Acitretin
Alcohol
Ambrisentan
Angiotensin-converting enzyme inhibitors
Angiotensin-receptor blockers
Androgens
Bexarotene
Bosentan
Carbamazepine
Chloramphenicol
Cocaine
Corticosteroids
Cyclophosphamide
Danazol
Diethylstilbestrol (DES)
Efavirenz
Fluconazole
Isotretinoin
Lamotrigine
Lead
Leflunomide
Lenalidomide
Lithium
Methimazole
Mercury
Methotrexate
Misoprostol
Mycophenolate
Paroxetine
Phenobarbital
Phenytoin
Radioactive iodine
Ribavirin
Tamoxifen
Tetracycline
Thalidomide
Tobacco
Toluene
Topiramate
Trastuzumab
Tretinoin
Valproic acid
Warfarin
Although only a relatively small number of medications have proven harmful effects, there is significant concern surrounding medication use in pregnancy. This is because most pregnant women take medications and for most medications, available safety data are limited. In a review of more than 150,000 pregnancies, 40 percent of women were prescribed a medication other than multivitamins in the first trimester (Andrade, 2004). More recently, data from the National Birth Defects Prevention Study showed that women use an average of 2 to 3 medications per pregnancy and that 70 percent take medication in the first trimester (Mitchell, 2011).
Despite improvements in safety information, data are particularly limited for newer medications. For example, in a review of medications approved by the FDA between 2000 and 2010, the Teratogen Information System (TERIS) expert advisory board deemed the pregnancy risk “undetermined” for more than 95 percent (Adam, 2011).
TERATOLOGY
The study of birth defects and their etiology is termed teratology. The word teratogen is derived from the Greek teratos, meaning monster. Pragmatically, a teratogen may be defined as any agent that acts during embryonic or fetal development to produce a permanent alteration of form or function. Thus, a teratogen may be a drug or other chemical substance, a physical or environmental factor such as heat or radiation, a maternal metabolite such as in phenylketonuria or diabetes, a genetic abnormality, or an infection. Tightly defined, a teratogen causes structural abnormalities, whereas a hadegen—after the god Hades—is an agent that interferes with normal maturation and function of an organ. A trophogen is an agent that alters growth. Substances in the latter two groups typically affect development in the fetal period or after birth, when exposures are often more difficult to document. In most circumstances, teratogen is used to refer to all three types of agents.
Studies in Pregnant Women
The study of medication safety—or teratogenicity—in pregnant women is fraught with complications. Animal studies are considered necessary but insufficient, which is a lesson learned from the safety of thalidomide in several animal species. Rarely are medications approved by the FDA with the specific indication for their use being pregnancy. Between 1996 and 2011, for example, the only medication approved specifically for pregnancy was hydroxyprogesterone caproate for recurrent preterm birth prevention.
Pregnant women are generally considered a special population and excluded from trials. An obvious reason is to protect the embryo and fetus from potentially harmful effects of a medication—its pharmacodynamic effect(s) on the body. Another reason is that pregnancy physiology affects the medication—its pharmacokinetics. Specifically, changes in volume of distribution, cardiac output, gastrointestinal absorption, hepatic metabolism, and renal clearance may each affect drug concentration and thus embryo-fetal exposure (Chap. 4, p. 51).
Case Reports and Series
Several major teratogens were first described by astute clinicians. Congenital rubella syndrome was identified in this way by Gregg (1941), an Australian ophthalmologist whose observations challenged the view that the uterine environment was impervious to noxious agents. Other teratogens identified through case series include thalidomide and alcohol (Jones, 1973; Lenz, 1962). Unfortunately, teratogens are less likely to come to attention through identification of clinical cases if the exposure is uncommon, if the defects are relatively nonspecific, or if abnormalities occur in only a small proportion of exposed infants. A major limitation of case series is that they lack a control group.
