Abstract
Respiratory diseases in pregnancy such as pneumonia or thromboembolic disease are relatively common, and thoracic imaging can be crucial to the diagnosis. Radiologic modalities are subdivided into ionizing or non-ionizing techniques. In pregnancy, ideally, it is preferable to choose non-ionizing modalities to avoid radiation exposure. However, the choice of imaging modality also needs to take into account the modality best likely to obtain the diagnosis. Amongst physicians, the perceived fetal risk from ionizing radiation exposure in pregnancy is generally higher than the actual risk.
Introduction
Respiratory diseases in pregnancy such as pneumonia or thromboembolic disease are relatively common, and thoracic imaging can be crucial to the diagnosis. Radiologic modalities are subdivided into ionizing or non-ionizing techniques. In pregnancy, ideally, it is preferable to choose non-ionizing modalities to avoid radiation exposure. However, the choice of imaging modality also needs to take into account the modality best likely to obtain the diagnosis. Amongst physicians, the perceived fetal risk from ionizing radiation exposure in pregnancy is generally higher than the actual risk. It should be remembered when counselling pregnant women that the majority of ionizing radiation in diagnostic imaging falls well below the threshold at which teratogenic or carcinogenic effects are noted. One should ask oneself when faced with an unwell pregnant woman, ‘what would I do if she were not pregnant?’. The general consensus from all the guidelines is that if a particular diagnostic scan is likely to change clinical outcome, then it should be performed American College of Radiology (ACR),1 American College of Obstetrics and Gynaecologists (ACOG), American Thoracic Society (ATS),2 Society of Obstetrics and Gynaecologists Canada (SOGC)3 and Royal College of Obstetrics and Gynaecologists UK (RCOG). Good practice is for the dose to the patient of any radiographic procedure to abide by the ALARA principal, i.e. be ‘As Low As Reasonably Achievable’. This chapter details both the fetal and maternal concerns with commonly used radiation imaging. We also describe the benefits of various imaging modalities and their associated safety profiles, both in the antepartum and lactating phases.
Fetal Effects of Radiation Exposure
The effect of radiation on the fetus is determined by both gestational age at exposure and dosage of radiation. Assessing the exact gestational age is extremely important, and yet confusing. By convention, gestational age is counted from the first day of the last menstrual period. However, ovulation typically occurs on day 14 of that cycle, and hence conception occurs between 2–4 weeks from the last menstrual period. For the purpose of this chapter, gestational age will be counted from the actual time of conception rather than from the last menstrual period.
Timing of radiation exposure can be subdivided into the following periods: pre-implantation (0–2 weeks post-conception), embryogenesis (2–8 weeks post-conception), early fetal (8–15 weeks) and late fetal (15–40 weeks). After the 25th week of gestation there is decreased radiosensitivity, and congenital anomalies are highly improbable. In the pre-implantation period radiation exposure typically results in an ‘all or none’ effect: either death of the embryo, or no effect whatsoever. The period of embryogenesis (2–8 weeks) is very sensitive to the teratogenic effects of ionizing radiation. In the early fetal period (8–15 weeks) the neuronal cells are rapidly dividing and migrating, and as a result the central nervous system can be affected (see Table 20.1). The effects of radiation exposure can be classified as deterministic or stochastic. The deterministic effects occur principally above a threshold dose and consist of cellular damage resulting in cell death. Stochastic effects are delayed and manifest only after a period of time following exposure. These effects consist of damage to cellular nuclear material and result in mutagenic effects. There is no specific threshold radiation level at which a stochastic effect occurs. Much of the information on the effect of radiation on the fetus is derived from environmental exposure, animal studies and human exposure to diagnostic and therapeutic radiation. The following sections discuss the evidence from both environmental and diagnostic radiation exposure. In this chapter the unit of absorbed energy referenced is the milligray (mGy). A mGy is equivalent to 0.001 gray. A gray is a dose of one joule of energy absorbed per kilogram of matter. The alternative non-SI unit which is no longer in common usage is the rad (0.01 Gy). To place the subsequent discussion in perspective, fetal radiation exposure from medical diagnostic imaging procedures is in the range of 1 mGy to 100 mGy (discussed in more detail below).
