Parity
Number of uteri
Mean weight (SD)
Maximum weight (gm)
Vertical length
Transverse
Anteroposterior
0
30
63.2 ± 21.4
110
7.7 ± 0.2
4.7 ± 0.1
2.9 ± 0.2
1
26
90.4 ± 39.8
170
8.6 ± 0.3
5 ± 0.2
3.5 ± 0.2
2,3
173
104.1 ± 36
210
9.2 ± 0.1
5.6 ± 0.1
3.9 ± 0.1
4,5
115
118.5 ± 42.2
243
9.4 ± 0.1
5.8 ± 0.1
4.2 ± 0.1
6+
117
125.7 ± 36.9
242
9.7 ± 0.1
5.9 ± 0.1
4.2 ± 0.1
However, when the analysis was repeated taking age into consideration, the average uterine weight did not vary with advancing age in nulligavidas (Table 5.2) or in women with any other parity, but variation was observed based on age when all patients were considered together. This suggested that parity rather than age was the primary determinant of uterine weight.
Table 5.2
The relation between uterine weight, dimensions and parity taking age into account
All uteri | Nulligravidas | ||||||
---|---|---|---|---|---|---|---|
Age range | Number of uteri | Mean weight ± SD for all group | Maximum weight | Vertical length | Transverse | Anteroposterior | Mean weight ± SD |
10–19 | 3 | 56 ± 13.9 | 65 | 8 ± 0.0 | 5 ± 0.3 | 2.8 ± 0.4 | 63 |
20–29 | 68 | 107 ± 34.5 | 225 | 9.2 ± 0.2 | 5.5 ± 0.1 | 4.1 ± 0.1 | 46 |
30–39 | 223 | 114.9 ± 36.1 | 240 | 9.4 ± 0.1 | 5.7 ± 0.1 | 4.1 ± 0.1 | 71 |
40–49 | 114 | 118.1 ± 44.7 | 243 | 9.5 ± 0.1 | 5.9 ± 0.1 | 4.2 ± 0.1 | 67 |
50–59 | 38 | 84.3 ± 41.9 | 189 | 8.1 ± 0.3 | 5 ± 0.2 | 3.2 ± 0.2 | 51 |
>60 | 15 | 56.1 ± 20.3 | 98 | 8 ± 0.5 | 4.5 ± 0.2 | 2.8 ± 0.2 | 54 |
The maximum weight of an apparently normal uterus also varied with age and parity and was almost fourfold higher compared to the mean weight of nulliparous women. In addition, the data suggested that parity not gravidity was the primary determinant of uterine weight. Thus pregnancies resulting in a miscarriage prior to viability did not result in permanent enlargement of the uterus. Thus determining the point at which a uterus would be considered abnormally large based on its weight becomes difficult. Based on estimates from the study population, Langlois (1970) proposed 130 g as the cut-off point for nulliparous women, 210 g for parity 1 to 3 and 250 g for higher parities [5]. Another observation made by Langlois (1970) is that uterine weight was lower in Caucasian compared to black women [5]. For example, the uterine weight for Caucasian nulliparous women was 49 ± 5.1 g and for nulliparous Black women was 78.3 ± 5.8 g. This difference was not present after the first pregnancy that reaches the age of viability (90.4 ± 8.7 g and 90.5 ± 13.2 g respectively). Some older writings employed the terms ‘subinvolution’, ‘uterine fibrosis’ or myometrial hypertrophy to describe the observation of symmetrically enlarged uteri that contain no gross or microscopic abnormalities. But given the variations in the size of the uterus described here, it becomes unclear how uterine size and myometrial hyperplasia can be considered within the definition of adenomyosis apart – perhaps – in the less frequently encountered extremes.
In a more recent study Esmaelzadeh et al. (2004) assessed uterine size using ultrasound in 231 healthy women [6]. The study group comprised 54 nulliparous and 177 multiparous women. For nulliparous women, the mean (SEM) uterine length, antero-posterior diameter and width were 72.8 mm (±1.3), 32.4 mm (±0.1) and 42.8 mm (±1.2) respectively. The corresponding figures for parous women were 90.8 mm (±1.1), 43.0 mm (±0.8) and 51.7 mm (±0.7) respectively. The measurements are in line with those reported by Longlois (1970) [5].
The Structure of the Myometrium
Myometrial Zones
The idea of myometrial zonation came into prominence with the advent of ultrasound and MRI imaging. Based on ultrasound appearance the normal myometrium can appear as having three distinct sonographic layers. The middle layer is the most echogenic and is separated from the thin outer layer by the arcuate venous and arterial plexus. The inner layer is hypo-echoic relative to the middle and outer layers (subendometrial or myometrial halo). The presence of adenomyosis can alter or distort the sonographic appearance of these zones [7–13].
