html xmlns=”http://www.w3.org/1999/xhtml” xmlns:mml=”http://www.w3.org/1998/Math/MathML” xmlns:epub=”http://www.idpf.org/2007/ops”>
The androgen receptor is central to the overall understanding of the effects of androgens throughout the body. In this chapter, we will review some of the key elements around the structure of the androgen receptor, the relationship between these structural parameters, and the function of the androgen receptor system. This knowledge enables the clinician to better understand and modulate the impact of androgens in health and in disease states.
Historical context
Understanding the role of androgens in gynecology was for many years driven by a simple approach to androgens and the androgen receptor complex. There were two primary constructs relative to androgens and their action:
One construct highlights the broad range of activity of testosterone and its androgenic precursors. It states that these compounds act both directly as androgens, and as estrogens. The latter effect occurs via the conversion of testosterone to estradiol by the aromatase enzymatic system. This construct has fueled much scientific exploration, as well as heated debates, around which effects of testosterone truly reflect androgen-mediated effects, as opposed to estrogenic effects achieved through aromatization. In this model, much of the so-called androgenic effects are purely an extension of those ascribed to estrogens.
The other construct emphasizes the activity of non-aromatizable androgens. These include dihydrotestosterone, as an endogenously produced androgen, and methyltestosterone, as a pharmacologic agent. The historical perspective is that these compounds can only act via the androgen receptor and, therefore, display uniquely androgenic effects.
In this chapter, we will review the current and evolving science of the androgen receptor, its complex dynamics, and how the traditional constructs must be revised in light of the evolving evidence.
High-level overview of the androgen receptor structure
Advances in molecular biology over the past decades have established the androgen receptor as a cornerstone of health and many disease states [1,2]. The androgen receptor is located on the X chromosome [3,4]. Most mutations detected so far in the androgen receptor are associated with disease.
The androgen receptor is similar in structure and function to other steroid hormone receptors, such as the estrogen and the progesterone receptors [5]. The last 20 years have contributed much to the overall understanding of the physiology of these receptors. The classic model for years was one of “lock and key,” where a given hormone would bind to its receptor and trigger a specific activity. Competitive inhibitors, so-called antagonists, would have the ability to bind the receptor and block any further activity. It is now obvious that the reality is far more complex and dynamic [5,6].
All of the steroid receptors appear to function in a similar manner [6]. For purposes of the current description, we will refer to the androgen receptor, though much of the understanding has been derived initially from the study of the estrogen and glucocorticoid receptors [7,8].
The free form of the androgen receptor is predominantly found in the cytosol, where it is essentially inactive [9]. This neutral state is the result of a highly dynamic set of interactions with an array of intracellular factors, referred to as chaperones [9].
The cytoplasm and nucleus are also rich in regulatory factors [10]. Some of these can amplify the activity of the hormone-bound receptor; they are known as co-activators [11]. Other regulatory factors cause downregulation; they are known as co-repressors [11]. These regulatory factors bind to the receptor, even in the absence of the primary androgen substrate. An important concept is that the amount and type of regulatory factors varies by cell type and the prevailing hormone environment within the cell [11].
The general mechanism of hormone action is now well described, though much remains to be further elucidated [5,6,12]. When the androgen reaches the cytoplasm, through diffusion, it binds to the receptor. Generally, two hormone-bound receptors pair up, thus forming a dimer. The hormone-bound dimer reaches the nucleus, where it binds to the target gene and triggers the hormone-linked effect. The binding of the receptor dimer on the DNA occurs in a region referred to as the hormone response element (HRE). While the accompanying regulatory factors are likely to bind the androgen receptor at any time, the most critical time for the modulatory effect is estimated to be at the time of binding of the receptor with DNA.
It is important to understand the broad range of effects that can be seen throughout the body. For the same androgen agonist, the effect varies depending on the target cell, and the magnitude of the effect is modulated based on the balance of co-activators and co-repressors [11]. Some substrates consistently bind to the androgen receptor and block any further activity, so-called pure antagonists [5]. Many more substrates have the ability to act both as agonists and antagonists, depending on the target cell, and on the prevailing balance of co-activators and co-repressors within that cell at that particular point in time [5,6].
