Fig. 16.1
Luteinizing hormone and human chorionic gonadotropin molecules. (a) LH is a glycoprotein with two subunits, the alpha subunit (red), similar to that of FSH and hCG with two carbohydrate attachment sites, and the beta subunit (blue), with only one carbohydrate attachment site. The light blue balls represent the carbohydrate chains. (b) hCG is similar in its structural attributes to LH. A notable exception is the presence of a long carboxyl terminal segment that is O-glycosylated (O-linked CHO), conferring longer half-life to hCG. The alpha and beta subunits are represented in red and blue strands, respectively, whereas the light blue balls represent the carbohydrate chains (Adapted from Leão and Esteves [3])

Fig. 16.2
Glycosylation patterns of LH and hCG. The alpha subunits of each hormone are identical in amino acid sequence and contain two sites of N-linked glycosylation. The beta subunit confers hormone specificity and contains variable amounts of N-linked glycosylation. LH beta subunit contains a single site of N-linked glycosylation, while hCG beta subunit contains two sites of N-linked glycosylation. In addition, hCG has an extended C-terminal that contains four sites of O-linked glycosylation
LH binds to a subgroup of G protein-coupled receptors with 7 transmembrane domains and a large N-terminal extracellular region (Fig. 16.3) [12, 13]. Receptor activation requires that hormones bind to the N-terminal region, thus leading to intramolecular signal transduction from the ligand–receptor complex to the transmembrane domains. Although the mechanism that underlies this intramolecular signaling pathway is not fully understood, it involves stimulation of adenyl cyclase via coupling to Gs proteins [12, 13]. Unlike FSH receptors that are expressed exclusively in the granulosa cells (GC), LH receptors are expressed in both GC and theca cells. The LH receptor expression is at its maximum in the GC of preovulatory follicles, but antral follicles with 3–10 mm in diameter have already expressed these receptors at approximately 10 % of the maximum [14].


Fig. 16.3
LH/hCG receptor. LH receptors are included in the subgroup of G protein-coupled receptors. Such receptors are characterized by having 7-transmembrane domains and a large N-terminal extracellular region, which is the predominant site of hormone binding
16.2.1 The Role of LH on Ovarian Steroidogenesis
The two-cell system, first proposed by Falck in 1959, is based on the assumption that while FSH receptors are present only in the GC, LH receptors are present in the theca cells and absent in the GC during the early follicular stages [15–18]. Theca cells are characterized by exhibiting steroidogenic activity in response to LH stimulation. Specifically, cholesterol is converted into androgens (i.e., testosterone and androstenedione) by transcription activities of cholesterol side-chain cleavage enzyme (P450scc), P450c17, and 3β-hydroxysteroid dehydrogenase (3β-HSD) genes. The starting point of steroid biosynthesis is cholesterol, a carbon 27 (C27) steroid. Cholesterol is converted to pregnenolone (C21) by P450scc (CYP11A – cytochrome P450, family 11, subfamily A, polypeptide 1), whose regulation is mediated by steroidogenic acute regulatory protein (StAR). StAR facilitates the influx of cholesterol into the mitochondria where P450scc is located. StAR expression is enhanced by cAMP and by stimulation of GC with FSH and LH or hCG [3, 4, 18, 19].
The primary route of pregnenolone metabolism is via the delta 5 pathway, the first two steps of which are driven by the same enzyme, CYP17 (P450c17). The hydroxylation of pregnenolone at the C17a position forms 17-hydroxypregnenolone, and the subsequent removal of the acetyl group forms the androgen precursor dehydroepiandrosterone (DHEA). Accordingly, CYP17 has both hydroxylase and lyase activity. Lastly, DHEA is converted to androstenedione by 3β-HSD [15, 19, 20]. A secondary route of metabolism involves the conversion of pregnenolone to progesterone by the action of 3β-HSD via the delta 4 pathway. Progesterone is then converted to 17-hydroxyprogesterone by CYP17 (Fig. 16.4) [20]. Importantly, CYP17 is located exclusively in thecal and interstitial cells, the extrafollicular compartment of the ovary, whereas CYP19 (aromatase), that converts androgens to estrogens, is expressed exclusively in GC, the intrafollicular compartment [20–22]. Thus, aromatization of androgens to estrogens is a distinct activity within the granulosa layer induced by FSH via activation of the P450 aromatase (P450arom) gene. Androgens produced in the theca layer must therefore diffuse into the granulosa layer to be converted to estrogens (Fig. 16.5). Hence, increasing levels of estradiol in the peripheral circulation during the follicular phase reflect the release of estrogen from granulosa cells into blood vessels [15, 22]. Theca and granulosa cells also secrete peptides that act as both autocrine and paracrine factors. Insulin-like growth factor (IGF) is secreted by theca cells and enhances LH-mediated androgen production within the thecal compartment as well as FSH-mediated aromatization in granulosa cells [15]. Inhibin and activin are produced in the granulosa cells in response to FSH and modulate the expression of steroidogenic enzymes, especially P450c17 in theca cells. While inhibin enhances androgen synthesis, activin has an opposite effect. Activin also has the important autocrine role of enhancing FSH action mainly by increasing the expression of FSH receptors [15] (Fig. 16.6).




