© Springer International Publishing AG 2018
Ahmad Majzoub and Ashok Agarwal (eds.)The Complete Guide to Male Fertility Preservationhttps://doi.org/10.1007/978-3-319-42396-8_55. The Effect of Cancer and Its Treatment on Natural-Born Offspring
(1)
Scott Department of Urology, Baylor College of Medicine, Houston, TX, USA
(2)
Scott Department of Urology, Baylor College of Medicine, 6624 Fannin Street, Suite 1700, Houston, TX 77030, USA
Keywords
Natural conceptionCongenital abnormalitiesFertility preservationMen with cancerSpermatogenesisChemotherapyRadiationAbnormal semen parametersAssisted reproductive technologiesOligospermiaIntroduction
Cancer continues to be a leading cause of morbidity and mortality in our society and does not discriminate based on age. Many patients are of reproductive age or younger. Approximately 9.2% of patients diagnosed with cancer in the United States are less than 45 years old, and 1.1% are under 20 years old [1]. As advancements in medicine lead to improvements in cancer diagnosis and treatment , more of these younger patients survive. The 5-year survival rate is now greater than 80% among all age groups [2]. In this context of increasing survival among oncology patients with reproductive potential, it is becoming increasingly important to consider future fertility in patients undergoing cancer treatment.
Although new modalities are emerging, surgery, chemotherapy, and radiation remain the foundation of cancer treatment. Unfortunately, all of these modalities can negatively affect fertility potential. The American Society of Clinical Oncology released formal recommendations for fertility preservation in 2006 and an updated version in 2013 [3]. The recommendations include discussing fertility preservation with all patients of reproductive age or their guardians if the patients are minors and referring patients who express an interest in fertility preservation to reproductive specialists.
Despite these recommendations, many patients with not only reproductive potential but also interest in future fertility are not referred for fertility preservation . Schover et al. reported that of men and women who were childless before cancer diagnosis, 76% desired children in the future [4]. Additionally, only 57% received information from their physicians about fertility after cancer treatment, and only 24% of childless men banked sperm before cancer treatment. Kohler et al. found that, although 86% of responding pediatric oncologists agreed with the recommendations to refer all pubertal males to a physician who specializes in fertility preservation prior to oncologic treatment, only 46% of respondents reported actually doing so more than half of the time [5]. It is also important not to make assumptions based on age about desired fertility. Salonia et al. found that up to 20% of men preparing to undergo a radical prostatectomy with an average age of 62.2 years reported desire to cryopreserve sperm before the procedure [6]. Therefore, it is imperative that the physician include a discussion of fertility preservation when counseling patients before receiving treatment for cancer.
This chapter reviews the effects of cancer and its treatment on male fertility in regard to natural conception specifically, as the use of assisted reproductive technologies and sperm cryopreservation before cancer therapy will be covered elsewhere. The literature describing the impact of cancer and its treatment on spermatogenesis, likelihood of natural conception, and discussion of potential congenital abnormalities as a result of DNA damage in sperm is discussed.
Spermatogenesis in Cancer Patients
Spermatogenesis occurs in the seminiferous tubules which account for approximately 70–80% of the testicular volume. There are an estimated 83,000,000 diploid germ cells present in the seminiferous tubules prior to pubertal development [7, 8]. Spermatogonial cells are comprised of three subtypes with specific roles: type A (dark), type A (pale), and type B. Type A (dark) spermatogonia function as quiescent, diploid reserve cells, which are active only during initial pubertal development and following stem cell depletion from exposure to gonadotoxins. Type A (pale) spermatogonia are more mitotically active and serve as permanent progenitor cells, with self-renewal occurring at each mitotic division. Type B spermatogonia also undergo frequent division as the immediate precursors to primary spermatocytes that then undergo two meiotic divisions before ultimately producing spermatids. Type A (dark) spermatogonia are therefore considered the most resistant to injury from chemotherapy or radiation, as opposed to type A (pale) and type B spermatogonia which have higher mitotic activity. These cellular qualities likely explain the transient arrest in spermatogenesis and preservation of sperm production observed following small, finite doses of chemotherapy and radiation [9–19].