One final key concept is that there is a lot of interaction between the various steroid receptors [13]. It has long been recognized that androgens have the ability to bind the estrogen and progesterone receptors [12]. Conversely, estrogens and progestins can bind the androgen receptor [12]. However, recent data have revealed that there are direct interactions between the steroid receptors, which creates even more opportunities for a wide range of activity from any given steroid, including all androgens [13].
Ultimately, the overall system anchored by the androgen receptor is a highly dynamic and complex one. It is very far from the simple and early models that fueled our clinical approach for much of the twentieth century. What is even more important to acknowledge is that we are likely to be still in the early stages of our understanding of the androgen and steroid receptor systems.
Detailed structure of the androgen receptor
The androgen receptor belongs to what is described as a superfamily of nuclear receptors [6]. All of these act as transcription factors. The tally, at the writing of this chapter, enumerates 48 of these in humans, including the receptors for the respective families of steroid hormones, for thyroid hormone, and for vitamin D.
For research purposes, the receptors have been grouped into subfamilies. One grouping (subfamily 3) includes the androgen, progesterone, glucocorticoid, and mineralocorticoid receptors [6].
The androgen receptor shares a common overall structure with other steroid receptors [5,6]. There are many ways of describing the structure of these receptors. A big picture approach describes three components: a region that allows for the binding of regulatory factors (Region A/B), a region that binds to DNA (Region C), and a region that binds the hormone ligand (Region E).
On a more detailed, “anatomical” level, this group of receptors consists of five distinct areas that comprise a total of six regions, as follows [5,6]:
1. The first area encompasses Regions A and B, generally written as Region A/B. This area shows the most variation from one type of steroid receptor to the other. This is the region where the co-regulatory factors described earlier bind to the receptor complex. Functionally, it is referred to as the hormone-independent transactivation function (activation function 1, AF-1) area.
2. The second area is known as Region C. This is the area of greatest similarity between steroid hormone receptors. This area binds directly to DNA, referred to as the DNA-binding domain. It also specifically recognizes target gene promoters (zinc fingers).
3. Next is Region D. It is referred to as the “hinge domain” and is responsible for modulating binding to DNA.
4. The fourth area is Region E. It is the area where the androgen binds to the receptor, or ligand-binding domain (LBD). Functionally, this area acts as a molecular “switch” that stops the receptor from being active in the absence of ligand (or when treated with an antagonist) and that converts it into an active transcriptional factor in the presence of the hormone. This area is also the target for the binding of specific regulatory factors, dependent on the ligand binding to Region E. This is referred to as the transactivation factor (AF-2) area.
5. The fifth and final area is referred to as Region F. It is not present in all steroid receptors and the role of this region has yet to be elucidated. The androgen receptor does not possess this area.
Most steroid receptors have two forms: estrogen receptor: α and β, progesterone receptor: A and B, and for the androgen receptor: isoforms A (AR-A) and B (AR-B) [12,14]. The B form is the full-length androgen receptor, with a full length of 110 kDa [14].
Function of the androgen receptor
Androgen receptor in its inactive state
A number of receptors from the superfamily have the ability to bind to DNA and cause gene activation in the absence of their primary ligand [6]. This finding has led to the understanding that the neutral state for steroid receptors is very much an active process, linked to proteins found within the cytoplasm that bind the receptor and keep it inactive, so-called chaperone proteins, as already stated [9]. An updated understanding has expanded the number and type of these factors, which are now referred to as the foldosome [15]. Data are evolving that aberrations in the dynamics associated with the foldosome can either trigger or increase the susceptibility to specific disease states [15]. This will be discussed later.
The androgen receptor, in its inactive form, is predominantly found in the cytoplasm and bound by the foldosome, including chaperone proteins. Another set of factors is labeled co-chaperone proteins. Additionally, a number of heat shock proteins further contribute to the stabilization and neutralization process, such as HSP90 and HSP70. Other proteins are also involved in the process, such as p23.