Fig. 16.4
Human ovarian steroidogenesis. The starting point for steroid biosynthesis is the conversion of cholesterol in pregnenolone by P450scc. One route of pregnenolone metabolism is the delta-5 pathway (red arrows) by the action of CYP17 (P450c17). Hydroxylation of pregnenolone at the C17a position forms 17-hydroxypregnenolone, and subsequent removal of the acetyl group forms the androgen precursor dehydroepiandrosterone (DHEA). Another route of pregnenolone metabolism is the delta-4 pathway (purple arrows) in which pregnenolone is converted to progesterone by the action of 3b-HSD (an irreversible conversion). Progesterone is then converted to 17-hydroxyprogesterone by CYP17. In humans, 17-hydroxyprogesterone cannot be further metabolized. Aromatization of androgens to estrogens is a distinct activity within the granulosa layer induced by FSH via activation of the P450 aromatase (P450arom) gene (From Leão and Esteves [3])

Fig. 16.5
The “two-cell” system. FSH receptors are present exclusively in the granulosa cells. LH receptors are present in the theca cells and initially absent in the granulosa cells. In response to LH, theca cells convert cholesterol to androgens (testosterone and androstenedione). CYP17 is located exclusively in thecal cells, whereas CYP19 (aromatase) is expressed only in the granulosa. Thus, androgens must diffuse into the granulosa layer to be converted to estrogen via aromatization induced by FSH. Both FSH and LH act via AMPc production. In the late follicular phase, FSH induces LH receptor formation in the granulosa cells, which acquire LH responsiveness. In the granulosa, LH enhances FSH action (increasing estrogen production)

Fig. 16.6
Modulation of steroidogenic enzymes. In the early follicular phase, inhibin and activin are produced in the granulosa cells in response to FSH. They have important paracrine functions to modulate the expression of steroidogenic enzymes, especially P450c17 in theca cells. Inhibin enhances LH function, thus stimulating androgen synthesis to latter aromatization to estrogen in the granulosa, whereas activin suppresses androgen synthesis. Activin has also an important autocrine role of enhancing FSH action, especially by increasing the production of FSH receptors. Production of inhibin by the granulosa cells is increased in the late follicular phase while activin is decreased, with a positive effect on androgen production by theca cells. FSH induces LH receptor formation in the granulosa cells, which acquire LH responsiveness and therefore less FSH dependence. In granulosa, LH enhances FSH action that in turn increases estrogen production, initiates progesterone production (negatively modulated by activin), and control granulosa production of inhibin. The increase in inhibin, in turn, suppresses FSH secretion by the pituitary, important to ensure the dominance of the follicle
LH also acts in GC to stimulate progesterone production. Most circulating progesterone (~95 %) is produced in the intrafollicular compartment by the granulosa cells via the action of 3b-HSD that catalyzes conversion of pregnenolone (delta-4 pathway) under the LH influence (see Fig. 16.4) [18, 22]. Despite a marked increase in progesterone levels measured at the veins of the active ovary in the mid-follicular phase, peripheral concentrations increase only slightly probably due to active liver metabolism [23]. Progesterone can be further converted to 17-hydroxyprogesterone by CYP17 (via delta-4 pathway). However, very little 17-hydroxyprogesterone is converted to androstenedione, since human CYP17 catalyzes this reaction at only 3 % of the rate for the conversion of 17-hydroxypregnenolone to DHEA [18, 22, 24]. Therefore, 17-hydroxyprogesterone is basically the final product of the delta-4 pathway in humans. Moreover, progesterone itself cannot be metabolized in the GCs because CYP17 is not expressed within this cell compartment; as such, progesterone is the final product of the delta-4 pathway in the intrafollicular compartment and cannot be converted to estradiol in the GC under the effect of LH [20]. The preovulatory rise in progesterone facilitates the positive feedback action of estrogen on the pituitary; the latter is the key factor to induce the midcycle LH peak in the natural cycle. Progesterone also stimulates a midcycle FSH surge, important to support the full expression of LH receptors at the granulosa layer [22, 25].
In summary, ovarian steroidogenesis is the result of combined LH and FSH stimulation of the two cell types, theca and granulosa, influenced by autocrine and paracrine factors.
16.2.2 The Role of LH on Follicular Maturation and Luteal Phase Support
In the mid-follicular phase, FSH induces LH receptor expression in the granulosa cells of developing follicles [26]. The action of LH on its receptors activates cyclic AMP-protein kinase A (cAMP/PKA) pathway, which represents an additional stimulus to follicular growth [27]. Thereby, the maturing follicle also reduces its dependency on FSH by acquiring LH receptors and LH responsiveness [26–30]. FSH and LH cooperate in inducing the local production of the soluble molecule inhibin B and growth factors. Among these, insulin growth factors (IGF) I and II, which are expressed by both granulosa and theca cells throughout folliculogenesis, are important in promoting follicular maturation [31, 32]. Furthermore, LH exerts an antiapoptotic effect on the GCs, mediated by the production of fibroblast growth factors that maintain calcium homeostasis and granulosa cell viability by stimulating calcium efflux via a protein kinase C (PKC) delta-dependent pathway [33]. Additional signaling pathways (e.g., AKT and ERK1/2 pathways) involve the expression of EGF-like growth factors that influence GC proliferation, differentiation, and survival (apoptosis blockage) [34, 35]. Lastly, aromatase expression and steroidogenic function via LH receptor activation are likely to involve cAMP/PKA, extracellular signal-regulated (ERK) 1 and 2, and AKT pathways, all playing a crucial role in the final stages of maturation of human oocytes and follicles [36, 37].