Table 20.1 Timing of exposure and effect of radiation
Timing of exposure (post-conception) | Effect of radiation | Threshold dose (mGy) |
---|---|---|
| Death or no consequence | ≥50–100 |
| Congenital anomalies | ≥200 |
|
|
|
|
|
|
Environmental Exposure and In Utero Effect
In 1945, the Japanese cities of Hiroshima and Nagasaki experienced the first use of atomic weaponry. An epidemiological study of atomic bomb survivors who were exposed in utero was established to determine the long-term effects of the exposure. The cohort consisted of 3300 individuals exposed at the time of the bombings. No clear evidence was found of a link between parental radiation exposure and the frequency of untoward pregnancy outcomes such as congenital malformations, stillbirths or peri-natal deaths. However, an increase in intellectual disability was found amongst those exposed in utero during the early and late fetal periods. This was accompanied by a reduction in head circumference. An IQ test administered to 1670 school-age children demonstrated a general decrease in IQ of 25 points at exposures of >1000 mGy between 8–15 weeks post-conception.4 In relation to the carcinogenic effect of radiation, during the first 15 years of life there was only one death recorded from leukaemia in the in utero cohort.
Additional evidence from fetal environmental radiation exposure is available from the nuclear disaster that occurred in Chernobyl in 1986. Over a 10-day period, the emission of radioactive material, predominantly 131I, affected areas not only in Ukraine but across Western Europe remote from the reactor site. 131I is absorbed by the thyroid gland. Five years following the disaster, an unusually high occurrence of childhood thyroid cancer was documented in Ukraine, Belarus and Russia. However, this was noted only in those who were children at the time of the disaster and not with exposure in utero. It was postulated that the fetal thyroid was protected by maternal physiology.5 In contrast, the rates of childhood leukaemia were noted to be higher among those exposed in utero at the time of the explosion. The number of cases was small and rates were not consistent between studies and exposed countries. Nevertheless, the highest annual incidence of childhood leukaemia was recorded in 1987, one year after the disaster and occurred in the most contaminated regions in Belarus.6
Diagnostic Imaging and In Utero Effect
Biological effects such as congenital anomalies are deterministic. In a study published in 1929, 34% of 74 neonates exposed to pelvic irradiation for maternal uterine cancer had congenital anomalies. These anomalies included microcephaly, hypoplasia of genitalia and cataracts. The estimated dose of radiation was >100 mGy. The authors concluded that the most serious congenital anomalies occurred when exposure was between 3 and 19 weeks’ gestation (it is not specified in the text, but presumably this refers to 3–19 weeks following last menstrual period, which would have been the standard way of reporting at the time).7
The largest and most comprehensive study on childhood malignancy following in utero radiation exposure is the Oxford Survey of Childhood Cancer registry.8 It consisted of >15 000 case-control pairs studied between 1943 and 1965. They reported a twofold increased risk of leukaemia and all childhood malignancies. The radiological studies were primarily abdominal plain X-rays and the findings were subject to recall bias in relation to timing of exposure. The subsequent UK Childhood Cancer Study (1976–1996) concluded that there was a slight but non-significant increase in the risk of all malignancies (OR 1.14 (0.9–1.45)) and for leukaemia (OR 1.36 (0.91–2.02)) with exposure to abdominal imaging in pregnancy.9 It is reported that the relative risk of childhood cancer after 50 mGy exposure is 2, which means that the probability of childhood cancer increased from 1/1000 to 2/1000.10 The findings from the Oxford study are at odds with those from children exposed to significantly higher environmental radiation doses in utero in Hiroshima and Nagasaki. This value of 50 mGy may be antiquated with time and possibly over-conservative. More recently, Ray et al. assessed the outcome from 1.8 million maternal child pairs between 1991 and 2007 exposed to major radio-diagnostic imaging modalities such as CT during pregnancy. The incidence of childhood malignancy was 1.13/10 000 person years in the exposed and 1.56/10 000 person years in the non-exposed groups. The study concluded that offspring exposed to major radio-diagnostic testing in pregnancy did not appear to be at higher risk of childhood malignancy when compared to the non-exposed group.11 The conflicting data can make counselling of pregnant women on carcinogenic risk with radiation exposure a challenge. The older data can appear alarming, whereas newer data is more reassuring. The most important information to impart is that the absolute baseline risk of dying from a childhood malignancy is exceedingly low (0.5–1.0/1000).12
As per the ACR Guidelines 2010, at a fetal radiation dose below 100 mGy there is no identified developmental delay and interruption of pregnancy is not warranted based on radiation effect.1 The consensus from the National Council on Radiation Protection and Measurements recognizes there is a low risk with fetal radiation exposure below 100 mGy. A threshold of 50 mGy (cumulative over the duration of the pregnancy) has been set empirically as the safety threshold for fetal radiation exposure. The majority of diagnostic imaging in current practice exposes the fetus to significantly lower doses than the recommended threshold level. Carcinogenic effects have never been conclusively observed for a dose <100 mGy. If clinically indicated, the benefit of a diagnostic image far outweighs any potential risks to the fetus.