This process of inactivation appears to serve at least two purposes. One is to keep the unbound receptor physiologically inactive. The other is to keep the receptor at the ready to bind its ligands, when they reach the cytoplasm.
The foldosome is best thought of as a dynamic functional unit within the cell. It now appears that the respective components are gathered in a sequential manner. Certain heat shock and chaperone proteins appear to initiate the process and recruit other components to produce the stable androgen receptor [16,17]. Early participants in the foldosome include HSP90, HSP70, HIP, HOP, the co-chaperone p23, the immunophilins cyclosporin A-binding protein (Cyp40), and FK506-binding proteins 51 and 52 (FKBP51 and FKBP52) [15]. The current understanding is that foldosome assembly consists of three individual stages, all of which coexist at any given time within a cell [15]. These stages represent orderly cycles of association and dissociation from the androgen receptor [15].
One stage, thought of as the early stage in the process, is related to the association of HSP70 and HSP40 with the androgen receptor [18]. The next stage, considered the intermediate stage, involves binding of proteins such as HOP to HSP70 and further recruitment of other heat shock proteins, such as HSP90, which are thought to bind directly to the ligand-binding area (Region E) of the androgen receptor [17]. The third stage, referred to as the late or final stage, consists of dissociation from the receptor of HSP70, HSP40, and HOP. This dissociation step allows proteins such as HSP90 to interact with other key components of the foldosome, such as p23 and immunophilins listed above, to allow the receptor complex to achieve full ligand-binding activity [19]. At all times, the process remains in constant flux and each of these stages is unstable until ligand binding occurs [16]. Ligand binding triggers a chain of dissociation of chaperone proteins and the activation of the receptor complex [15].
Ligand binding, receptor activation, and transcriptional activation
The primary ligands for the androgen receptor are so-called androgenic compounds, including agonists, antagonists, and selective agonist-antagonist compounds (or selective androgen receptor modulators – SARMs) [5]. In addition, estrogenic, progestogenic, and other compounds can bind the androgen receptor [12]. For the purpose of this section, we will purely use the term “androgen” or “ligands” to represent any compound that has the ability to bind the androgen receptor.
Androgens do not need any cell surface receptor and are thought to diffuse freely into the cytosol [6]. The androgen binds to the receptor at the ligand-binding region (Region E). This binding process triggers a chain of physical changes to the receptor complex, which leads to dissociation of several components of the foldosome [15]. These changes free up areas in the hinge region of the receptor (Region D), which facilitates (or may be entirely responsible) for the transfer of the receptor complex to the nucleus [20,21]. The areas in the hinge region are called the nuclear localization sequence (NLS), since they allow the re-localization of the androgen receptor complex from the cytosol to the nucleus.
For many years, it was believed that steroid receptors were predominantly found in the nucleus at all times, and that this is where steroid binding occurred. This has now been disproven, with binding primarily occurring in the cytosol [6]. In a similar manner, the process by which the receptor-bound complex reaches the nucleus has evolved from the notion of a passive transfer to the nucleus to a complex and active one [6,22].
The foldosome appears to play a critical role in the transfer of the bound receptor complex to the nucleus, and continues to play a role when the receptor reaches the nucleus and binds to DNA [23]. Transport of the receptor complex to the nucleus appears to be mediated, or at the very least modulated, by elements of the foldosome such as HSP90, FKBP51, and FKBP52 [15,23,24]. An attractive model to explain these complex interactions is that transport of the receptor complex to the nucleus is linked to tubulin proteins, which essentially serve as a highway within the cell to transport bound receptors to the nucleus [22]. The foldosome components would then play a key role in association of the receptor complex to the tubulin proteins. This construct opens up a number of new therapeutic opportunities targeting the foldosome complex, as well as tubulin proteins. This will be discussed later in the chapter.