The junctional zone (JZ) was shown to be hormonally dependent; being indistinct before puberty and after the menopause, and showing maximum increase in thickness in the second half of the proliferative phase [14, 15]. The normal JZ as seen in MRI or ultrasound is defined as being regular and ≤5 mm thick [16], and a JZ ≥12 mm is reported to be highly predictive of adenomyosis [17]. The diffuse homogenous low-signal-intensity seen in adenomyosis was attributed to smooth muscle hyperplasia; a recognised feature of the disease [18].
In women of reproductive age, three different zones may be identified within the uterus using MRI. The normal endometrium and endometrial secretions appear as a high signal-intensity type stripe on T2-weighted sagittal images. Immediately subjacent to this is a band of low signal intensity that represents the innermost layer of the myometrium: the Junctional Zone (JZ) that forms the outer boundary of the EMI [19]. The outer layer of the myometrium is of intermediate signal intensity. The thickness of the normal JZ varies considerably, ranging from 2 to 8 mm [20, 21]. Diffuse or focal widening of the JZ on MRI is suggestive of adenomyosis. Areas of low signal intensity are taken to correspond to smooth muscle hyperplasia and high signal intensity foci or linear striations are taken to represent ectopic endometrial tissue.
Hricak et al. (1983) published the first study on the use of MRI for the assessment of the female pelvis [22]. The study included 7 volunteers, 12 patients who had non-gynecological pathology and 2 women with gynecological disease. One participant was premenarcheal, 12 were of reproductive age (including two patients who had had a hysterectomy), 2 were postmenopausal and one was 8 weeks pregnant. Hricak et al. (1983) observed a low-intensity line separating the myometrium from the cyclic endometrium in women of reproductive age [22]. Their initial assessment was that this layer may represents the basal layer of the endometrium or that it relates to vascular or physiochemical phenomena at the myometrial-endometrial junction. They went on to suggest that assessment of this layer may allow the recognition of changes in the endometrium in relation to the menstrual cycle. In a following report, Lee at al. (1985) studied twelve uteri removed by hysterectomy [20]. These included 9 cases which did not have cancer or previous radiotherapy. They noted a 2–6 mm band of low-intensity signal between the central area of high intensity and the outer area of intermediate density. This area was clearly visible in 4 specimens, faintly visible in two cases and absent in three cases. Comparing endometrial thickness on histological examination with that measured on MRI, demonstrated that the high intensity central area corresponded to the endometrium, but there were no histological features that could explain the low intensity area and there were no histological differences between specimens which exhibited the low intensity zone and those specimens which did not. McCarthy et al. (1986) concurred with the conclusion that the junctional zone represents an area within the myometrium [23]. The explanation for the presence of the low-intensity area remains puzzling and its presence in uterine specimens suggests that it may not be related to the difference in blood flow. McCarthy et al. (1989) attempted to explain the presence of this area of low intensity by comparing the inner and outer myometrium. No differences were noted in the number of blood vessels, the content and nature of elastin, the amount of extracellular mucin and intracellular glycogen or the amount of collagen when comparing the junctional zone and the remainder of the myometrium [24]. However, there was a statistically significant difference in the water content. This was lower in the junctional zone compared to the endometrium and to the rest of the myometrium. As a percentage of weight, water constituted 82.88 % (SD ± 2.9) of the endometrium, 79.28 % (SD ± 1.4) of the junctional zone and 81.05 % (SD ± 1.8) of the myometrium. McCarthy et al. (1989) attributed the different signal intensity in the uterus to differences in water content as well as to differences in the relaxation phenomena that occur in heterogeneous tissue when imaged by MRI [24]. They also opined that other factors such as differences in blood flow do not need to be invoked to explain the phenomena.
Scoutt et al. (1991) compared the myometrial junctional zone and the outer myometrium after staining tissue section with Feulgen to delineate nuclei [25]. Five sections were examined per region utilizing the 20× objective light microscopy lens and image analysis. Sections were also stained for Collagen type III, IV and V; laminin; and fibronectin. Immunoreactivity was scored on a semi-quantitative score (from 0 to 3). The percentage of the nuclear area per high power field reported in this study was 61.7 % (SD ± 6.7) for the junctional zone and 21.3 % (SD ± 4.4) for the outer myometrium giving a JZ/OM ratio of 3.00 ± 0.61. On the other hand, there were no significant differences in the distribution of laminin; types III, IV, and V collagen; or fibronectin between the junctional zone and the outer myometrium. Scoutt et al. (1991) concluded that the decrease in T2 values observed in the JZ may be related to increase in cell number per unit volume with resultant decrease in extracellular matrix [25]. The findings are in line with the earlier observation of increased musculature in the inner myometrium compared to the outer layers [26]. Thus differences in composition between the inner and outer layers of the myometrium have been noted for more than half a century. Schwalm and Dubrauszky (1966) examined a total of 40 uteri representing various physiological states (pre- and post- menopausal and postpartum) and uteri removed surgically as well as uteri from cadavers to determine the percentage of musculature in each region of the uterine wall [26]. Uterine wall was divided into three layers (inner, middle and outer thirds). The muscle content was higher depending on the region examined. Thus it was higher in the uterine corpus compared to the isthmus and was lowest in the cervix and in each region the muscle content was higher in the inner followed by the middle and was lowest in the outer layers. The difference in muscle content between the inner and outer layer of the anterior-posterior wall of the uterine corpus was statistically significant.