Pregnancy Registries
Potentially harmful agents may be monitored by clinicians who prospectively enroll exposed pregnancies in a registry. The FDA maintains a list of ongoing pregnancy registries on their website: www.fda.gov. Included are medication groups used to treat asthma, autoimmune disease, cancer, epilepsy, human immunodeficiency virus (HIV) infection, and transplant rejection. Similar to case series, exposure registries are limited by lack of a control group. To compare the prevalence of an abnormality identified among exposed infants with that expected in the general population, investigators use a birth defect registry. One example is the Metropolitan Atlanta Congenital Defects Program, which is an active surveillance program ongoing since 1967 for fetuses and infants with birth defects.
Case-Control Studies
In these studies, investigators retrospectively assess prenatal exposure to particular substances between affected infants and controls. Birth defect registries are ideal for ascertainment of cases. However, case-control studies have two inherent weaknesses. First, there is potential for recall bias, in which the mother of an affected infant may be more likely to recall exposure. Second, case-control studies can only evaluate associations, rather than causality, and are thus hypothesis-generating. For these reasons, Grimes and Schulz (2012) caution that unless odds ratios in case-control studies are above three- to fourfold, then observed findings may be incorrect.
The National Birth Defects Prevention Study. This population-based case-control study involves 10 states with active birth defects surveillance programs (www.nbdps.org). It represents an important collaborative effort by the Centers for Birth Defects Research and Prevention to evaluate medications as a cause of birth defects. Since 1997, each center has annually enrolled 300 or more cases—including live births, stillbirths, and terminated pregnancies that have one or more of 30 structural birth defects, along with 100 randomly selected controls. Clinical geneticists review each potential case, and standardized telephone interviews are conducted 6 weeks and 24 months after delivery to obtain information regarding medication exposure and medical risk factors (Mitchell, 2011). This large volume has considerable statistical power and permits identification of relatively small associations.
As of 2012, the National Birth Defects Prevention Study (NBDPS) had identified associations between individual birth defects and the following classes of medications: sulfonamides and nitrofurantoin, asthma medications, antiemetics, nonsteroidal antiinflammatory drugs, and opioids (Broussard, 2011; Crider, 2009; Hernandez, 2012; Lin, 2012; Munsie, 2011). For each, the authors discussed the possibility that the observed associations may have been due to chance alone or that the underlying medical disorder for which the drug was given may have caused the abnormality. Based on the degree of risk identified, if any of these medications is eventually deemed teratogenic, it would be considered a low-risk teratogen, as subsequently discussed. Thus, the American College of Obstetricians and Gynecologists (2013b) considers nitrofurantoin and sulfonamides appropriate for first-trimester use if no suitable alternative is available, and they remain the first-line agents for treatment and prevention of urinary infections beyond the first trimester (Chap. 53, p. 1052).
CRITERIA FOR DETERMINING TERATOGENICITY
The guidelines shown in Table 12-2, which were proposed by Shepard (1994) as a framework for discussion, have proven useful for more than 20 years. Although all criteria may not be required to establish teratogenicity, the following tenets must be considered (Shepard, 2002a):
• The defect has been completely characterized. This is preferably done by a geneticist or dysmorphologist because various genetic and environmental factors may produce similar anomalies. It is easiest to prove causation when a rare exposure produces a rare defect, when at least three cases with the same exposure have been identified, and when the defect is severe.
• The agent must cross the placenta. Although almost all drugs cross the placenta, transport must be of sufficient quantity to directly influence embryonic or fetal development or to alter maternal or placental metabolism to exert an indirect effect. Placental transfer depends on maternal metabolism; on specific characteristics of the drug, such as protein binding and storage, molecular size, electrical charge, and lipid solubility; and on placental metabolism such as the cytochrome P450 enzyme systems. In early pregnancy, the placenta also has a relatively thick membrane that slows diffusion.
• Exposure must occur during a critical developmental period:
1. The preimplantation period is the 2 weeks from fertilization to implantation and is known as the “all or none” period. As the zygote undergoes cleavage, an insult damaging a large number of cells typically causes embryonic death. However, if only a few cells are injured, compensation may be possible, with normal development (Clayton-Smith, 1996). Based on animal data, insults that appreciably diminish the cell number in the inner cell mass may produce a dose-dependent diminution in body length or size (Iahnaccone, 1987).