Maternal Effects of Radiation
The principal concern with radiation exposure to the mother is the risk of carcinogenicity to the breast, particularly from computed tomography (CT). The breast during the pregnant state is a highly proliferative glandular structure and particularly radiosensitive. A CT pulmonary angiogram (CTPA) delivers a minimum dose of 20 mGy per breast to the average-sized woman. The dose delivered by CTPA can represent up to a 150-fold increase in the dose to the breast when compared to perfusion scintigraphy.13 In the long term, it is estimated that CTPA confers a 14% increased risk over the background risk for breast cancer in pregnant women <40 years of age.14 In contrast, a recent study by Ray et al. demonstrated no increased incidence of early breast cancer amongst 5859 women exposed to thoracic CT in pregnancy. The follow-up period was approximately 6 years. However, despite these reassuring findings, the authors did emphasize that a longer study duration is essential to determine the safety profile of CT in pregnancy.15
Review of Specific Diagnostic Imaging Techniques
Chest X-Ray
A plain X-ray of the chest renders exposure to a patient that is so low that the pregnant state should not alter the decision to proceed. In the third trimester, chest X-ray may expose the fetus to direct beam, given the proximity of the uterus to the diaphragm; however, when good technique is used, the dose remains exceedingly low (approximately 0.0005–0.01 mGy). The fetus is also less radiosensitive in the third trimester.
Computed Tomography Thorax
CT is an extremely useful and commonly used imaging modality in non-pregnant individuals. CT imaging is typically readily available in institutions and images can be rapidly acquired. The ACOG and ACR both recommend that CT imaging should not be withheld in pregnancy if the clinical scenario dictates. Where possible, the pregnant woman should be fully informed of the potential risks with ionizing radiation.1,16 The dose to the fetus during a CT thorax examination can be difficult to calculate as it cannot be directly measured. The dose delivered is dependent upon gestational age and proximity of the fetus to the scanned area of interest. The delivered dose is also dependent upon the thickness of the patient and the technique used. The dose to the conceptus is estimated based on both anthropomorphic phantom measurements and geometric phantom simulation methods. The Monte Carlo simulation is one such geometric phantom technique which estimates fetal dose as equivalent to that delivered to the uterus.17 It is, therefore, accurate in early gestation, but not necessarily with advanced gestational age. The principal limitations to these techniques is that the calculation dose is based on a uniform maternal habitus and static environment.18 As a result these techniques may over- or underestimate the delivered fetal dose. It is worth stating that in a CT of the thorax, the fetus is only exposed to scatter radiation, assuming appropriate planning of the examination. In early pregnancy, the distance between the directly irradiated region at the lung and the location of the embryo is generally at least 20 cm. The scattered radiation would be estimated to be no more than 1% of the chest dose.19 The maximum fetal dose from a chest CT, therefore, is estimated to be (0.1–0.66 mGy).20
A CTPA uses IV contrast along with computer processing to create tomographic images, with an intraluminal defect being diagnostic of a pulmonary embolus. CTPA is discussed in greater detail below. There is no available data to suggest potential harm to the fetus from exposure to iodinated contrast media (see belowwww.acr.org/-/media/ACR/Files/Clinical-Resources/Contrast%5FMedia.pdf). There is a risk, however of an anaphylactic reaction and renal impairment in the mother, similar to the non-pregnant woman.