Once in the nucleus, a number of additional transformational steps occur, many of which involve further changes to the interaction between the remaining foldosome components and the bound androgen receptor.
At some point in the transition from the cytosol to the nucleus, the receptor complex binds to another activated receptor complex, a process referred to as dimerization. Since there are two types of androgen receptors, as stated above, dimers may consist of coupled AR-A or AR-B, so-called homodimers, or coupling of an AR-A and an AR-B, referred to as heterodimers [6]. The dimerization process is linked to physico-chemical changes that occur within the DNA-binding area (Region C) [6].
At or near the time of binding of the androgen receptor to the DNA androgen response element, much dynamic activity occurs. This is the predominant time when co-activators or co-repressors bind to the androgen receptor complex [6]. These interactions occur in the activation factor 1 area (Region A/B) as well as in the activation factor 2 area (Region E – which is also the ligand-binding region). There appears to be much competition among these regulatory proteins for their binding to the receptor complex, and it is clear that the ligand itself modulates which regulatory proteins are more likely to bind and upregulate or downregulate transcriptional activity. It also appears that the process is not a static one, where one set of factors associates with the receptor dimer complex and results in a set transcriptional activity, but much more of a dynamic state where regulatory factors associate and dissociate in a cyclic fashion [15]. Last but not least, components of the foldosome also appear to play a key modulatory role at this stage of the process [15].
As already stated, the androgen receptor dimer binds DNA at areas known as androgen response elements (AREs). Over 1500 AREs have been identified in the human genome. This clearly highlights the wide array of complex actions that androgens may exert. In addition, more than half of these ARE sites can bind the androgen receptor, but are about half the size of regular response elements, suggesting the potential for even more complex interactions occurring at these so-called half sites [25].
In addition to binding to DNA at AREs, the androgen receptor can bind to glucocorticoid response elements (GREs), just like the progesterone and mineralocorticoid receptors [26].
Co-activators and co-repressors
As already stated, a number of proteins modulate the ultimate transcriptional activity of any compound that binds the androgen receptor. These are divided into two groups, co-activators, which upregulate activity, and co-repressors, which downregulate activity (5,6]. As is the case for all other components of the androgen receptor system, these display a high level of complexity.
So far, many more co-activators have been found compared with co-repressors [11]. Classic co-activators include families of proteins described as SRC1, SRC2, and SRC3 [11]. A large number of additional co-activators continue to be identified, now numbering in the hundreds [11].
The mechanisms by which co-regulators exert their action continue to be the focus of much research [11]. They appear to regulate transcription through a wide variety of mechanisms, primarily targeting the configuration of DNA during the transcription process. The activity includes modulation of such key DNA-linked enzymes as histone acetyltransferase activity, histone deacetylase activity, arginine methyltransferase activity, ubiquitin ligase activity, and ATP-dependent chromatin-remodeling activity.
It is essential to grasp the concept that the ultimate effect of any given androgen depends on the complex interaction between the receptor, the components of the foldosome, and the balance of co-activators and co-repressors present in any particular cell at the time. Modulation of these co-activators and co-repressors, either from the ligand itself (e.g., agonist vs. SARM) or via the prevalent intracellular homeostasis, is critical to the ultimate physiologic effect seen. This system also lends itself to multi-site targeting for therapeutic purposes.
