothyroid disease. The same investigators randomized women positive for TPOAb undergoing fertility treatment to levothyroxine or placebo and then compared these women to TPOAb negative women [38]. Pregnancy rates were similar between these groups. Although miscarriage was significantly higher in the TPOAb positive women compared to TPOAb negative women, oddly, they did not compare miscarriage rates between levothyroxine treated and placebo treated women, perhaps because the number in each group was so small. However, the 2011 meta‐analysis concluded after analysis of the cohort, case‐control and randomized control trials (RCTs), that presence of TPOAbs is associated with increased risk of miscarriage and, “there is evidence that treatment with levothyroxine can attenuate the risks” [36]. In a cohort study of 293 pregnant women, children’s ability testing and IQ testing were compared in five‐year‐old children between those whose mothers who had TPOAb in pregnancy versus the mothers without TPOAb [30]. Even after controlling for maternal depression and current thyroid dysfunction, lower scores on the General Cognitive Scale remained significantly associated with TPOAb positivity during pregnancy, raising the concern that TPOAb positivity in pregnancy could be associated with impaired cognitive development in children [30]. There are no treatment trials as of 2016 testing whether treatment of TPOAb positive women during pregnancy impacts childhood outcomes. In nonpregnant women subclinical hypothyroidism has been associated with eventual development of overt hypothyroidism, but data supporting associations with other morbidities such as development of abnormal lipids or cardiac disease are poor [40]. In addition, there are no data that support benefits of treatment with T4 in nonpregnant individuals with subclinical hypothyroidism [40]. Thus the interest in potential maternal effects of subclinical hypothyroidism has been focused on the fetal/neonatal effects. There are numerous conflicting retrospective and prospective series evaluating the potential associations of maternal subclinical hypothyroidism and perinatal and childhood outcomes [2, 41–45]. In a paper utilizing second trimester serum, 2.2% of the over 10 000 women screened were found to have elevated TSH [2]. These women were compared to those women with normal TSH and FT4 and there was no difference in miscarriage, hypertensive disease, diabetes, or PTD. In contrast, in screening over 17 000 pregnant women, those women with subclinical hypothyroidism had significantly higher rates of delivery less than 34 weeks (4% vs. 2.5%; p = 0.01), and abruption (1% vs. 0.3%; p = 0.03) compared to women with normal TSH [42]. In a case‐control study comparing 11–13 week thyroid functions between 102 women who delivered before 34 weeks to 4318 women with a term delivery, no difference was reported in TPO antibody positivity, TSH or FT4 [41]. Utilizing the Swedish Medical Birth Register, a comparison of 8669 women reporting use of T4 during pregnancy to over 800 000 women who did not report use of T4, reported that pre‐eclampsia was significantly higher in those women using T4 (1.32; 1.19–1.47) [46]. However, PTD was only marginally greater in the T4 treated women (OR 1.13; 1.03–1.25) and, neither small for gestational age, or birth weight <2500 g were significantly different between the groups. Subclinical hypothyroidism was not associated with development of gestational diabetes, but was associated with a significant increase in severe pre‐eclampsia [44, 45]. Maternal hypothyroxinemia (FT4 subnormal but normal TSH) has not been shown to be associated with poor perinatal outcomes [47]. At a mean of 12 weeks, 1.3% of over 17 000 women had hypothyroxinemia and there was no difference in hypertensive disease, diabetes, PTD, or birth weight compared to women with normal thyroid function tests. In this same study women with subclinical hypothyroidism did have significantly higher PTD <34 weeks (4.3%) compared to women with normal thyroid function (2.5%) (p = 0.005). Thus the association between maternal elevated TSH or T4 treatment, with PTD or other obstetric complications appears to be negative or at least inconsistent. Based on nonrandomized trial data the relationship between maternal subclinical hypothyroidism or hypothyroxinemia and childhood outcomes is even more unclear. A widely publicized study by Haddow reported that elevated maternal TSH in second trimester stored serum from 62 women was significantly associated with cognitive dysfunction in seven‐year‐old offspring [42]. Although the mean IQs were not significantly different, the children of the mothers with elevated TSH did significantly worse on 2/14 neurocognitive tests. In contrast, a much larger population based cohort study of over 2700 mothers and children reported no relationship between maternal TSH level and 18 or 30 month expressive language or nonverbal or verbal functioning [48]. In this study women with hypothyroidism were excluded. There is one prospective