2. The embryonic period is from the second through the eighth week. It encompasses organogenesis and is thus the most crucial period with regard to structural malformations. Critical developmental periods for each organ system are illustrated in Figure 12-1.
3. The fetal period, which is beyond 8 weeks, is characterized by continued maturation and functional development, and during this time, certain organs remain vulnerable. For example, brain development remains susceptible to environmental influences such as alcohol exposure throughout pregnancy.
• A biologically plausible association is supportive. Because birth defects and medication exposures are both common, they may be temporally but not causally related.
• Epidemiological findings must be consistent. Because initial evaluation of teratogen exposure is often retrospective, it may be hampered by recall bias, inadequate reporting, and incomplete assessment of the exposed population. Potential confounding factors include varying dosages, concomitant drug therapy, and maternal disease(s). Familial and environmental factors can also influence development of birth defects. Thus, an important criterion for teratogenicity is that two or more high-quality epidemiological studies report similar findings. Finally, a relative risk of 3.0 or greater is generally considered necessary to support the hypothesis, whereas a lesser risk is interpreted with caution (Khouri, 1992).
• The suspected teratogen causes a defect in animal studies. Human teratogenicity is more likely if an agent produces an adverse effect in different animal species. However, a major lesson was learned from the thalidomide tragedy in that its teratogenicity was not recognized because of reliance on animal data, in which thalidomide produced no defects in several animal species. Not until thousands of infants were affected by thalidomide embryopathy was it realized that this agent was a potent human teratogen.
TABLE 12-2. Criteria for Determining Teratogenicity
Essential Criteria:
1. Careful delineation of clinical cases, particularly if there is a specific defect or syndrome
2. Proof that exposure occurred at critical time during development (see Fig. 12-1)
3. Consistent findings by at least two epidemiological studies with:
a. exclusion of bias,
b. adjustment for confounding variables,
c. adequate sample size (power),
d. prospective ascertainment if possible, and
e. relative risk (RR) of 3.0 or greater, some recommend RR of 6.0 or greater
or
For a rare environmental exposure associated with a rare defect, at least three reported cases. This is easiest if defect is severe
Ancillary Criteria:
4. The association is biologically plausible
5. Teratogenicity in experimental animals is important but not essential
6. The agent acts in an unaltered form in an experimental model
FIGURE 12-1 Timing of organogenesis during the embryonic period. (Redrawn from Sadler, 1990, with permission.)
Failure to employ these tenets and criteria has contributed to erroneous conclusions regarding the safety of some widely used drugs. The poster child for this is the medicolegal fiasco surrounding Bendectin. This antiemetic was a combination of doxylamine and pyridoxine that was both safe and effective for nausea and vomiting common in early pregnancy. More than 30 million women used this drug worldwide, and the 3-percent congenital anomaly rate among exposed fetuses was not different from the background rate (McKeigue, 1994). Despite evidence that the drug was not teratogenic, Bendectin was the target of numerous lawsuits, and the financial burden of defending these forced its withdrawal. As a consequence, hospitalizations for hyperemesis doubled (Koren, 1998). Ironically, the combination of doxylamine and pyridoxine has now been recently remarketed under the brand name Diclegis, which was FDA approved in 2013.
COUNSELING FOR TERATOGEN EXPOSURE
Questions regarding medication and illicit drug use should be part of routine preconceptional and prenatal care. Women who request counseling for prenatal drug exposure often have misinformation regarding their level of risk. Not uncommonly, they may underestimate the background risk for birth defects in the general population and exaggerate the potential risks associated with medication exposure. Koren and colleagues (1989) reported that a fourth of women exposed to nonteratogenic drugs thought they had a 25-percent risk for fetal anomalies. Misinformation may be amplified by inaccurate reports in the lay press. Knowledgeable counseling may allay anxiety considerably and in some situations may even avoid pregnancy termination.