Ventilation Perfusion Scintigraphy
Ventilation perfusion scintigraphy or V/Q scanning has been pivotal to the diagnostic management of pulmonary embolus in pregnancy for decades. A V/Q scan involves radioisotopes being infused and also inhaled. Gamma cameras are used to make a two-dimensional image and a mismatch between ventilated and perfused lung is diagnostic or gives a ‘high probability’ of a pulmonary embolus. The radiation dose delivered to the fetus and maternal tissue is estimated using body phantoms and software similar to techniques described in the previous section. The radiation dose delivered to the fetus is estimated at 0.32–0.74 mGy, which is slightly higher than that of CTPA. However, institutions can employ the use of perfusion-only imaging in the presence of a normal chest X-ray, which lowers the dose of radiation to the fetus to 0.1–0.37 mGy. Furthermore, if the perfusion scan is normal (no defects), there is no diagnostic reason to proceed with a ventilation scan. The maternal dose delivered to the breast is significantly lower than CTPA (0.11–0.3 mGy).20
For perfusion only imaging, 99mTc-labelled macro-aggregated human albumin particles are injected into a peripheral vein and are excreted via the kidneys. Ventilation scintigraphy is not typically necessary but if used, 99mTc-DTPA (diethylenetriaminepentacetic acid) is a commonly used radiotracer. It is absorbed via the lungs and excreted through the kidneys.19 Women are encouraged to frequently void post-imaging to decrease fetal exposure to radiotracer in the mother’s bladder.
Positron Emission Tomography Scan
Positron emission tomography (PET) scanning is a tomographic technique measuring the three-dimensional distribution of positron-emitting labelled radiotracers. The most commonly used tracer is 18F-labelled fluorodeoxyglucose (FDG). FDG is a glucose analogue taken up by living cells via cell membrane glucose transporters. FDG accumulates in tissue in proportion to the amount of glucose utilization and increased consumption is characteristic of malignancies. In the non-pregnant setting, PET imaging is a mainstay in the evaluation of several malignancies, including breast cancer and lymphoma.
Radiopharmaceutical delivery to the fetus is by two mechanisms. Firstly, there is irradiation from maternal organs where FDG accumulates, such as the bladder and secondly, there is internal irradiation from trans-placental spread. The approximate dose to the uterus is typically used in early gestation as an estimate of fetal absorbed dose. Animal studies indicate that FDG is transferred across the placenta and can accumulate in fetal tissue.21 To date in the literature, 20 pregnant women have been injected with FDG for clinical indications.22 These reports show that higher doses of the radioisotope are absorbed in early pregnancy when fetal cells are rapidly proliferating. The estimated dose from 18F-FDG PET is between 2 and 5 mGy.23
Anatomical and morphological information derived from PET integrated with CT or magnetic resonance imaging (MRI) can be used to improve the localization and characteristics of FDG-avid lesions. As expected, the estimated dose is higher with hybrid PET/CT than for PET imaging alone. For a whole body PET/CT the estimated fetal dose is between 10 and 20 mGy. PET/MRI imaging does not involve the use of ionizing radiation for attenuation correction and would be recommended in preference to PET/CT. The dose delivered to the fetus by PET/MRI is estimated at <5 mGy. Of note, the total radiation exposure to the fetus from PET is well below the threshold effect for deterministic effects. In a series of papers on gynaecological and haematological cancers in pregnancy published by the Lancet in 2012, the use of FDG PET was explicitly discouraged, based on the available animal data and paucity of data in humans.24,25 There is no formal recommendation by the ACOG, ACR, RCOG UK or SOGC on the use of PET in pregnancy. The European Association of Nuclear Medicine (EANM) recommend that the absorbed dose to the fetus can be minimized by reducing the amount of activity given to the patient and by facilitating removal of the radiotracer.26 Adequate hydration and frequent voiding of urine is recommended to reduce the radiotracer content in the maternal bladder. The doses of the median radiation exposure to the fetus from the discussed imaging modalities are highlighted in Table 20.2.