LH activity during the luteal phase is totally responsible for the maintenance and the steroidogenic activity of the corpus luteum [38]. LH is responsible for the upregulation of growth factors like vascular endothelial growth factor A [39, 40], which plays a dynamic role in luteal angiogenesis, and epidermal growth factor-like ligands, amphiregulin and epiregulin, which regulate apoptosis in luteinized human granulosa cells [34, 41–44]. Furthermore, LH stimulates expression of extragonadal LH receptors in the endometrium [45, 46] and production of cytokines involved in implantation [47].
Therefore, LH regulates both granulosa and theca cells and has a pivotal role in follicular development and maturation. In light of the aforementioned findings, we can conclude that (1) both gonadotropins contribute (via granulosa) to maintain the autocrine–paracrine system governing dominant follicle’s growth; (2) LH is crucial in sustaining FSH activity in the granulosa during intermediate–late stages of folliculogenesis; and (3) LH is critical for maintaining corpus luteum function during the luteal phase.
16.3 Rationale of LH Supplementation in Stimulated Cycles
The “LH window” concept, as outlined by Shoham in 2002, proposes that in the absence of a threshold level of serum LH, estradiol production will be insufficient for follicular development, endometrial proliferation, and corpus luteum formation [48]. This concept can be clearly observed in patients with hypogonadotrophic hypogonadism who do not achieve adequate steroidogenesis by stimulation with FSH alone, but resume sufficient estradiol production by LH supplementation [49]. Evidence therefore suggests that in reproductive cycles optimal follicular development occurs within a “LH window,” that is, above an LH threshold of 1.1 and below an LH ceiling of 5.1 IU/L [48, 49].
After pituitary suppression, still widely used in association with COS, residual circulating levels of endogenous LH are usually adequate to support multiple follicular growth and oocyte development in COS with gonadotropins devoid of LH activity [50, 51]. In fact, only 1 % of LH receptors need to be occupied to drive adequate ovarian steroidogenesis. Fair evidence indicates that most normogonadotropic women have sufficient levels of endogenous LH and do not require exogenous LH supplementation [52–54]. Despite of that, a recent large meta-analysis including a total of 40 RCTs and 6443 women aged 18–45 years found a small relative increase (estimate of 9 %) in clinical pregnancy rate in patients treated with of rec-hFSH plus rec-hLH versus rec-hFSH alone (RR 1.09; 95 % CI 1.01–1.18) [55]. More importantly, ovarian response to COS with FSH-only-containing gonadotropins has shown to be suboptimal in subsets of normogonadotropic women, including those with advanced reproductive age (≥35 years old) [56, 57], diminished ovarian reserve [54, 58], and highly suppressed levels of endogenous LH, in whom LH activity falls below the LH threshold [59–63]. In addition, a subgroup of normogonadotropic patients who had normal estimated ovarian reserve but suboptimal responses to FSH-alone stimulation has also been identified and termed “hyporesponders” [64–67].
Clinical evidence indicates that the aforementioned subgroups have less responsive ovaries in stimulated cycles with FSH, which could be explained by a wide range of factors, including reduced paracrine ovarian activity [68], genetically determined reduced LH bioactivity [69], reduced androgen secretory capacity [70], and decreased number of functional LH receptors [71]. Serum androgen levels, especially total testosterone (T), calculated free T, dehydroepiandrosterone sulfate, and androstenedione, decline steeply with age, with the decline of each being greater in the early reproductive years than the later decades [72, 73]. Hence, it has been hypothesized that such women would benefit from LH-containing gonadotropin preparations. Action of LH at the follicular level could promote an increase in ovarian steroidogenesis and androgen production for its later aromatization into estrogens, with a positive impact on the follicular milieu. Furthermore, LH has also a direct effect on follicular growth and maturation via different signaling pathways that positively impact oocyte quality [74].
16.4 Clinical Evidence Supporting LH Supplementation During COS in Selected Patients
16.4.1 Hypogonadotropic Hypogonadism
The European Recombinant Human LH Study Group investigated the efficacy of rec-hLH for supporting FSH-induced follicular development in hypogonadotropic hypogonadal women (LH levels of <1.2 IU/l; WHO group I anovulation) [49]. Thirty-eight patients were randomized to receive 0, 25, 75, or 225 IU/day of rec-hLH in addition to a fixed dose of rec-hFSH (150 IU/day). The authors found that rec-hLH was able to promote a dose-related increase in estradiol and androstenedione secretion by rec-hFSH-induced follicles. Serum concentrations on the last day of FSH administration were 65 ± 4, 195 ± 94, 1392 ± 585, and 2441 ± 904 pmol/L for E2 and 3.6 ± 0.9, 5.1 ± 1.3, 6.4 ± 1.3, and 6.7 ± 1.3 nmol/L for androstenedione in the patients treated with 0, 25, 75, and 225 IU rec-hLH, respectively. LH supplementation also increased ovarian sensitivity to FSH, as shown by the proportion of patients who developed follicles after the administration of a defined dosage of FSH. While only 12.5 % of the patients treated with FSH alone developed follicles, the proportion substantially increased, according to the varying doses of rec-hLH (42.8 % in 25 IU and 77.8 % and 80 % in 75 IU and 225 IU, respectively). Furthermore, follicles that had been exposed to rec-hLH showed an increased ability to luteinize after hCG exposure.