case‐control study of 108 neonates whose mothers had FT4 in <10th percentile in the first trimester compared to controls with FT4 in the 50–90th percentiles [49]. The case neonates at three weeks of age had significantly worse score on only one variable (orientation) of a 7 variable neonatal behavioral assessment scale compared to the control neonates [49]. Severe hypothyroxinemia (FT4 <5th percentile in women with normal TSH) was significantly associated with expressive language delay and cognitive delay at 18 and 30 months [48]. A criticism of this paper is that all the childhood cognitive assessments were made by parents which could bias the results. However, if there is a significant relationship between reduced maternal thyroid function it makes more sense that low FT4 rather than elevated TSH would be the important variable because only T4 crosses the placenta [22]. These data are provocative and illustrate the need for randomized trials to test whether maternal treatment of either subclinical hypothyroidism or hypothyroxinemia would ameliorate childhood cognitive adverse outcomes. Finally in 2017 the first large treatment trial of pregnant women with subclinical hypothyroidism was published [50]. Out of 97 226 pregnant women who were screened, 3.1% were identified with subclinical hypothyroidism. The 677 women who met inclusion criteria (singleton, <20 weeks gestation) were then randomized to placebo or levothyroxine and developmental testing was performed on their children annually until age 5. There was no difference in median IQ scores at five years of age between the placebo (94) and levothyroxine (97) exposed children. The results of this large randomized controlled trial that there was no difference in five‐year‐old IQ scores between those children of mothers with subclinical hypothyroidism treated with placebo compared to those treated with levothyroxine supports the current American College of Obstetricians and Gynecologists (ACOG) recommendation not to routinely screen pregnant women for subclinical hypothyroidism [51]. Of note, these investigators also screened for maternal hypothyroxinemia and randomized these woman to placebo versus levothyroxine and found no difference in five year old IQ scores in these children [50]. There are at least two reports that maternal subclinical hypothyroidism is associated with subsequent development of clinical hypothyroidism [43, 52]. There was an increase in the development of clinical hypothyroidism seven years after birth in a small study of 62 women who had subclinical hypothyroidism in pregnancy compared to controls with normal thyroid function (64% vs. 4%) [43]. A much larger study of over 5000 women followed for 20 years, found that overt hypothyroidism, subclinical hypothyroidism, and TPO antibody positivity in pregnancy were all significantly associated with development of thyroid disease [52]. These data suggest that if any of these diagnoses are made, the patient should be informed that she is at risk for later develop thyroid disease. The short answer is no, however it is illustrative to appreciate the historical debate on this question. Since the 1999 publication of the US paper suggesting an association between maternal low TSH and childhood cognitive impairment described above [43], routine screening of pregnant women for subclinical hypothyroid disease has been heavily debated [53]. This debate largely revolved around disparate view on two questions: (i) Are the data convincing that subclinical hypothyroidism in pregnancy is associated with poor cognitive childhood outcomes; and (ii) Is the fact that there are no trials showing any efficacy of treatment in pregnancy important before routine screening is implemented. Despite the publication of several national guidelines advocating for screening of only high‐risk women, a high proportion of obstetric providers persist in screening low‐risk women [16, 40, 51, 54, 55]. Any screening test should at least meet the following two criteria: it must address a common problem and there must be evidence that treatment ameliorates the problem. Between 2000 and 2011 there was no clear evidence that treatment of women with subclinical hypothyroidism with T4 had any positive effect on perinatal or neonatal morbidity. However, in 2012 the first randomized controlled trial comparing screening and then treating women with elevated TSH or low T4, to untreated women reported no difference in any outcomes including PTD or IQs in the three‐year‐old children [56]. Women were randomized to screen or control groups at initial prenatal visit. The women in the screened group were screened and if positive were treated with 150 mcg levothyroxine at a median gestational age of 13 3/7 weeks. The control women were screened, but the results were not reviewed until delivery and hence no treatment was provided. Five percent of over 20 000 women were screen positive. As of 2016 there are now clear data from a large randomized trial that show that treatment of either subclinical hypothyroidism