The Food and Drug Administration Classification System
This system for evaluating drug safety in pregnancy was developed in 1979. It was designed to provide therapeutic guidance by using five categories—A, B, C, D, or X, shown in Table 12-3, to simplify risk-benefit information. In its present form, the system has important limitations, and these have been acknowledged by the FDA. One is that drugs in categories D and X, and some in category C, may pose similar risks but are in different categories because of different risk-benefit considerations. A higher letter grade does not necessarily confer an increased risk, and even drugs in the same category may have very different risks! And, despite the understanding that medications may affect human and animal development differently, the letter category is often based on animal data.
TABLE 12-3. Food and Drug Administration Categories for Drugs and Medications
Category A: Studies in pregnant women have not shown an increased risk for fetal abnormalities if administered during the first (second, third, or all) trimester(s) of pregnancy, and the possibility of fetal harm appears remote.
Fewer than 1 percent of all medications are in this category. Examples include levothyroxine, potassium supplementation, and prenatal vitamins, when taken at recommended doses.
Category B: Animal reproduction studies have been performed and have revealed no evidence of impaired fertility or harm to the fetus. Prescribing information should specify kind of animal and how dose compares with human dose.
or
Animal studies have shown an adverse effect, but adequate and well-controlled studies in pregnant women have failed to demonstrate a risk to the fetus during the first trimester of pregnancy, and there is no evidence of a risk in later trimesters.
Examples include many antibiotics, such as penicillins, most cephalosporins, and macrolides.
Category C: Animal reproduction studies have shown that this medication is teratogenic (or embryocidal or has other adverse effect), and there are no adequate and well-controlled studies in pregnant women. Prescribing information should specify kind of animal and how dose compares with human dose.
or
There are no animal reproduction studies and no adequate and well-controlled studies in humans.
Approximately two thirds of all medications are in this category. It contains medications commonly used to treat potentially life- threatening medical conditions, such as albuterol, zidovudine, and calcium-channel blockers.
Category D: This medication can cause fetal harm when administered to a pregnant woman. If this drug is used during pregnancy or if a woman becomes pregnant while taking this medication, she should be apprised of the potential hazard to the fetus.
This category also contains medications used to treat potentially life-threatening medical conditions, for example: corticosteroids, azathioprine, carbamazepine, and lithium.
Category X: This medication is contraindicated in women who are or may become pregnant. It may cause fetal harm. If this drug is used during pregnancy or if a woman becomes pregnant while taking this medication, she should be apprised of the potential hazard to the fetus.
There are a few medications in this category that have never been shown to cause fetal harm but should be avoided nonetheless, such as the rubella vaccine.
From the foregoing, it follows that it may be insufficient or even inappropriate to rely on this classification to make complex therapeutic decisions in pregnant women. Rather than simplifying counseling, the letter classification shifts responsibility to the clinician, who must interpret category information in the context of medication dosage, route and timing of exposure, other medications used, and underlying medical condition(s).
Because of the limitations of its current classification, the FDA (2011b) has proposed a new system for labeling drugs for use by pregnant and lactating women. Letter categories A through X are to be replaced with: (1) a fetal risk summary, (2) a section on clinical considerations—including inadvertent exposure, (3) a section on prescribing decisions for pregnant and lactating women, and (4) a detailed discussion of human and animal data. It is anticipated that this evidence-based rating system may soon become available (Gee, 2014).
The FDA has also expanded the information available on its website: www.fda.gov. The website includes updated, detailed information regarding potentially harmful medications—in the form of drug advisories, registries, and product information. It is an excellent resource for counseling. Current, accurate information is also available through online reproductive toxicity services such as Reprotox and TERIS.
Presenting Risk Information
In addition to potential embryonic and fetal risks from drug exposure, counseling should include a discussion of the risks and/or genetic implications of the underlying condition for which the drug is given, as well as risks associated with not treating the condition. Even the manner in which information is presented affects perception. Jasper and associates (2001) found that women given negative information—such as a 2-percent chance of a malformed infant—are more likely to perceive an exaggerated risk than women given positive information—such as a 98-percent chance of a child without a malformation. Instead of citing an increased odds ratio, it may be helpful to provide the absolute risk for a particular defect or the attributable risk, which is the difference between prevalence in exposed and unexposed individuals (Conover, 2011). The association between oral corticosteroid medications and cleft lip sounds far more concerning when presented as a tripling or 200-percent increase in risk than when presented as an increase from 1 per 1000 to 3 per 1000, or a 99.7-percent likelihood of not developing a cleft following exposure.