In the aforementioned study, a daily dose of 75 IU rec-hLH was effective in the majority of women in promoting optimal follicular development (defined as > or = 1 follicle > or = 17 mm; E2, > or = 400 pmol/L; midluteal phase progesterone, > or = 25 nmol/L) and maximal endometrial growth. Lastly, rec-hLH was shown not to be immunogenic and was well tolerated by the patients.
In conclusion, exogenous LH activity supplementation is mandatory in stimulation protocols applied to women with hypogonadotropic hypogonadism.
16.4.2 Older Women (>35 Years Old)
The impact of luteinizing hormone administration in ovarian stimulation with gonadotropin-releasing hormone (GnRH) antagonist cycles was examined by Bosch and colleagues in a randomized controlled trial (RCT) involving 720 women undergoing their first or second IVF [75]. The authors compared cycle outcome, according to the use of rec-hFSH or rec-hFSH + rec-hLH in an age-adjusted analysis. For the patients <36 years old, the total starting dose of gonadotropins was 225 IU/d for both stimulation protocols. In the rec-hFSH-alone group, 225 IU/d SC of rec-hFSH was administered, and the starting dose for the rec-hFSH + rec-hLH group was 150 IU/d of rec-hFSH and 75 IU/day of rec-hLH. As noted, LH supplementation was started on stimulation day 1. For those patients aged 36–39 years, the total starting dose of gonadotropins was 300 IU/d for both study groups: rec-hFSH-alone, 300 IU/d SC of rec-hFSH was administered, and for the rec-hFSH + rec-hLH group, 225 IU/d of rec-hFSH + 75 IU/d rec-hLH. The rec-hLH dose remained fixed across the cycle. In the younger population (up to 35 years old), implantation rates were similar, 27.8 % versus 28.6 %, odds ratio (OR) 1.03 (95 % confidence interval [CI] 0.73–1.47), as was the ongoing pregnancy rate per started cycle, 37.4 % versus 37.4 %, OR 1.0 (95 % CI 0.66–1.52). In older patients (36–39 yrs.), the implantation rate was significantly higher in the rec-hFSH + rec-LH group: 26.7 % versus 18.6 %, OR 1.56 (95 % CI 1.04–2.33). Ongoing pregnancy rates per started cycle were not statistically different: 33.5 % versus 25.3 %, OR 1.49 (95 % CI 0.93–2.38).
Contrary results have been reported by Konig et al. in an RCT involving 253 couples undergoing IVF/ICSI [76]. In their study, women were 35 years or older and received ovarian stimulation in a GnRH antagonist protocol with either rec-hFSH 225 IU/day or rec-hFSH + rec-hLH 150 IU/d starting on stimulation day 6. The intention-to-treat analysis revealed implantation rates (18.8 % vs. 20.7 %; mean difference −1.9 %, 95 % confidence interval [CI] −8.0 to 11.7) and clinical pregnancy rates (28.0 % vs. 29.7 %; mean difference −1.5 %, 95 % CI −9.4 to 12.7).
A systematic review and meta-analysis of the studies examining the age-related effects of LH supplementation in COS were conducted by Hill and colleagues [57]. The authors demonstrated that LH supplementation in women aged >34 years old undergoing COS with rec-hFSH was beneficial. Their study included 7 RCTs (902 women) and compared COS using rec-hFSH alone or in combination with rec-hLH. GnRH-agonist downregulation was used in five trials, while GnRH antagonist and GnRH-agonist micro-flare were used in the remaining trials. The dose and day of starting rec-hLH supplementation varied among trials. In five of them a fixed dose of 150 IU rec-hLH, which started either on the sixth or seventh stimulation day, was used. One trial used a fixed 2:1 ratio of rec-hFSH and rec-hLH, while another used a fixed dose of 75 IU rec-hLH regardless of the FSH dose; in both of them LH supplementation was given from the first day of stimulation on. Implantation (OR = 1.36; 95 % CI: 1.05–1.78, I 2 = 12 %) and clinical pregnancy rates (OR = 1.37; 95 % CI: 1.03–1.83, I 2 = 28 %) were significantly higher for women who received rec-hLH in addition to rec-hFSH compared with those in whom rec-hFSH was administered alone.
The meta-analysis by Hill et al. was subsequently reexamined by Konig and colleagues, who replaced the study of Bosch and cols. by their own and concluded that no effect whatsoever could be observed by adding LH to older patients [77]. Nevertheless, a methodological bias could have been produced by replacing the Bosch and colleagues’ study, which represented 36 % of the weight of all studies pooled in the aforementioned meta-analysis, by the one of Konig and cols. because the protocols of COS differed with regard to the day LH supplementation has started; LH supplementation was started in the mid-follicular phase in the latter in contrast to the former, in which rec-LH was administered since the first day of stimulation. It has been suggested that LH supplementation should be initiated on the beginning day of stimulation to get total advantage of the LH effects on both theca and granulosa cells [78].
In conclusion, evidence suggests that rec-hLH supplementation has a positive effect on cycle outcome of older women (>35 years old), particularly when used from the start of COS. Nevertheless, given the heterogeneity of the published data, additional large RCTs examining the impact of rec-hLH supplementation from the early phases of COS are needed to draw a conclusive recommendation about the routine incorporation of rec-hLH in older women undergoing IVF/ICSI.