However, a more recent study demonstrated that the transition from the inner to the outer myometrium is gradual with no line of demarcation that would correspond to the MRI appearance [27]. In addition, it should be borne in mind that the distinction between the myometrial layers is not a consistent finding on MRI and is absent in a good proportion of cases. Mehasseb et al. (2011) examined sequential high-power fields (hpf ×200; 124,403 μm 2 ) of the whole myometrial thickness from the endometrium to the serosa avoiding glandular tissue and large blood vessels [27]. Each field was assessed for the number of nuclei (nuclear count = n/hpf) as a reflection of cell density, the total nuclear area (i.e. area of the image occupied by nuclei, expressed as percentage), and the average nuclear size (μm2). The percentage area that expressed α-SMA per hpf was examined using image analysis as an index of the muscle mass. In premenopausal women, with or without adenomyosis, cell density, nuclear size, total nuclear area and muscle mass were significantly greater (p < 0.01) in the inner compared to the outer myometrium (Table 5.3). The same was noted in postmenopausal women, but the difference in nuclear size was not statistically significant [27]. In premenopausal uteri with adenomyosis, both the inner and outer myometrium featured lower cell density and larger nuclear size compared to controls (p < 0.05), and the total nuclear area was lower in adenomyosis compared to controls, but the difference was statistically significant in the inner but not the outer myometrium. Although not statistically significant, similar differences were noted in postmenopausal uteri. Examination of the full myometrial thickness through sequential high power fields showed that the reduction in cell density and total nuclear area starting from the inner through to the outer myometrium in both adenomyosis and unaffected control samples was gradual and there was no distinct zonation that would correspond to the demarcation seen on MRI [27].
Table 5.3
The characteristics of the inner (IM) and outer myometrium (OM) in control and adenomyotic uteri
Cell density, nuclear count (n/hpf) | Nuclear size (μm2) | Total nuclear area (%) | Muscle mass (%/hpf)c | ||
---|---|---|---|---|---|
Premenopausal control (n = 35) | IM | 1171 ± 52a | 24.81 ± 0.47a | 23.12 ± 1.17a | 65.01 ± 0.76a |
OM | 801 ± 43 | 23.44 ± 0.52 | 14.83 ± 0.8 | 42.6 ± 1.39 | |
Premenopausal Adenomyosis (n = 54) | IM | 970 ± 36a,b | 26.38 ± 0.27a,b | 20.54 ± 0.77a,b | 65.01 ± 0.76a |
OM | 625 ± 25b | 25.15 ± 0.33b | 12.76 ± 0.57 | 46 ± 1.25 | |
Post-menopausal control (n = 10) | IM | 1374 ± 53a | 24.6 ± 0.89 | 26.7 ± 1.51a | 57.27 ± 3.85a |
OM | 813 ± 55 | 24.5 ± 0.59 | 16.3 ± 1.23 | 39.35 ± 1.85 | |
Post-menopausal Adenomyosis (n = 10) | IM | 1106 ± 44a | 26.2 ± 0.72 | 23.4 ± 0.74a | 62.78 ± 2.45a |
OM | 778 ± 61 | 25.6 ± 0.84 | 16.2 ± 1.49 | 44.54 ± 5.61 |
Junctional Zone Contractility Function
The junctional zone (JZ) was shown to be hormonally dependent. On MRI, it is indistinct before puberty and after the menopause and showing maximum increase in thickness in the second half of the proliferative phase [14, 15]. Studies using video-sonography have demonstrated peristaltic waves confined to the JZ myometrium. These waves vary during the cycle [28]. JZ contractions during the late proliferative phase may have a role in sperm transport, whilst quiescence during the secretory phase may facilitate implantation [29].