With a few notable exceptions, most commonly prescribed drugs and medications can be used with relative safety during pregnancy. All women have an approximate 3-percent chance of having an infant with a birth defect. Although exposure to a confirmed teratogen may increase this risk, the magnitude of the increase is usually only 1 or 2 percent or at most, doubled or tripled. The concept of risk versus benefit is often central to counseling. Some untreated diseases pose a more serious threat to both mother and fetus than medication exposure risks.
There undoubtedly are a number of major teratogens. However, many drugs described in this chapter are low-risk teratogens, which are medications that produce defects in fewer than 10 per 1000 maternal exposures (Shepard, 2002a). Some examples include corticosteroids, lithium, trimethoprim, and methimazole. Because risks conferred by low-risk teratogens are so close to background, they may not be a major factor in deciding whether to discontinue treatment for an important condition (Shepard, 2002b). Obviously, clinicians and patients must carefully weigh risks and benefits in these instances.
GENETIC AND PHYSIOLOGICAL SUSCEPTIBILITY TO TERATOGENS
Teratogens act by disturbing specific physiological processes, which may in turn lead to abnormal cellular differentiation, altered tissue growth, or cell death. Because pathophysiological processes may be induced in various cell types and tissues, exposure may result in multiple effects, and because different teratogens may disturb similar processes, they may produce similar phenotypic abnormalities. But even the most potent teratogen induces birth defects in only a fraction of exposed embryos. Despite the numerous factors that influence exposure, teratogens appear merely to have potential to cause birth defects. The reasons why some infants are affected and others are not remains largely unknown.
Fetal Genome
In some cases, genetic composition has been linked to susceptibility to teratogenic effects of specific medications. For example, fetuses exposed to hydantoin are more likely to develop anomalies if homozygous for a gene mutation that results in abnormally low levels of epoxide hydrolase (Buehler, 1990). If activity of epoxide hydrolase enzyme is reduced, then hydantoin, carbamazepine, and phenobarbital are metabolized by microsomes to oxidative intermediates that accumulate in fetal tissues (Horning, 1974). These free oxide radicals have carcinogenic, mutagenic, and other toxic effects that are dose related and increase with multidrug therapy (Buehler, 1990; Lindhout, 1984).
Disruption of Folic Acid Metabolism
Fetal neural-tube defects, cardiac defects, and oral clefts can be a result of folic acid metabolic pathway disturbances. Folates are essential for methionine production, which is required for gene methylation and thus production of proteins, lipids, and myelin. Some anticonvulsants—phenytoin, carbamazepine, valproic acid, and phenobarbital—either impair folic acid absorption or act as folic acid antagonists. The resultant low periconceptional folic acid levels in some women with epilepsy may then cause fetal malformations (Dansky, 1987; Hiilesmaa, 1983). In one study of more than 5000 infants with birth defects, exposure to these folic acid antagonists was associated with a two- to threefold increased risk for oral clefts and cardiac abnormalities (Hernandez-Diaz, 2000).
Some congenital heart defects are related to interactions between folate-related genes and environmental or genetic factors. In a case-control study with more than 500 infants with a cardiac defect, maternal polymorphisms in three folate-related genes were found to increase the risk for cardiac anomalies when combined with maternal cigarette smoking, alcohol consumption, or obesity (Hobbs, 2010). Data from the National Down Syndrome Project demonstrated that trisomy 21 fetuses born to women not given periconceptional folic acid supplementation may be more likely to have endocardial cushion defects (Bean, 2011).
Paternal Exposures
In some cases, paternal exposures to drugs or environmental influences may increase the risk of adverse fetal outcome. Proposed mechanisms include induction of a gene mutation or chromosomal abnormality in sperm. Because of the 64 days in which male germ cells mature into functional spermatogonia, drug exposure during the 2 months before conception could cause gene mutations. It may be that epigenetic pathways suppress germ-cell apoptosis or interfere with imprinting (Cordier, 2008). Another possibility is that during intercourse the developing embryo is exposed to a teratogenic agent in seminal fluid.