16.4.3 Poor Responders
Mochtar et al., evaluating poor responders, have demonstrated the usefulness of adding rec-hLH to COS. These authors pooled three RCTs including 310 participants and showed that higher ongoing pregnancy rates (OR = 1.85; 95 % CI: 1.1–3.11) were obtained in patients treated with the combination of rec-hFSH and rec-hLH compared with rec-hFSH alone [54]. In another meta-analysis of Bosdou et al., which included 7 RCTs and 603 patients classified as poor responders, differences in clinical pregnancy were not detected in the group of patients receiving LH supplementation [58]. Nevertheless, the definition criteria for poor responders were not uniform among the included studies, and two of the RCTs evaluated slow/hyporesponders rather than poor responders. The protocols of stimulation also varied as GnRH antagonists and agonists were applied in two trials each, and GnRH-agonist short protocol was used in three subjects. The way rec-hLH supplementation was given also varied as daily doses of either 75 IU or 150 IU were used, and the starting day differed or was not traced. Rec-hLH was added to rec-hFSH from the first stimulation day in one trial, at stimulation day 7 in three trials, at day 8 in one trial, and on the day of the first GnRH antagonist injection in another trial. Although statistical significance was not reached, the magnitude of the effect size and the width of the 95 % CI regarding the clinical pregnancy rates (RD = +6 %; 95 % CI: −0.3 to +13 %; p = 0.06) suggested a potential clinical benefit of LH supplementation. Nevertheless, the authors of the aforesaid meta-analyses did find that rec-hLH supplementation was beneficial in terms of live birth rates after IVF (RD = +19 %; CI: +1 to +36 %), but their results were derived from a single RCT.
Lately, a large meta-analysis assessed the outcomes of rec-hFSH plus rec-hLH or rec-hFSH alone for ovarian stimulation in association with GnRH analogues during ART [55]. A total of 40 RCTs involving 6443 women aged 18–45 years were included, of which 14 studies (1129 patients) specifically investigated poor responders. Poor response (POR) was defined according to study authors’ criteria and although the studies were published prior to the European Society of Human Reproduction and Embryology (ESHRE) consensus definition of POR [79], in 10 of the 14 studies reporting POR data, the definition of POR employed was aligned with the subsequently reported ESHRE definition. According to the ESHRE consensus, POR is defined by the presence of at least two of the following three features: (1) advanced maternal age (≥40 years) or any other risk factor for POR, (2) a previous POR (≤3 oocytes with conventional stimulation), and (3) an abnormal ovarian reserve test (antral follicle count [AFC] <5–7; anti-Mullerian hormone [AMH] <0.5–1.1 ng/mL), but two episodes of POR after maximal COS per se are sufficient to define a patient as poor responder. Patients of advanced age with an abnormal ORT may be classified as POR since these features indicate reduced ovarian reserve and act as a surrogate of ovarian stimulation cycle outcome. In this case, the patients should be defined as “expected poor responder” [79]. In the aforementioned study by Lehert and colleagues, significantly more oocytes were retrieved with rec-hFSH plus rec-hLH versus rec-hFSH alone in poor responders (12 studies, n = 1077; weighted mean difference +0.75 oocytes; 95 % CI 0.14–1.36). Also, significantly higher clinical pregnancy rates were observed in this patient category with rec-hFSH plus rec-hLH versus rec-hFSH alone (14 studies, n = 1179; RR 1.30; 95 % CI 1.01–1.67; ITT population).
In conclusion, current evidence suggests that there is an increase in both the number of oocytes retrieved and clinical pregnancy rates in poor responders treated with rec-hLH in addition to rec-hFSH.
16.4.4 Hyporesponders
The concept of “hypo-response” to COS has been proposed to identify those at first hand good prognosis normogonadotropic young women with normal ovarian reserve who turn out to require high amounts of rec-hFSH (e.g., >2500 IU total dose) to obtain an adequate number (i.e., >4) of oocytes retrieved [64–67]. Such normo-ovulatory and normogonadotropic women differ from classical poor responders because they are usually young (<39 years) and ovarian biomarkers (AMH/AFC) are within normal ranges. Although the pathogenesis of hyporesponsiveness to FSH is unknown, it has been speculated that hypo-response is a genetically determined condition (see Chapter 14: Pharmacogenomics Approach to Controlled Ovarian Stimulation). More specifically, ovarian resistance to exogenous FSH has been associated with the presence of at least two genetic variations, including a polymorphic allele of the LH beta-subunit gene (v-betaLH), which has been shown to have altered in vitro and in vivo activities, and FSH receptor (FSH-R) Ser/680 variant [69, 80–83]. Given a possible association between ovarian resistance to FSH stimulation (hypo-response) and a genetically determined less bioactive LH molecule or FSH-R dysfunction, several investigators have examined the roles of exogenous LH activity supplementation and increased FSH doses on ART cycle outcome.