Imaging technique | Median radiation exposure to fetus (mGy) |
---|---|
Chest X-ray | 0.0005–0.01 |
Chest CT/CTPA | 0.01–0.66 |
Ventilation-perfusion (V/Q) scan | 0.1–0.8 |
Low-dose perfusion scintigraphy (‘Q’ only) | 1–0.37 |
FDG PET/CT | 9.4–21.9 |
V/Q vs CTPA for Diagnosis of Pulmonary Embolism in Pregnancy
In the most recent confidential inquiry into maternal deaths in the UK and Ireland (Dec 2017) thromboembolic disease retains its position as a leading cause of maternal deaths that are directly attributed to pregnancy.28 During all trimesters of pregnancy, the incidence of venous thromboembolic event is seven times higher than age-matched non-pregnant controls. In women immediately post-partum there is up to a 35-fold increase in venous thromboembolism (VTE) when compared to age-matched controls.15 The mortality rate associated with an untreated pulmonary embolism can be as high as 30%.29 The symptomatology of pregnancy itself includes dyspnoea and palpitations, which can mimic clinical symptoms of a pulmonary embolism. It is unsurprising therefore, that a common radiological request from physicians is to rule out a thromboembolic event in pregnancy. Fortunately the actual incidence of confirmed pulmonary embolic events is low at approximately 3%. Unlike the non-pregnant state, clinical suspicion typically necessitates a diagnostic radiological investigation, as D-dimers and clinical decision rules such as the Wells score are not validated in pregnancy.30 A low clinical suspicion will typically result in a negative diagnostic scan, but can result in unwanted radiation exposure, a possible reaction to contrast media and a cost to the healthcare system. Indeterminate results such as subsegmental pulmonary emboli can result in potentially unnecessary anticoagulation and have an implication for future pregnancy management and contraceptive choice.
A number of algorithms have been developed to assist clinicians in the work up of women with suspected VTE. Figure 20.1 details the algorithm adopted by the SOGC, which is similar to that of the American and UK professional bodies.2,3,31 The initial investigation of choice is compression ultrasound (CUS) of lower extremities. The image should extend to include the iliac veins as there is a higher incidence of pelvic thromboembolic events in pregnancy. The sensitivity of CUS is 94% for detection of a deep venous thrombosis (DVT). If detected, the patient should be anticoagulated and the need for further investigation is negated. If a clinical suspicion of a pulmonary embolism remains in the presence of a normal lower extremity ultrasound, further diagnostic investigation is warranted. A chest X-ray (CXR) should then be performed, not only as it dictates further imaging choice, but also as it can exclude alternative pathology such as pneumonia or a pneumothorax. The next diagnostic step can be a clinical conundrum. The choice of imaging in most institutions is between ventilation perfusion scintigraphy or CTPA. The SOGC and RCOG UK recommend that in the presence of a normal CXR, V/Q should be performed in preference to CTPA.31 The European Association of Nuclear Medicine (EANM) advocates for V/Q single-positron emission computerized tomography (SPECT) as the gold standard in the diagnosis of pulmonary embolism (PE) in pregnancy.33,34
Figure 20.1 Algorithm for the diagnosis of pulmonary embolism in pregnancy in women.
The advantages and disadvantages of both imaging modalities are described in Table 20.3. Both V/Q and CTPA confer a high negative predictive value of almost 100% with a low fetal radiation dose. A recent Cochrane review of imaging for exclusion of pulmonary embolism in pregnancy concluded that both CTPA and V/Q are appropriate.35 The review included 11 studies of 695 CTPA and 665 V/Q imaging results. Of the studies included, five reported on V/Q, two on perfusion-only scintigraphy, one on SPECT V/Q and four on CTPA. A total of 230 patients underwent CUS prior to either CTPA or V/Q, with only one DVT diagnosed. All patients included in the review had a CXR performed. The incidence of confirmed PE was low at 3.3%. The median negative predictive value (NPV) for both V/Q and CTPA was 100%. A recent survey conducted amongst practitioners in the UK revealed CTPA as the most commonly used modality in the setting of suspected PE in pregnancy, despite the guidelines advocating V/Q.36 A cited reason for the preference for CTPA was the lack of availability of nuclear imaging in institutions after typical working hours. A potential solution offered is to empirically start anticoagulation with low molecular weight heparin (if there is no contraindication) where the clinical suspicion is high, and conduct the V/Q scan the next working day.