There is some evidence to support these hypotheses. For example, ethyl alcohol, cyclophosphamide, lead, and certain opiates have been associated with an increased risk of behavioral defects in the offspring of exposed male rodents (Nelson, 1996). In humans, paternal environmental exposure to mercury, lead, solvents, pesticides, anesthetic gases, or hydrocarbons may be associated with early pregnancy loss (Savitz, 1994). In a 20-year study of Swedish university employees, paternal exposure to carcinogenic solvents resulted in offspring with more than a fourfold increase in neural-crest malformations (Magnusson, 2004). Other occupations associated with an increased risk for men to have anomalous offspring include janitors, woodworkers, firemen, printers, and painters (Olshan, 1991; Schnitzer, 1995). Risk attribution is limited because assessment of paternal exposure is often imprecise, and there is potential for simultaneous maternal exposure, particularly for environmental agents such as pesticides (Cordier, 2008).
KNOWN AND SUSPECTED TERATOGENS
The number of medications and other substances strongly suspected or proven to be human teratogens is small, as shown in Table 12-1. With few exceptions, in every clinical situation potentially requiring therapy with a known teratogen, alternative drugs can be given with relative safety. As a general rule, because there are no adequate and well-controlled studies in pregnant women for most medications, and because animal reproduction studies are not always predictive of human response, any medication in pregnancy must be carefully considered and only used if clearly needed.
Alcohol
Ethyl alcohol is a potent and prevalent teratogen. Alcohol is one of the most frequent nongenetic causes of mental retardation as well as the leading cause of preventable birth defects in the United States. According to the Centers for Disease Control and Prevention (CDC)(2012), 8 percent of women report drinking alcohol during pregnancy, and the NBDPS identified a prevalence as high as 30 percent (Ethen, 2009). Between 1 and 2 percent of pregnant women admit to binge drinking.
The fetal effects of alcohol abuse have been recognized since the 1800s. Lemoine (1968) and Jones (1973) and their coworkers are credited with describing the spectrum of alcohol-related fetal defects known as fetal alcohol syndrome (Table 12-4). The Institute of Medicine (1996) has estimated that prevalence of the syndrome ranges from 0.6 to 3 per 1000 births.
TABLE 12-4. Fetal Alcohol Syndrome and Alcohol-Related Birth Defects
Fetal Alcohol Syndrome Diagnostic Criteria—all required
1. Dysmorphic facial features (all 3 are required)
a. Small palpebral fissures
b. Thin vermilion border
c. Smooth philtrum
2. Prenatal and/or postnatal growth impairment
3. Central nervous system abnormalities (1 required)
a. Structural: head size < 10th percentile, significant brain abnormality on imaging
b. Neurological
c. Functional: global cognitive or intellectual deficits, functional deficits in at least three domains
Alcohol-Related Birth Defects
1. Cardiac: atrial or ventricular septal defect, aberrant great vessels, conotruncal heart defects
2. Skeletal: radioulnar synostosis, vertebral segmentation defects, joint contractures, scoliosis
3. Renal: aplastic or hypoplastic kidneys, dysplastic kidneys, horseshoe kidney, ureteral duplication
4. Eyes: strabismus, ptosis, retinal vascular abnormalities, optic nerve hypoplasia
5. Ears: conductive or neurosensory hearing loss
6. Minor: hypoplastic nails, clinodactyly, pectus carinatum or excavatum, camptodactyly, “hockey stick” palmar creases, refractive errors, “railroad track” ears
For every child with fetal alcohol syndrome, many more are born with neurobehavioral deficits from alcohol exposure (American College of Obstetricians and Gynecologist, 2013a). Fetal alcohol spectrum disorder is an umbrella term that includes the full range of prenatal alcohol damage that may not meet the criteria for fetal alcohol syndrome. Prevalence of this disorder is estimated to be as high as 1 percent of births in the United States (Centers for Disease Control, 2012; Guerri, 2009).