Studies using ultrasound scan and radioisotope scintigraphy reported that the subendometrial myometrium has distinct contractile properties that varied with the phases of the normal menstrual cycle. Using ultrasound identified the contractions as antegrade (from fundus to cervix) during menstruation, and retrograde (from cervix to fundus) during in the rest of the cycle. It was suggested that these contractions have a role initially to facilitated sperm transport and subsequently to support blastocyst implantation [30, 31]. It was also speculated that inner myometrial contractility could help to control menstrual blood flow and that its disturbance might explain the occurrence of menorrhagia [32]. In the study by Fraser et al. (1986) there was one woman (age 24 years) who had severed bleeding leading to anaemia [33]. She had an enlarged uterus to 14 weeks size and was histologically confirmed on full thickness biopsy with pure myometrial hyperplasia. Indeed Benson et al. (1958) quoted Meyer R (1925) as the first to suggest altered uterine contractility as a mechanism of bleeding in adenomyosis [34, 35].
Using MRI and hysterosalpingo-scintigraphy (HSSG), endometriosis and adenomyosis were linked to hyperperistaltic and dysperistaltic utero-tubal transport, but reduced fertility was linked to adenomyosis in women with patent tubes [36]. This study should be interpreted with caution, first because of the unusually high incidence of adenomyosis and the lack of clear diagnostic criteria. Second; and perhaps most importantly, because of the test chosen to assess tubal function. It has been argued that many of the images produced by HSSG may be artefacts [37], and radioactive-labelled particle transport is inconsistent [38–40]. Habiba (1994) demonstrated that HSSG has a false negative rate of 34 % in the control group (n = 13 representing 26 tubes) who had patent tubes on laparoscopy and that the positive predictive value for HSSG for predicting tubal patency was 65 % and the negative predictive value for an obstructed fallopian tube was 42 % [37]. Wånggren et al. (2011) examined 99mTc-radio-labelled particle transport through the uterus and the tubes in ten women with proven fertility. Transport of radioactive particles could only be seen in some cases and most frequently during the periovulatory period [40].
Embryonic Origins
The endometrial-subendometrial unit has also been described in the literature as the “archimetra” (the endometrium of older phylogenetic origin) [41], with reference to Werth and Grusdew (1898), who used the term archimyometrium to describe the ontogenetically old character of the subendometrial myometrium [42]. A distinctive feature of the EMI is the lack of an intervening connective tissue layer, or a protective submucosa. As a result, the endometrial glands and stroma lie in direct contact with the myometrium, allowing extensive free interaction [43–45]. The EMI is also irregular over its entire surface [43].
Although neither Noe et al. (1999) nor Uduwela et al. (2000) studied the embryological origin of the uterus, they stated that both EMI components (basalis endometrium and subendometrial myometrium) are believed to have a common embryological origin from the paramesonephric ducts whereas the outer myometrium is of non-paramesonephric mesenchymal origin [45, 46]. There are few early studies of the subject. Konishi et al. (1984) examined autopsy material obtained from human abortuses and stillborn fetuses obtained at different gestational ages (12–40 weeks) [47]. They observed that the outer part of the mesenchyme of the uterus gives rise to the myometrium and that the inner part corresponds to the endometrial stroma [47]. The Mullerian (paramesonephric) origin of the outer myometrium is also supported by Robboy et al. (1982) who described the normal development of the human female reproductive tract and the alterations resulting from experimental exposure to diethylstilbestrol [48].
Noe et al. (1999) argued that the endometrium including both the glandular and stromal component together with the subendometrial myometrium form a unit which they termed the ‘archimetra’ [46]. They argued that the archimetra is derived from the paramesonephric ducts and the surrounding mesechyme whilst the stratum vasculare and the stratum suravasculare develop later and do not share the same embryonic origin as the archimetra. This view contrasts with electron microscopy studies which suggested that the development of inner myometrial layers from undifferentiated stromal cells at the endometrial-myometrial junction [47]. Whilst there appears to be agreement that the stratum vasculare does not develop from the archemyometrium (Lebedev 1952; quoted from Wetzstein 1965) [49, 50], it is unclear if the layers of the myometrium do have different origins. In the study by Wetzstein (1965), the direction of the muscle bundles in the inner myometrium was predominantly circular [49]. In the largest part of myometrium or the stratum vasculare, muscle bundles were interwoven in random directions forming a meshwork. Bundles were shown not to run the whole length of the uterus but to lose and gain additional bundles along their path and have also been shown to change direction abruptly. The outermost stratum supravasculare was formed of four layers: a longitudinally directed outermost layer followed by a circular layer, an incomplete longitudinal layer and an innermost circular layer. Wetzstein (1965) also noted that there was clear interconnectivity between all the layers of the myometrium [49]. Muscle bundles were also anchored to the vasculature. Experimental observations from early uterine development in mice suggest that uterine layers evolve through a process of differentiation from the undifferentiated mesenchyme that surrounds the endometrial epithelium [51, 52]. The mesenchymal cells that will form the endometrial stroma and both the inner and outer myometrium could be identified in the very early phases.