Table 20.3 Commonly used imaging modality in clinical evaluation for pulmonary embolism
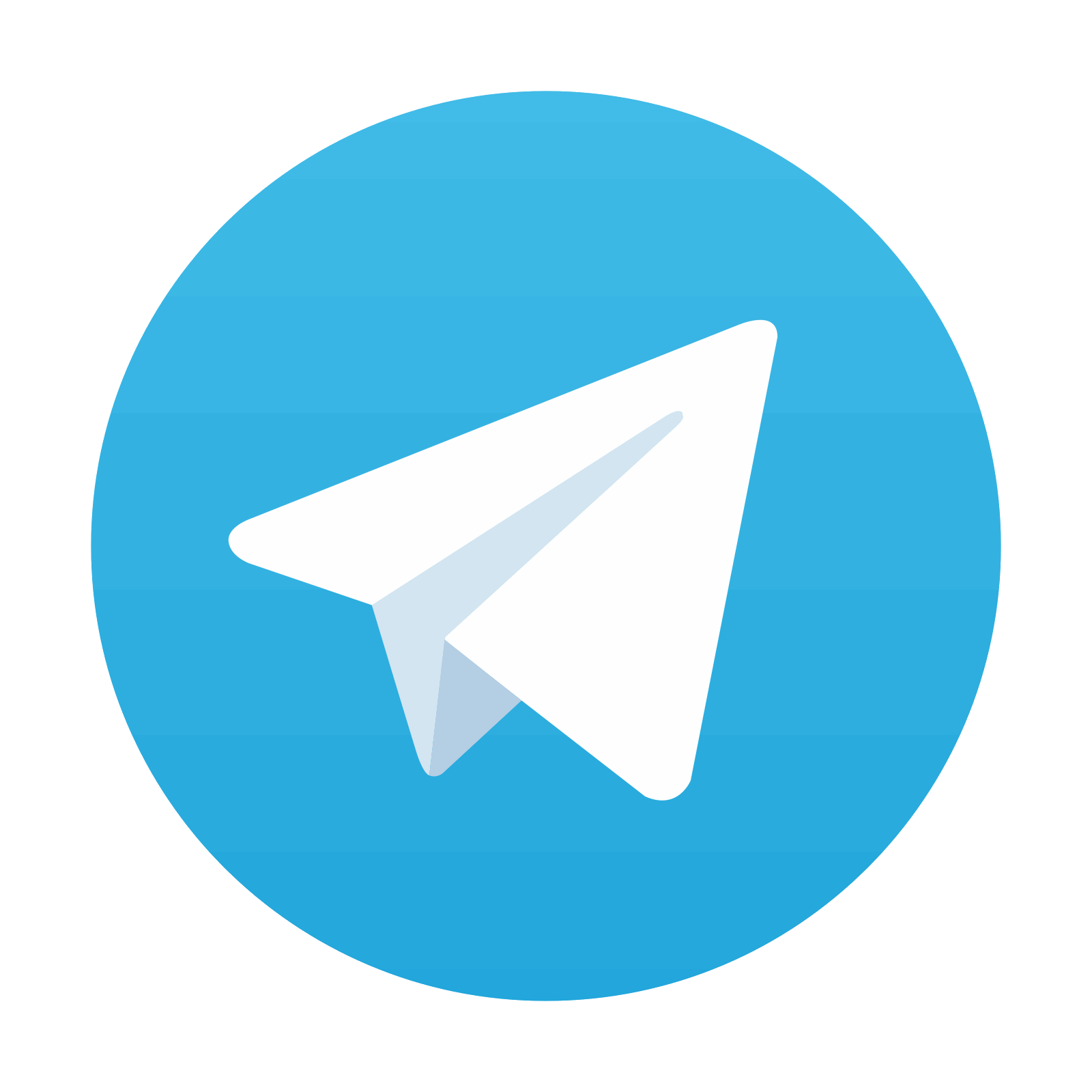
Stay updated, free articles. Join our Telegram channel

Full access? Get Clinical Tree
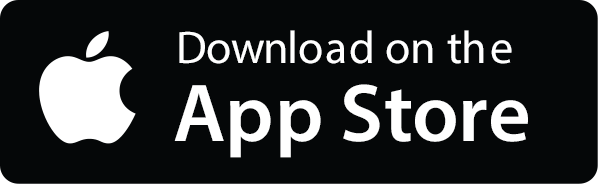
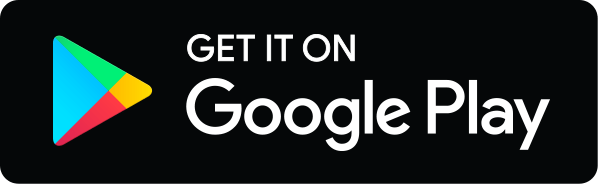