Abnormal Semen Parameters in Cancer Patients
It is important to discuss abnormal semen parameters seen in men with cancer prior to receiving treatment. It is not unusual to see abnormal semen parameters in men without cancer; however, men with cancer prior to treatment are more frequently observed to have abnormal semen parameters at baseline as a result of the disease. One study reviewed all patients presenting for sperm cryopreservation in an American sperm bank and found that over 35% of all men having sperm cryopreserved for any reason had low sperm counts, while over 50% of men presenting with testicular cancer had oligospermia [20]. Hendry et al. found that 50–70% of testicular cancer patients were subfertile or had impaired spermatogenesis before the start of chemotherapy [21]. These authors also described that impaired spermatogenesis was neither related to stage of disease nor to the duration or severity of symptoms attributed to testicular cancer. Although impaired semen parameters in pretreatment cancer patients have been most extensively study in the testicular cancer population, a clear association also exists with Hodgkin’s lymphoma. Williams et al. retrospectively evaluated 717 semen samples from 409 men with 8 different types of cancer including testicular cancer and lymphoma. The investigators found that men with most types of cancer had pretreatment semen parameters that were within the fertile range in regard to density and in the intermediate range for motility, but men with testicular cancer had statistically lower semen quality compared to those with other malignancies [20].
The etiology for abnormal semen parameters in cancer patients prior to treatment is likely multifactorial. Several putative mechanisms have been proposed. These include hormones and cytokines secreted by tumors that may disrupt spermatogenesis, disturbances between the balance of subpopulations of T lymphocytes in lymphomas, hyperthermia, and central endocrine disruption—all of which may play a role in this pathologic process [22, 23]. Barr et al. described local effects of the disease on the testis itself that are likely immune mediated, resulting in significant fibrosis in testicular cancer and hyalinization of the seminiferous tubules in lymphoma [24]. Stahl and colleagues conducted a study to evaluate the DNA integrity in cryopreserved sperm from 121 cancer patients before treatment [25]. Testicular cancer and Hodgkin’s lymphoma patients had a higher degree of DNA fragmentation than controls prior to treatment. The same trend was observed for other cancer diagnoses, but this trend did not reach statistical significance.
Abnormal sperm DNA condensation is adversely correlated with fertility potential. Spermon et al. evaluated DNA condensation and DNA breaks using chromomycin A3 (CMA3) and the TdT-mediated dUTP nick-end labelling assay (TUNEL) in patients who received hemi-orchiectomy for testicular cancer, but before receiving chemotherapy [26]. In all patients with testicular cancer after hemi-orchiectomy, 22% were found to have teratospermia before the start of chemotherapy, and 47% had teratospermia after chemotherapy. In contrast, the iatrogenic causes of germ cell loss through chemotherapy and radiation affecting mitosis and meiosis are well understood.
Negative Impact of Treatments on Sperm Production and Normal Ejaculation
Surgery, chemotherapy, and radiation are the mainstays of cancer treatment, and all affect spermatogenesis leading to abnormal semen parameters after treatment. Typically, the depression of spermatogenesis is transient, but occasionally it can lead to permanent sterility.
Chemotherapy
Chemotherapy exerts its effect on spermatogenesis by direct damage to spermatogonia. Depletion of spermatozoa is progressive over time after starting chemotherapy; however, sperm counts may rebound after cessation of therapy. Severe oligospermia and azoospermia frequently present in the first 6 months after the start of chemotherapy, and recovery depends on the extent of stem cell loss. Stem cell regeneration may be delayed by several years. Even in men with prolonged azoospermia, spermatozoa can be found in 30–50% of testicular sperm extractions 5 years after chemotherapy [27, 28]. The detrimental effect on spermatogenesis can vary significantly depending on what type of drug, combination of drugs, and cumulative dose are administered. Nitrogen mustard derivatives (e.g., mechlorethamine), alkylating drugs (e.g., cyclophosphamide, ifosfamide, procarbazine, and busulfan), and platinum-based drugs (e.g., cisplatin) have been shown to have the most adverse effects on sperm production [27, 29]. Cyclophosphamide has been found to have a dose-dependent effect on spermatogenesis with doses greater than 10 g/m2 associated with an increased risk of sterility [27, 30].