or hypothyroxinemia does not affect childhood cognitive outcomes [50]. These findings definitively support existing guidelines by ACOG to not perform routine screening for hypothyroid disease in pregnancy [51, 54]. Not only is it still unclear if there are untoward effects on childhood cognitive outcomes of mothers with elevated TSH, there are now data to show that levothyroxine treatment does not affect childhood cognitive outcomes. To further illustrate the ambivalence over this question of routine screening of all pregnant women for thyroid disease, two recent guidelines written by endocrinologists for the Endocrine Society and the American Thyroid Association reflect different opinions [10, 12]. The ATA states that there are insufficient evidence to recommend for or against routine screening and the Endocrine Society states that they could not reach agreement on this topic with five authors for routine screening and eight authors with no recommendation [10–12]. Targeted screening for hypothyroid disease in pregnancy has been advocated by ACOG, The Endocrine Society and others [12, 16, 54]. Women that should be screened for hypothyroid disease include those women with a personal or strong family history of thyroid disease, a history of autoimmune disease such as Type 1 diabetes, have known positive TPO antibodies, goiter, or history of neck irradiation. Several studies have evaluated the efficacy of selected screening in pregnancy [27, 57]. In a prospective cohort study, pregnant women were screened for thyroid function and antibodies in the first trimester [57]. These results were compared between women with no high‐risk history for thyroid disease and those women who were high‐risk for thyroid disease. A total of 413 women (25%) were classified as high‐risk. As expected significantly more high‐risk women had elevated TSH (6.8% vs 1.0% p < 0.0001). Although this validates the approach of screening only high‐risk women, the authors pointed out that they would have missed the 0.3% of women in the low‐risk group who had clinical hypothyroidism. Also of interest is that 23% of the women known to be on T4 were undertreated based on their elevated TSH. In a randomized controlled trial, over 4000 women in their first trimester were randomized to universal screening with TSH, FT4, and TPO antibodies versus testing only those women who were high‐risk for thyroid disease [27]. In each group approximately 20% (21.1% and 19.9%) of women met criteria for being high‐risk for thyroid disease and there was no difference in the percent of women who were euthyroid (approximately 97%) in each group. Hypothyroid was defined as TSH >2.5 mIU l−1 in TPOAb antibody positive women and these women were treated with levothyroxine. There were no women without TPOAb who had TSH >5.9 mIU l−1. There was no difference in perinatal adverse outcomes (including pre‐eclampsia, miscarriage, gestational diabetes, and PTD) between the universal screen group and the test case finding group. However, the authors argue that screening the low‐risk women picked up 2.8% with thyroid disease who were treated which might warrant screening low‐risk women even if there is no difference in adverse perinatal outcomes. It is unclear whether treatment of women with subclinical hypothyroidism in pregnancy is warranted [10, 12]. The recent ATA guidelines concluded that there are insufficient data to recommend for or against treatment in women with subclinical hypothyroidism and negative TPO‐ab but they do recommend treatment if TPO‐ab are positive and a diagnosis of subclinical hypothyroidism has been made [12]. In contrast, the recent Society of Endocrinology guidelines recommend treatment regardless of presence of TPO‐Ab although they acknowledge that the evidence to treat subclinical hypothyroidism in pregnancy when the TPO‐Ab are negative is poor [10]. PPT can present as new onset of hypothyroidism or hyperthyroidism within the first year after delivery and occurs in approximately 7% of women living in iodine sufficient areas [16, 58]. The majority of women with PPT are TPOAb positive and 40% of women with TPOAb develop PPT [59, 60]. Therefore, the strongest predictor for PPT is the presence of TPOAb. PPT is typically transient. The hyperthyroid symptoms of PPT often present at approximately three months postpartum, are generally mild and last only a couple of months which distinguishes PPT from new onset Graves’ disease. About 30% of women with PPT experience only hyperthyroid symptoms. Some women will exhibit hypothyroid symptoms after the initial hyperthyroidism while others exhibit only hypothyroid symptoms. The hypothyroid phase usually lasts only four to six months [61]. There are no trials that direct treatment of PPT. However, expert opinion suggests consideration of treatment of the hypothyroid phase if the TSH is >10 mIU l−1, and consideration of propranolol for women with hyperthyroid symptoms [58]. If the woman is trying to conceive then treatment with synthroid is recommended for a TSH >4 mIU l−1 [58].