Mehasseb et al. (2009, 2010) described uterine development in the neonatal mouse [51, 52]. On day 2, the uterine cavity consisted of an oval-shaped lumen, elongated in the mesometrial anti-mesometrial axis. The luminal epithelium consisted of a monolayer of low columnar cells. This was surrounded by mesenchymal cells that did not form distinct layers or have distinct orientation. The perimetrium was composed of a single layer of epithelium. By day 5, the mesenchymal cells started to segregate into three layers: endometrial stroma, inner circular, and prospective outer longitudinal muscle layers. The endometrial stroma formed the inner half of the uterine wall thickness. Stromal cells retained their undifferentiated shape. The prospective inner circular muscle layer was the most defined layer and was five to six cells thick. This was formed of circularly orientated and tightly packed cells. The prospective outer myometrium was formed of one to two layers of cells retaining their undifferentiated appearance. Vascular spaces started to appear especially between the inner and outer myometrium.
By day 10 all layers were more distinct. The stroma appeared more tightly packed. The inner circular muscle layer was organized into bundles. By day 15 the adult configuration of the uterus became apparent. Stromal cells were randomly orientated except around the individual glands. A distinct loose vascular layer separated the inner and outer myometrial layers. The outer myometrial cells became grouped in bundles connected by loose connective tissue sheaths, and separated from the inner myometrium by a distinct loose vascular layer.
The Expression of ECM Components
McCarthy et al. (1989) compared histological sections of the inner and outer myometrium for the number of blood vessels, smooth muscle cells, fibroblasts, elastin, iron, collagen, mucin, polysaccharide and amyloid [24]. There were no significant differences between the myometrial layers. In a subsequent study, the same group [25] compared the density of the extracellular matrix components using immunohistochemical staining with antibodies to type III, IV and V collagen; laminin, and fibronectin. They used a semiquantitative score (from 0 to 3+). Immunohistochemical analysis demonstrated a normal distribution of extracellular matrix components and no difference in distribution between the inner myometrium and outer myometrium in women without adenomyosis.
Metaxa-Mariantou et al. (2002) studied elastin distribution in the myometrium during the phases of the menstrual cycle using immunohistochemistry, orcein staining and image analysis [53]. Elastin was noted in arteries and arterioles and within the perivascular tissue in the myometrium. In the endometrium, elastin was present in the basal portions of the spiral arterioles. No elastin was found in the more superficial parts of the vascular tree or in the endometrial stroma. Within the smooth muscle of the inner myometrium, elastin was absent or of low abundance. In the outer myometrium, elastin was also noted within smooth muscles. Thus in contrast to the study by McCarthy et al. (1989), Metaxa-Mariantou et al. (2002) noted a decrease gradient from outer to inner myometrium [24, 53]. There was no distinct demarcation between the inner and outer myometrium. An interesting observation made by Metaxa-Mariantou et al. (2002) is that elastin was expressed in endoemtrial basal layer in 3 out of the 12 samples exposed to the Levonorgestrel Intrauterine Device (LNG-IUS, Mirena®), but not in any samples not exposed to LNG-IUS [53]. This suggests that exposure to progestogens may be associated with the acquisition of a myofibroblastic phenotype by endometrial stromal cells. This seems to support the observation by Kohnen et al. (2000) that some stromal cells in the basal endometrium express α-smooth muscle actin and thus exhibit a myofibroblastic phenotype in respose to progestogens [54]. Leppert and Yu (1991) used scanning electron microscopy and reported that the extracellular matrix of the myometrium contains flat sheets or lamellae within a sponge-like matrix [55]. Uterine elastic fibres were formed into two distinct structures: fibrils and thin sheets of elastic membranes. Isolated fibres and membranes formed thin sheets of elastic membranes and elastic fibrils that may allow the uterus to maintain elasticity without exerting excess pressure on the growing fetus. The elastic tissues in the non-pregnant human uterus had no specific architectural arrangement and formed a sponge-like structure. This contrasts with the elastic fibres of the cervix which formed membranes and fibrils, organized into fishnet-like structures. The concentration of insoluble elastin and collagen in human uterine body was 1.38 % and 38.8 % of dry-defatted tissues, respectively. No similar studies have been conducted focussing on adenomyosis [55].