Alkylating agents tend to be used in combination with other chemotherapeutic drugs which significantly increases their toxicity to spermatogenesis [31]. This negative effect is exemplified in patients treated with different regimens for Hodgkin’s lymphoma. The older MOPP treatment (mechlorethamine, vincristine, prednisone, and procarbazine) was shown to cause azoospermia in 100% (47 of 47 cases) of patients 1 year after treatment [29, 32]. In contrast, one study showed that 90% of men had no change in their sperm count 1 year after treatment with the newer ABVD regimen (doxorubicin, bleomycin, vinblastine, and dacarbazine) [29, 33]. With regard to testicular cancer, in one series after orchiectomy and cisplatin-based chemotherapy, Lampe et al. reported normospermia in 64% of patients after 1 year and in 80% of patients 3–5 years after treatment. Additionally, the combination of bleomycin, etoposide, and cisplatin has demonstrated an intermediate risk of prolonged sterility affecting 20% of patients following testicular cancer treatment [27, 34, 35].
Chemotherapy when added to radiation has been shown to substantially increase the risk of permanent sterility. Anserini et al. found that cyclophosphamide with total body or thoracoabdominal radiation resulted in azoospermia for at least 4 years with only 17% of patients recovering sperm in the ejaculate thereafter [27, 36].
Radiation
Two factors that have been shown to influence the level of spermatogonia toxicity in radiation are cumulative dose and testicular exposure to radiation. Unlike conventional radiation to other organ systems where fractionated doses have been shown to be favorable for overall organ toxicity, fractionated courses of radiation to the testis have been shown to increase toxicity when compared to a single dose. Howell et al. and Trottmann et al. reported that doses of 0.15 Gy can lead to sperm count reduction while doses of 0.35 Gy can cause reversible azoospermia [37, 38]. Additionally, doses up to 2.5 Gy can result in prolonged azoospermia with an increased chance of permanent azoospermia while doses greater than 20 Gy are generally considered to be lethal to spermatogonia [29, 37, 38]. The literature suggests that external beam radiation can lead to greater impairment of spermatogenesis when compared to localized brachytherapy or indirect scatter, e.g., from treatment of rectal cancer [29]. In addition to germinal epithelial damage, endocrine cells are affected as well. Howell et al. described a transient dose-dependent increase in FSH with concomitant decrease in sperm production with doses between 0.2 and 0.7 Gy that returned to normal values within 12–24 months [37]. Leydig cells have a higher threshold for damage, and testosterone production is not observed to be significantly impaired until doses reach greater than 14Gy [39].
Surgery
Surgery can affect sperm production and the delivery of sperm due to alterations in anatomy. Pelvic surgery can not only damage nerves but can also leave the genital tract in discontinuity, such as damage or removal of the vas deferens, seminal vesicles, or prostate [29]. Retroperitoneal lymph node dissection can damage sympathetic nerves that control normal ejaculatory processes, which can result in anejaculation or retrograde ejaculation [29]. Tumor invasion into a unilateral testicle or unilateral orchiectomy can impair sperm production by reducing seminiferous tubule mass, whereas bilateral orchiectomy obviously eliminates sperm production entirely [29].