Thyroid disease
Background
Disorder
TSH
FT4
Clinical or Overt hypothyroidism
Elevated
Reduced
Subclinical hypothyroidism
Elevated
Normal
Hypothyroxinemia
Normal
Reduced
Clinical or Overt hyperthyroidism
Suppressed
Elevated
Subclinical hyperthyroidism
Suppressed
Normal
Hyperthyroxinemia
Normal
Elevated
TSH (mIU l−1)
First trimester
0.1–2.5
Second trimester
0.2–3.0
Third trimester
0.3–3.0
Hypothyroid disease
Clinical questions
Conclusions
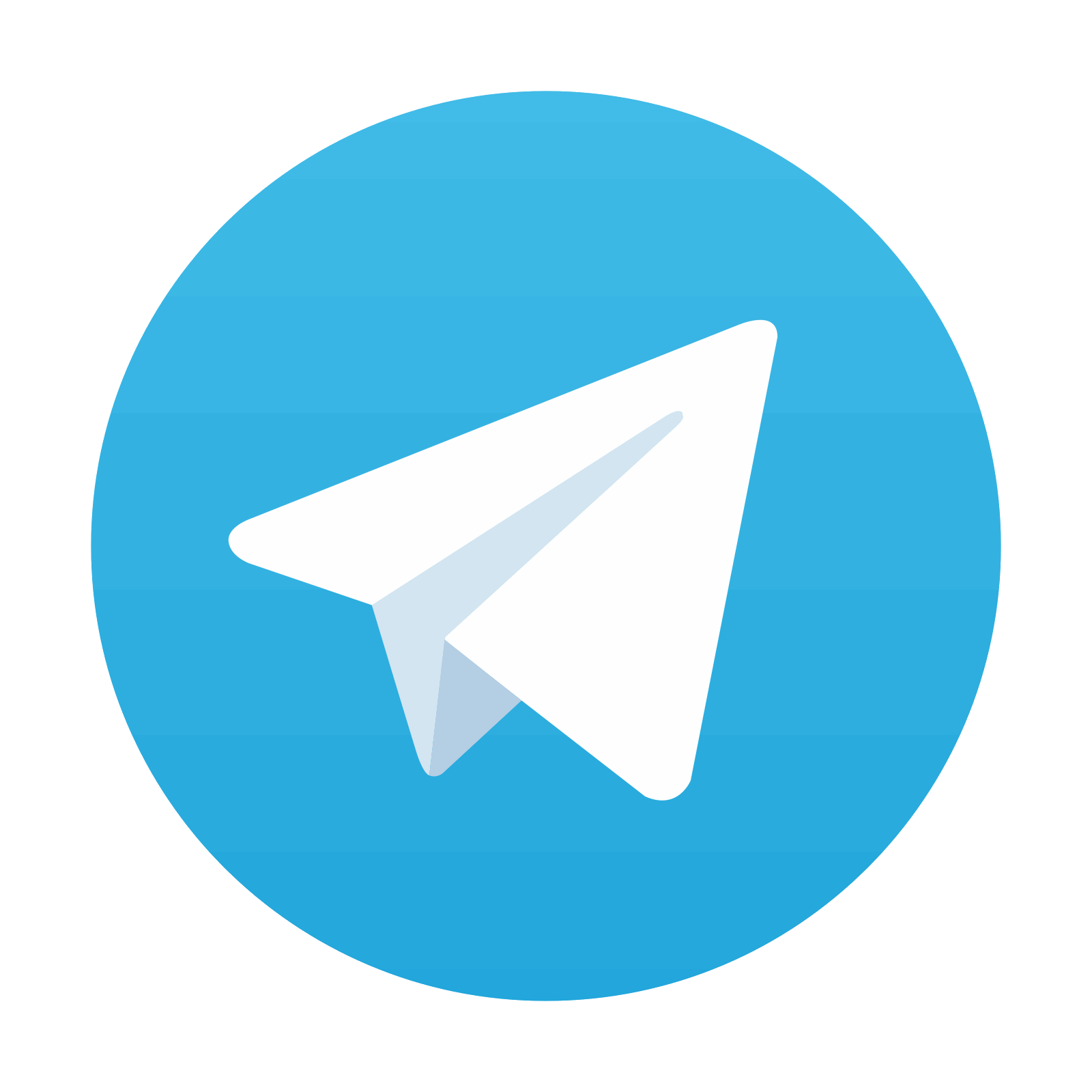
Stay updated, free articles. Join our Telegram channel

Full access? Get Clinical Tree
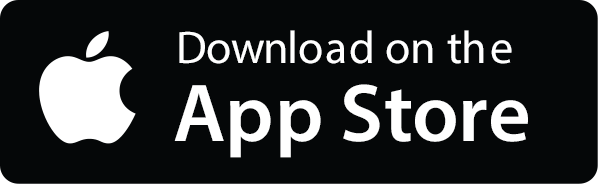
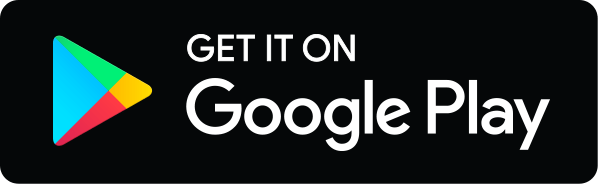