Zheng et al. (2006) used histochemical staining for elastin (orcein and modified Victoria blue/ethanol solution PPA-VB) in uteri with and without leiomyomas, adenomyosis and adenomyoma [56]. They reported that expression of elastin within the myometrium was largely present in perivascular tissue particularly near larger vessels in the outer myometrium. Scattered elastic fibres were also present between the myometrial fibres in the outer myometrium. There was a trend for higher elastin density in older women. Staining showed a decreasing gradient from the outer to the inner myometrium but interestingly, expression was absent in fibroids, adenomyosis or adenomyomas. There are no studies that examined the effect of parity on the distribution of elastin. These findings contrasts with those of McCarthy et al. (1989) referred to above [24].
Steroid Receptor Expression in the Myometrium with and without Adenomyosis
Scharl et al. (1988) reported on estrogen receptor expression in the uterus including the myometrium through the menstrual cycle [57]. They observed strong estrogen receptor (ER) expression in the majority of nuclei in the myometrium during the proliferative phase. The expression level did not vary during this phase but ER expression reduced significantly following ovulation. More than 50 % of myometrial cells in mid- and late- luteal phases were ER negative, and the rest were usually weakly stained. They also reported that the distribution of ER positive and ER negative muscle cells was not diffuse but was arranged in receptor positive and receptor negative muscle bundles, and that expression was lower in the subserosal myometrium compared to the subendometrial myometrium. The observations are interesting, but it is important to keep in mind that the number of samples examined per phase of the cycle varied from a maximum of 6 in the early proliferative phase, 5 in the late proliferative, 3 in the early secretory, 3 in the late secretory and only one sample representing the mid-secretory phase.
Mertens et al. (2001) examined the expression of androgen, estrogen and progesterone receptors in the uterus utilising full thickness biopsies [58]. Five sections were examined in each of the phases of the cycle. Immunostaining took into account the percentage of stained cells and staining intensity. In the myometrium, ER expression was at its maximum during the early proliferative phase and decreased markedly in the early secretory phase. The expression of progesterone receptor did not vary with the phase of the cycle (Table 5.4).
Table 5.4
The distribution of steroid receptors in the myometrium throughout the menstrual cycle [58]
Menstrual cycle phase | Estrogen receptor | Progesterone receptor | Androgen receptor |
---|---|---|---|
Menstrual | 91 ± 46 | 199 ± 22 | 70 ± 5 |
Early proliferative | 132 ± 38 | 193 ± 37 | 66 ± 9 |
Late proliferative | 92 ± 27 | 193 ± 35 | 56 ± 16 |
Early secretory | 22 ± 5 | 188 ± 40 | 49 ± 20 |
Mid secretory | 60 ± 4 | 175 ± 27 | 33 ± 7 |
Late Secretory | 18 ± 21 | 214 ± 9 | 0 |
Kawaguchi et al. (1991) reported that estrogen receptor is expressed in the myometrium during the proliferative phase of the cycle, but is suppressed during the secretory phase whilst there was strong progesterone receptor expression during both phases of the cycle [59]. The findings are consistent with the observation that estrogen induces the expression of both estrogen and progesterone receptors during the proliferative phase. But, whilst progresterone suppresses its own receptor in the endometrium, the same was not observed in the myometrium. The study by Snijder et al. (1992) reported that estrogen receptor expression in the myometrium fluctuates during the menstrual cycle [60]. Five samples were examined in each phase. Maximum estrogen receptor expression was noted in the late proliferative phase followed by a sharp drop in the early secretory phase. The pattern was similar to that noted in endometrial stroma, but contrasted with expression in endometrial glandular epithelium where the decreased expression from the late proliferative and into the secretory phase was gradual. Progesterone receptor expression in the myometrium was strong throughout the phases of the cycle and showed little fluctuation. Thus the pattern of receptor expression in the stroma of the functionalis, basalis and the myometrium was similar and shows little fluctuation. Neither Kawaguchi et al. (1991) or Snijder et al. (1992) made a distinction based on myometrial zonation [59, 60]. Lessey et al. (1988) used immunohistochemistry to examine the expression of estrogen and progesterone receptors in the uterus in 33 premenopausal women. Samples were classified according to the phases of the menstrual cycle into menstrual (n = 4), early proliferative (n = 9), late proliferative (n = 7), early secretory (n = 5) and late secretory (n = 8) [61]. The expression of estrogen receptor peaked in the late proliferative phase in the endometrial glands and stroma but in the myometrium, estrogen receptor expression peaked in the early proliferative phase. During the secretory phase, expression declined rapidly in all layers. In contrast to estrogen receptor, progesterone receptor expression increased in the endometrium throughout the proliferative phase but dropped rapidly in the glands but not in the stroma during the secretory phase. Also, progesterone receptor expression in the myometrium paralleled the pattern in the stroma not the glands. Thus, there was a divergence in progesterone receptor expression in the endometrial epithelium on one hand and the stroma and myometrium on the other. However, Lessey et al. (1988) do not provide statistical analyses comparing expression in the various layers [61]. Also, it appears that the analysis was confined to the inner layers of the myometrium.