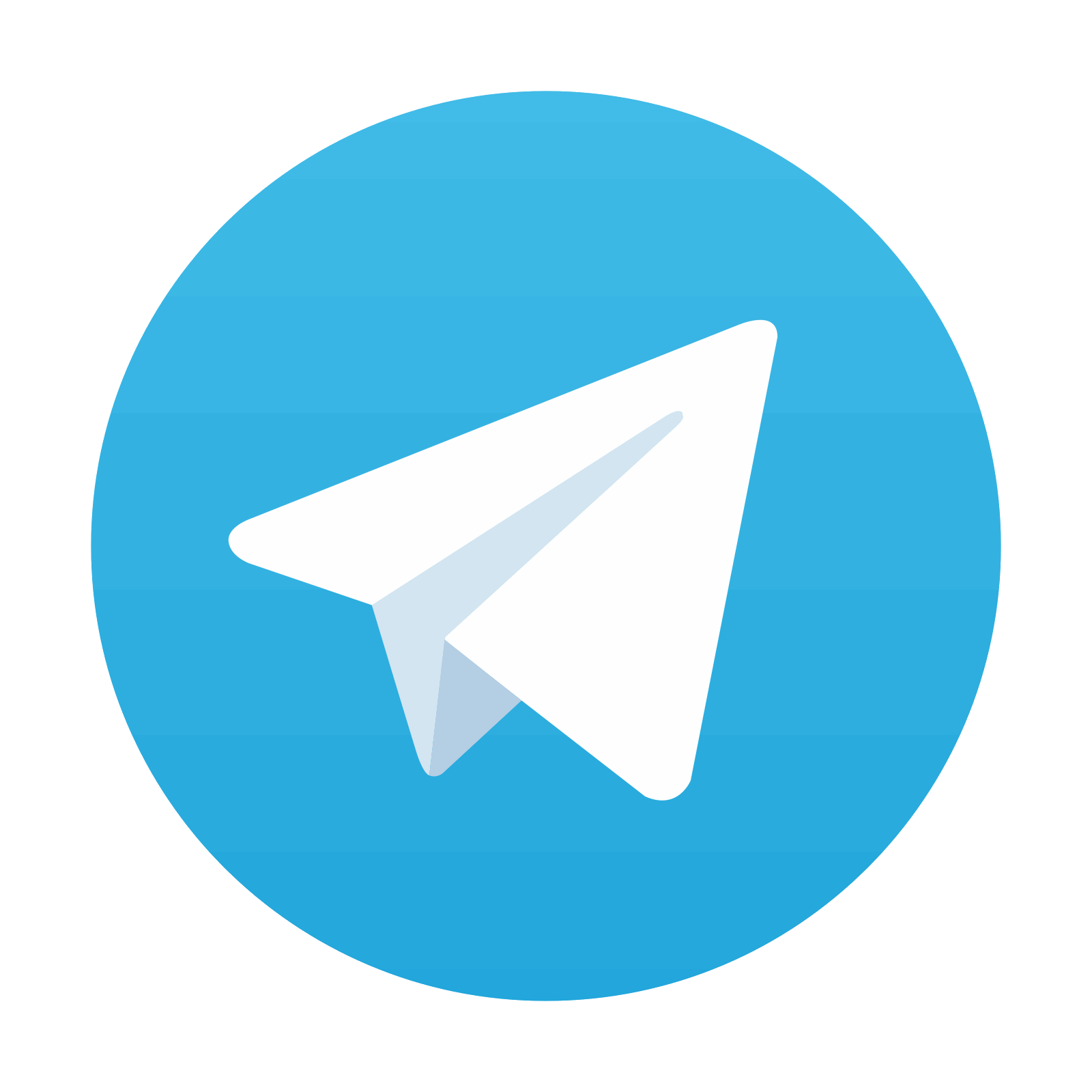
Stay updated, free articles. Join our Telegram channel

Full access? Get Clinical Tree
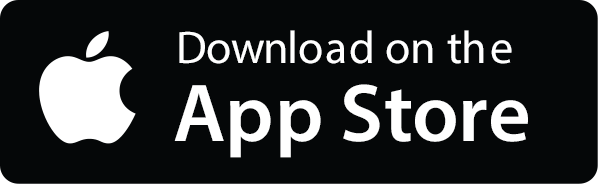
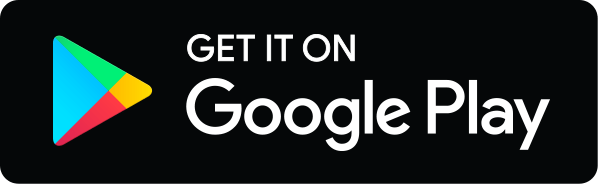