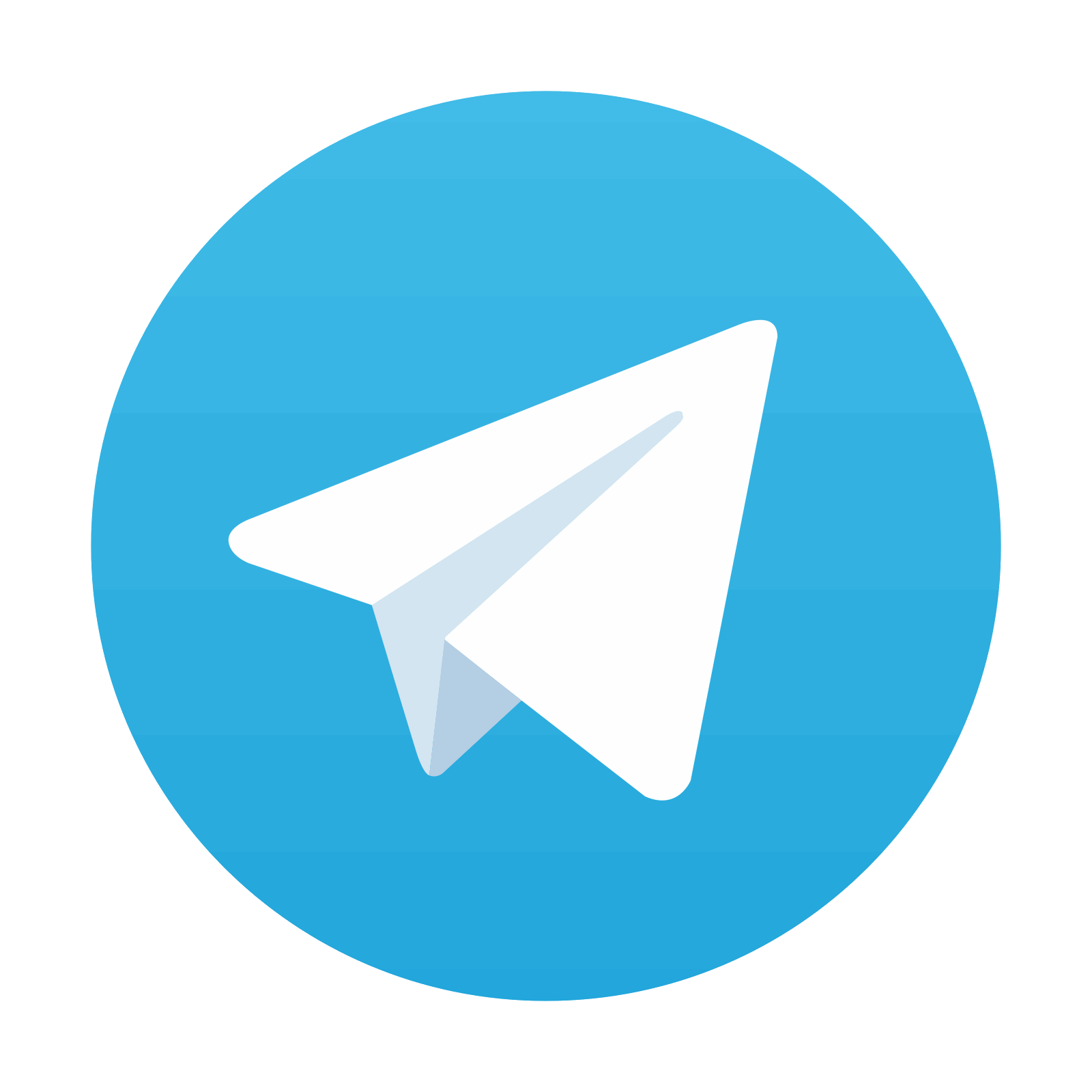
Stay updated, free articles. Join our Telegram channel

Full access? Get Clinical Tree
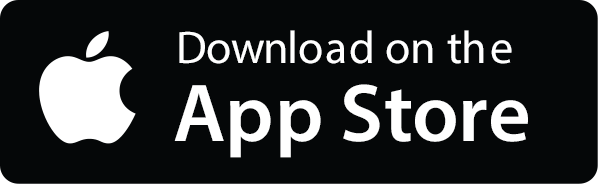
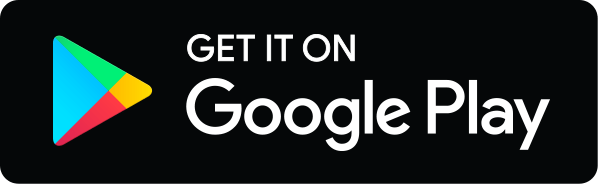