Amso et al. (1994) reported that the intensity of estrogen receptor expression in the myometrium was moderate throughout the cycle except in the late follicular phase when it was very strong, and that contrary to the slight fluctuations seen in ER, the intensity of progesterone receptor expression was strong throughout the cycle [62]. The study however was of poor quality because of the small sample size as each cycle phase was represented by only one or two samples. In this study only the superficial myometrium was assessed.
A more recent study by Noe et al. (1999) using immunohistochemistry of the whole uterine wall, took into account myometrial zonation [46]. The myometrium was divided into three zones: the stratum subvasculare or subendometrial myometrium adjacent to the endometrium with a predominantly circular muscular fibres; the subserosal stratum supravasculare with a predominantly longitudinal muscular fibres; and the stratum vasculare, which consists of a three-dimensional mesh of short muscular bundles that form the bulk of the uterine muscular wall. The expression of estrogen receptor in the subendometrial myometrium almost completely paralleled the expression in the endometrium. The immunoreactivity in the inner portion (about the inner third) of the stratum vasculare exhibited a reduced cyclical pattern, whilst the outer two thirds of the stratum vasculare and the stratum supravasculare showed no cyclic pattern. It is notable that the outer layers of the myometrium exhibited strong ER expression in all the phases of the cycle. The expression of progesterone receptor was reported as showing cyclical changes in the subendometrial myometrium and in the inner third of the stratum vasculare, but there were no cyclical changes in the outer portion of the stratum vasculare or in the stratum supravasculare. Again, the outer layers of the myometrium exhibited strong PR expression in all the phases of the cycle. Both ER and PR were highly expressed in all layers on the myometrium in postmenopausal samples suggesting that the receptors are constitutive for the myometrium.
Richards and Tiltman (1995) also examined the expression of estrogen receptor in the myometrium [63]. They reported that the number of myometrial cells per high power field (hpf ) was lower in the outer myometrium compared to the inner myometrium. In the fundal region, the total number (±SD) of cells per HPF was 111.7 (SD ± 12.66) in the subendometrial myometrium, 60.55 (SD ± 13.51) in the midmyometrium and 36.75 (SD ± 10.61) in the subserosal myometrium. The corresponding figures in the lower uterine segment were 105.27 (SD ± 20.8), 55.53 (SD ± 11.66) and 37.53 (SD ± 6.29) respectively. Whilst the number of estrogen receptor positive cells in the fundal region was 88.15 (SD ± 12.06) in the subendometrial myometrium, 39.7 (SD ± 15.25) in the midmyometrium, and 16.7 (SD ± 7.38) is the subserosal myometrium. The corresponding figures for receptor expression at the level of the lower uterine segment were 94.13 (SD ± 24.37), 32.4 (SD ± 8.77) and 18.67 (SD ± 7.83) respectively. Thus there were fewer estrogen receptor positive myometrial cells both in absolute terms and as a percentage of all myometrial cells in the outer layers of the myometrium. In addition, it is to be taken into account that the values quoted here from the study by Richards and Tiltman (1995) did not divide uteri based on cycle phase [63]. Their observation are thus at variance with those reported by Noe et al. (1999) [46]. This may be explained because the studies reported using different methodologies. Noe et al. (1999) relied on calculating an immunoreactive score that takes into account the percentage of stained cells for each intensity where intensity was graded as 0 = no, 1 = weak, 2 = moderate and 3 = strong [46]. But whilst the semiquatitive score has not been shown to reflect a doubling or tripling of receptor expression, it is unclear if the colorimetric measure is of biological significance. Richards and Tiltman (1995) calculated the percentage of estrogen receptor + ve cells and also included assessment of receptor concentration by radioimmunoassay and expressed as femtomoles per milligram of cytosolic protein [63]. The mean (±SD) receptor concentration in the subendometrial myometrium, midmyometrium and subserosal myometrium was 31.64 (SD ± 13.99), 14.01 (SD ± 6.78) and 9.50 (SD ± 4.28) respectively.
More recently, Mehasseb et al. (2011) reported on receptor expression in the myometrium taking into account both estrogen receptors α and β (ER-α and ER-β) and both progesterone receptors A and B (PR-A and PR-B) comparing women with and without adenomyosis and full thickness uterine wall biopsies [64]. All samples were standardised from the anterior wall of the uterus near the fundus and adenomyosis was defined by the presence of endometrial glands and stroma deeper than 2.5 mm below the endometrial-myometrial interface. The included specimens were classified according to the phase of the menstrual cycle into early, mid-, late- proliferative and early, mid-, late-secretory phases.