The role of LH supplementation and increased FSH doses in hyporesponders were evaluated by Ferraretti and colleagues, who conducted an RCT involving 184 patients (age <38 years) undergoing COS for IVF after pituitary desensitization [67]. Hyporesponsiveness to rec-hFSH was defined by the observation of a steady follicular growth (>10 antral follicles ≥8 mm in diameter) and estradiol levels (≥100 pg/mL) between stimulation days 7–10 despite continuous rec-hFSH administration. Upon reaching this stage, patients were randomized to receive (1) an increased rec-hFSH dose alone (max 450 IU/daily; n = 54), (2) LH activity supplementation with rec-hLH (75 IU/day or 150 IU/day) in addition to an increased FSH dose (n = 54), and (3) LH activity supplementation with hMG in addition to an increased rec-hFSH dose (n = 26). Fifty-four age-matched women with normal responses to COS were included as a control group. The average number of oocytes retrieved was significantly lower in hyporesponders treated with rec-hFSH step-up (8.2) versus the other three groups (11.1, 10.9, 9.8), respectively. Pregnancy rates were significantly higher in the group treated with rec-hLH plus increased rec-hFSH dose (54.4 %) compared with both the patients receiving rec-hFSH alone (24.4 %) and hMG (11 %; p < 0.05). Pregnancy rates in the group of women receiving rec-hLH supplementation and an increased rec-hFSH dose were not different from controls (41 %). Although live birth rates in both rec-hLH (40.7 %) and control (37 %) groups were twofold higher than the other two groups (22 % and 18 %, respectively), the difference did not reach statistical significance.
The role of rec-hLH supplementation per se in hyporesponders was evaluated by De Placido and colleagues, who conducted a multicenter RCT involving a total of 117 IVF/ICSI cycles [66]. Hyporesponders (age <37 years, basal FSH ≤10 IU/l) were defined by the presence of low serum estradiol levels (below 180 pg/mL) and at least 6 follicles ranging between 6 and 10 mm, but no follicles over 10 mm on stimulation day 8 with rec-hFSH. After pituitary desensitization and stimulation with a fixed dose (225 IU/day) of rec-hFSH for the first 8 days, the patients were randomized to receive either an additional 150 IU/day of rec-hLH supplementation (n = 65) or an increase in the daily dose of rec-hFSH by 150 IU/day (n = 65; rec-hFSH “step-up” protocol). An age/BMI-matched population of “normal responders” (i.e., tripling E2 levels between stimulation days 5 and 8 and more than 4 follicles >10 mm on stimulation day 8) was selected as a control group (n = 130). The number of oocytes retrieved was significantly higher in the patients who received rec-LH supplementation (9.0 ± 4.3) compared with those in whom an increased dose of rec-hFSH was administered (6.1 ± 2.6; p < 0.01), and the results of both aforementioned groups were lower than those obtained in the control group (10.49 ± 3.7; p < 0.05). Implantation and ongoing pregnancy rates were similar in “hyporesponders” treated with rec-hLH and “normal responders” (14.2 % and 32.5 % vs. 18.1 % and 40.2 %, respectively). Conversely, both parameters were significantly lower (p < 0.05) in “hyporesponders” treated with step-up rec-hFSH (10.0 and 22.0 %).
In conclusion, these studies reinforced the idea that hyporesponders benefit from LH supplementation and that hyporesponsiveness to rec-hFSH could be related to the presence of less bioactive LH due to specific genetic-determined variations in the beta subunit of the LH molecule.
16.4.5 Deeply Suppressed LH Levels
Profound suppression of LH concentrations as a consequence of GnRH-agonist downregulation has been found in 7–48 % of normogonadotropic women undergoing controlled ovarian stimulation [60, 61, 84, 85]. This wide variation might be justified by the type and mode of GnRH-agonist action. Intranasal administration of buserelin resulted in significantly less depressed levels of mid-follicular LH levels compared with the subcutaneous route. Moreover, the inhibitory effect on ovarian steroidogenesis and follicular development is more evident with the more potent buserelin than with leuprolide acetate [61, 85].
Early studies have suggested that ovarian response and IVF cycle outcome are negatively impacted when mid-follicular serum LH levels are below a certain threshold (between 0.5 and 0.7 UI/L) after downregulation with GnRH agonists and ovarian stimulation with FSH monotherapy [59–63, 84]. Westergaard and colleagues, retrospectively analyzing 200 normogonadotropic women, reported that as many as 49 % of those stimulated with rec-hFSH under pituitary suppression with buserelin acetate (0.5 mg SC daily) for 14 days achieved very low concentrations of LH (<0.5 IU/L) in the mid-follicular phase, albeit GnRH-a dose was reduced to 0.2 mg SC per day during ovarian stimulation [60]. In comparison with the normal LH group, these women had serum estradiol concentrations significantly lower on Sd8 (1349 ± 101 vs. 2908 ± 225 pmol/L; p < 0.001). Although the proportion of patients with a positive pregnancy test was similar in the two groups (30 % vs. 34 % per started cycle in the low and normal LH groups, respectively), a fivefold higher risk of early pregnancy loss was observed in the low LH group (45 % vs. 9 %; p < 0.005). In another study, Fleming and colleagues found that 26 % of women treated with highly purified or recombinant FSH and GnRH agonist (type and dose not reported) had suppressed LH concentration (≤0.7 IU/l) on Sd7 [84]. Patients with suppressed LH had lower estradiol concentrations (p = 0.001) irrespective of whether the FSH is derived from purified urinary or recombinant sources. However, the negative impact on cycle outcome (longer treatment duration combined with a reduced oocyte yield) was observed only in women treated with urinary FSH, thus indicating that the more potent recombinant FSH treatment could overcome the impact of LH suppression upon gross ovarian response. Furthermore, no effect upon pregnancy outcome was observed irrespective of the level of LH suppression and type of FSH preparation. Humaidan and colleagues also studied the effect of LH levels on stimulation day 8 on ovarian response and pregnancy outcome [61]. The authors retrospectively analyzed 207 normogonadotropic women receiving pituitary downregulation with buserelin acetate (0.8 mg SC daily until pituitary downregulation and 0.4 mg/day during ovarian stimulation) and ovarian stimulation with rec-hFSH. LH levels on Sd8 were directly related to estradiol levels and inversely related to the total consumption of exogenous FSH and duration of gonadotropin stimulation (p < 0.002). In their study, however, only 12 % of the patients showed LH levels <0.5 IU/L. While the number of retrieved oocytes was not affected by LH suppression, the frequency of fertilized oocytes was significantly lower in the group with profound LH suppression (p < 0.05). Likewise in the study of Fleming and cols., pregnancy and implantation rates were not significantly affected by profound mid-follicular LH suppression. Taken together, these aforementioned studies indicate that deeply suppressed LH levels in GnRH agonist-treated women have a significant impact on the ovarian response during ovarian stimulation, but its impact on pregnancy outcome is controversial.
Contrary results have been reported by Balasch and colleagues studying 144 infertile women undergoing IVF/intracytoplasmic sperm injection (ICSI) treatment, in whom pituitary desensitization was carried out by the administration of leuprolide acetate (1 mg SC daily, then reduced to 0.5 mg after downregulation was confirmed) [85]. Using a receiver-operating characteristic (ROC) analysis, the authors showed that the serum LH concentration on Sd7 was unable to discriminate between conception and non-conception cycles (AUC = 0.52; 95 % CI: 0.44–0.61). In this study, only 7 % of the patients had mid-follicular LH serum concentration <0.5 IL/L, and no significant differences were found with respect to ovarian response, number of oocytes retrieved, IVF/ICSI outcome, implantation, and the outcome of pregnancy between these patients and those with normal LH on Sd7.
In conclusion, conflicting evidence exists regarding the impact of deeply suppressed levels of mid-follicular serum LH levels on ovarian response to stimulation with rec-FSH. Current data indicate that the choice of GnRH-a plays a role in the frequency of patients exhibiting profound mid-follicular LH suppression. Whether these women would benefit from supplementation with LH activity during ovarian stimulation remains to be proven.
16.5 Gonadotropin Preparations Containing LH Activity
Currently, there are three commercially available gonadotropin preparations containing LH activity: (1) urinary hMG, in which LH activity is dependent on hCG rather than LH, (2) pure LH glycoprotein produced by recombinant technology (lutropin alfa), and (3) a combination of FSH (follitropin alfa) and LH (lutropin alfa) in a fixed ratio of 2:1 also manufactured by recombinant technology (Sect. 16.1) [3].
16.5.1 Menotropin
Menotropin, or human menopausal gonadotropin (hMG), was first extracted from the urine of postmenopausal women in 1949 [2]. Early preparations contained varying amounts of FSH, LH, and hCG in only 5 % pure forms [1–3]. Improvements in the purification techniques standardized FSH and LH activities to 75 IU for each type of gonadotropin in 1963, as measured by standard in vivo bioassays (Steelman–Pohley assay). Human menopausal gonadotropin preparations have both FSH and LH activity, but the latter is primarily derived from the hCG component present in postmenopausal urine and concentrated during purification [2, 86, 87, 88]. Sometimes hCG is added to achieve the desired amount of LH-like biological activity [2]. In 1999, purified hMG gonadotropins were introduced, allowing its subcutaneous (SC) administration [2, 3]. At present, both conventional hMG and highly purified hMG (HP-hMG) are commercially available in vials containing lyophilized powder of FSH and LH at 1:1 ratio [3]. The enhanced purity of HP-hMG enabled subcutaneous delivery [3].
16.5.2 Lutroprin Alfa
Lutroprin alfa was introduced in the market in the year 2000 intended for promoting ovarian stimulation in women with WHO type I anovulation. The manufacturing process of lutropin alfa involves recombinant technology in which the genes coding for human LH alpha and beta subunits are incorporated into the nuclear DNA of Chinese hamster ovary (CHO) cells via a plasmid vector [2, 3, 88]. As a result, a master LH-producing cell bank is built [88, 89]. A working cell bank is then made by growing cells in culture flasks, which are afterwards combined with a suspension of microcarrier beads and transferred to a bioreactor vessel with continuous culture media infusion. The cell culture supernatant medium, containing the proteins secreted by the cells, is collected from the bioreactor. The harvested “crude LH” is then purified by chromatography, followed by ultrafiltration. Each purification step is rigorously controlled in order to ensure batch-to-batch consistency of the final purified product that is the recombinant human LH (rec-hLH) [3].
Lutropin alfa is highly pure and has high biological activity (9000 IU/mg protein) [3, 90]. It is presented in vials of 82.5 IU lyophilized pure glycoprotein powder to be reconstituted with diluent before administration using a conventional syringe and needle (75 IU of lutropin alfa is delivered per vial). Lutropin alfa is intended for subcutaneous daily injections, which represents an important gain for patients as better tolerability (lower pain at injection site) has been reported with SC injections compared with the intramuscular route. Importantly, SC injections allow self-administration that is more convenient and less time consuming as patients need fewer visits to the clinic or hospital for injections [91, 92]. Due to the relatively short half-life of LH, daily injections of lutropin alfa are needed during the stimulation period [3, 90]. After each injection, terminal half-life is reached within 10–12 h, and then LH levels decline until the next injection.