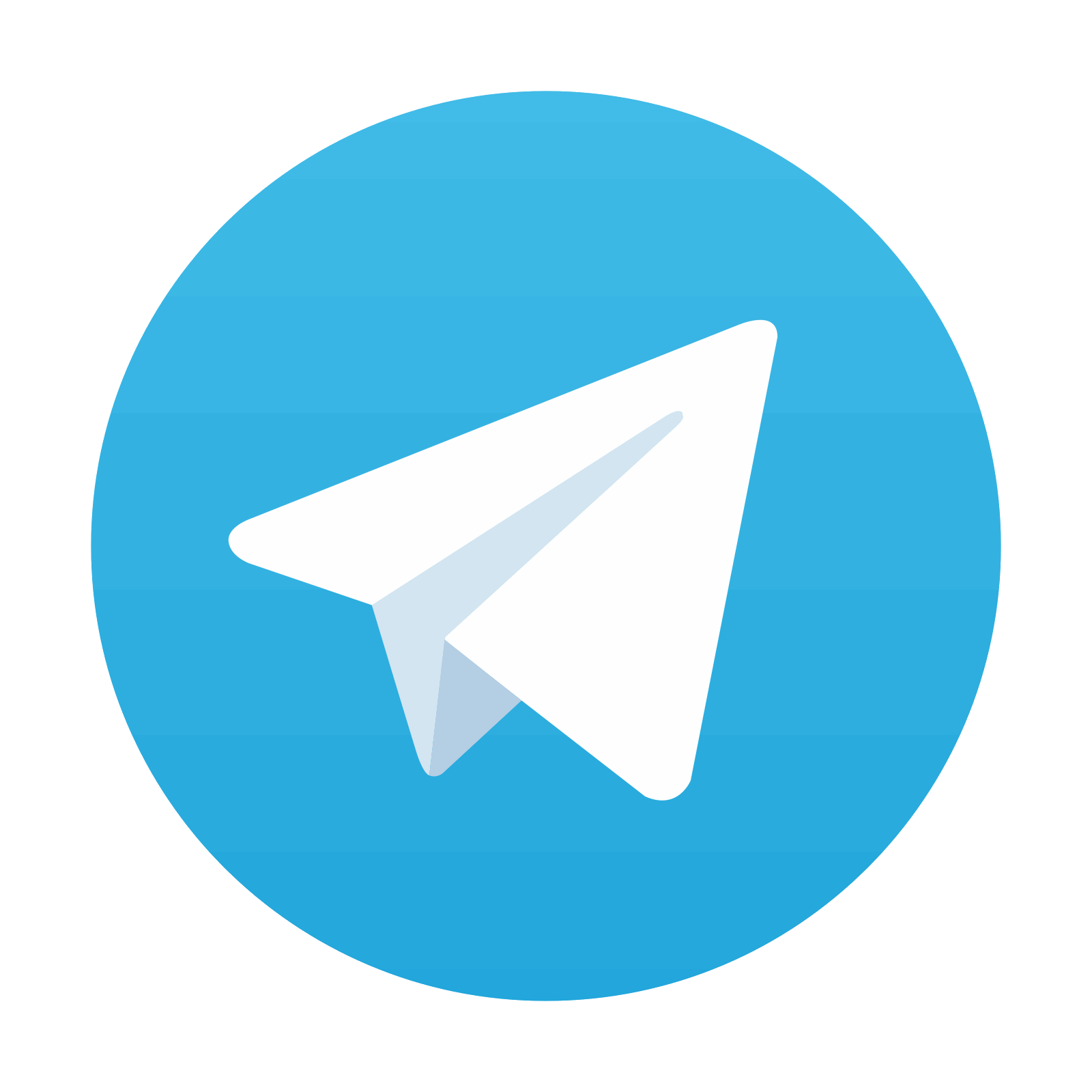
Stay updated, free articles. Join our Telegram channel

Full access? Get Clinical Tree
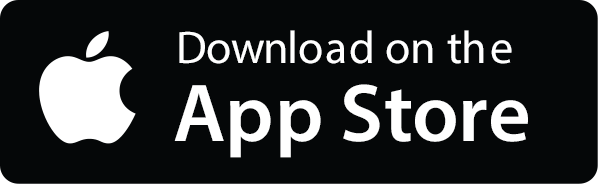
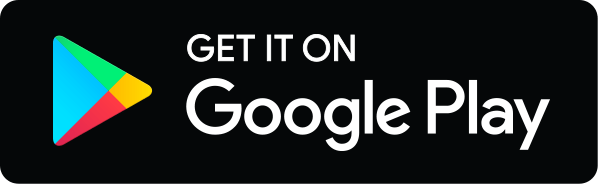