16.5.3 Follitropin Alfa in Combination with Lutropin Alfa
A preparation containing both rec-hFSH (follitropin alfa) and rec-hLH (lutropin alfa) at 2:1 ratio was launched in 2007 [93]. The 2:1 ratio of FSH and LH in a fixed dose combination was obtained by recombinant technology and vial filling using protein mass (FbM). The use of FbM as opposed of filled-by-bioassay was possible due to the specific activity; isoform distribution and sialylation profile of both gonadotropins are highly consistent among manufactured batches [88]. It is intended for subcutaneous daily injections and is presented in vials of lyophilized pure glycoprotein powder to be reconstituted with diluent before administration using a conventional syringe and needle (150 IU of follitropin alfa and 75 IU of lutropin alfa is delivered per vial). The results of two phase I, randomized, crossover studies demonstrated bioequivalence between rec-hFSH and rec-hLH administered alone or in fixed 2:1 combination, thus allowing administration of both recombinant gonadotropins in a single injection [94].
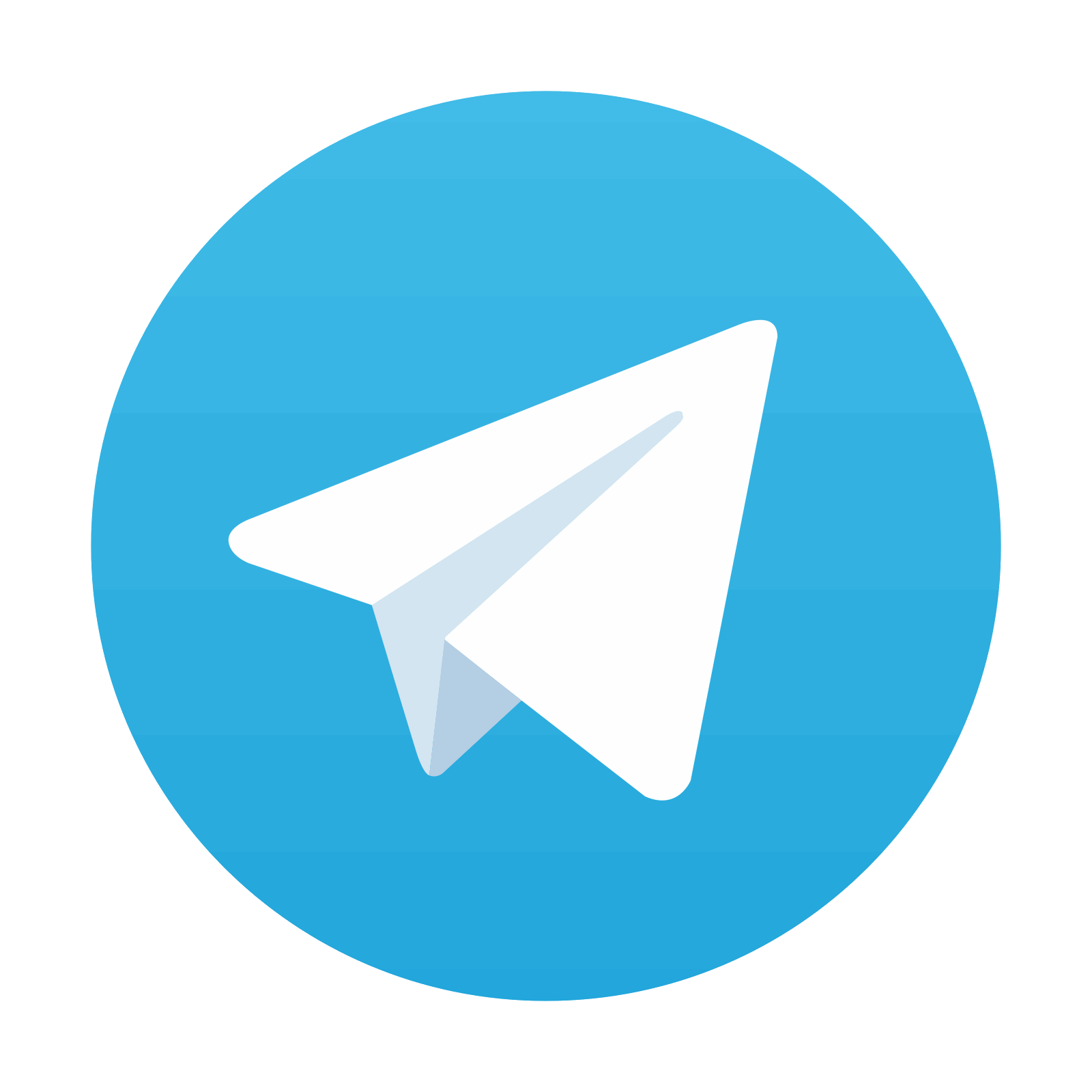
Stay updated, free articles. Join our Telegram channel

Full access? Get Clinical Tree
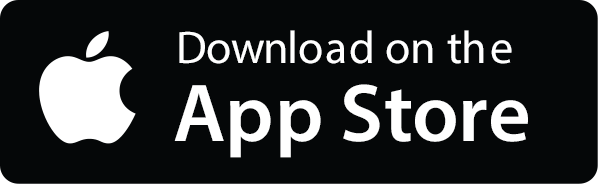
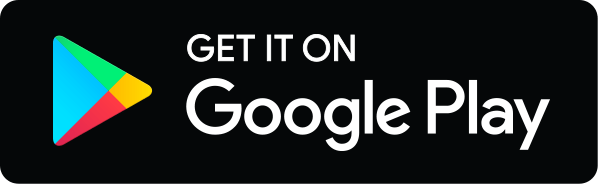