Clinical Characteristics
Fetal alcohol syndrome has specific criteria, listed in Table 12-4, and which include dysmorphic facial features, pre- or postnatal growth impairment, and central nervous system abnormalities that may be structural, neurological, or functional (Bertrand, 2005). The distinctive facial features are shown in Figure 12-2. Other alcohol-related major and minor birth defects include cardiac and renal anomalies, orthopedic problems, and abnormalities of the eyes and ears. An association has also been reported between periconceptional alcohol use and omphalocele and gastroschisis (Richardson, 2011). There are no established criteria for prenatal diagnosis of fetal alcohol syndrome, although in some cases, major abnormalities or growth restriction may be suggestive (Paintner, 2012).
FIGURE 12-2 Fetal alcohol syndrome. A. At 2½ years. B. At 12 years. Note persistence of short palpebral fissures, epicanthal folds, flat midface, hypoplastic philtrum, and thin upper vermilion border. (From Streissguth, 1985, with permission.)
Dose Effect
Fetal vulnerability to alcohol is modified by genetic factors, nutritional status, environmental factors, coexisting maternal disease, and maternal age (Abel, 1995). The minimum amount of alcohol required to produce adverse fetal consequences is unknown. Binge drinking, however, is believed to pose particularly high risk for alcohol-related birth defects and has also been linked to an increased risk for stillbirth (Centers for Disease Control, 2012; Maier, 2001; Strandberg-Larsen, 2008).
Anticonvulsant Medications
Pragmatically, no anticonvulsant drugs are considered truly “safe” in pregnancy. This is because most medications used to treat epilepsy have been proven or suspected to confer an increased risk for fetal malformations. The North American Antiepileptic Drug (NAAED) Pregnancy Registry was established to improve counseling information. Providers are encouraged to enroll pregnant women treated with antiepileptic medication through the FDA website or 1–888–233–2334.
Management of epilepsy in pregnancy, including risks associated with individual anticonvulsants, is discussed in Chapter 60 (p. 1190). Traditionally, women with epilepsy were informed that their risk for fetal malformations was increased two- to threefold. More recent data suggest that the risk may not be as great as once thought, particularly for newer agents. In a recent population-based study involving more than 800,000 pregnancies, exposure to newer anticonvulsants was associated with a 3-percent major malformation risk, compared with a 2-percent risk in unexposed fetuses (Molgaard-Nielsen, 2011). Similarly, the United Kingdom Epilepsy and Pregnancy Registry reported a 3-percent risk for major malformations in pregnancies treated with a single anticonvulsant—monotherapy. This is the same malformation rate as for those with untreated epilepsy (Morrow, 2006). There is one important exception to these findings. Women treated with valproic acid are at significantly increased risk for malformations as subsequently discussed.
The most frequently reported anomalies are orofacial clefts, cardiac malformations, and neural-tube defects (Food and Drug Administration, 2009). Several older anticonvulsants produce a constellation of malformations similar to the fetal hydantoin syndrome (Fig. 12-3). Of agents in current use, valproic acid confers the greatest risk. The NAAED Pregnancy Registry reported that major malformations occurred in 9 percent of fetuses with first-trimester valproate exposure and included a 4-percent risk for neural-tube defects (Hernandez-Diaz, 2012). Moreover, children with in utero exposure to valproic acid are reported to have significantly lower intelligence quotient (IQ) scores at age 3 years compared with scores of those exposed to phenytoin, carbamazepine, or lamotrigine (Meador, 2009). Of the newer agents, topiramate has recently been reported by the NAAED Pregnancy Registry and the NBDPS to confer a risk for orofacial clefts at least fivefold higher than in unexposed pregnancies (Food and Drug Administration, 2011c; Margulis, 2012). Regardless of the anticonvulsant medication used, specialized sonography should be considered.
FIGURE 12-3 Fetal hydantoin syndrome. A. Facial features including upturned nose, mild midfacial hypoplasia, and long upper lip with thin vermilion border. B. Distal digital hypoplasia. (From Buehler, 1990, with permission.)
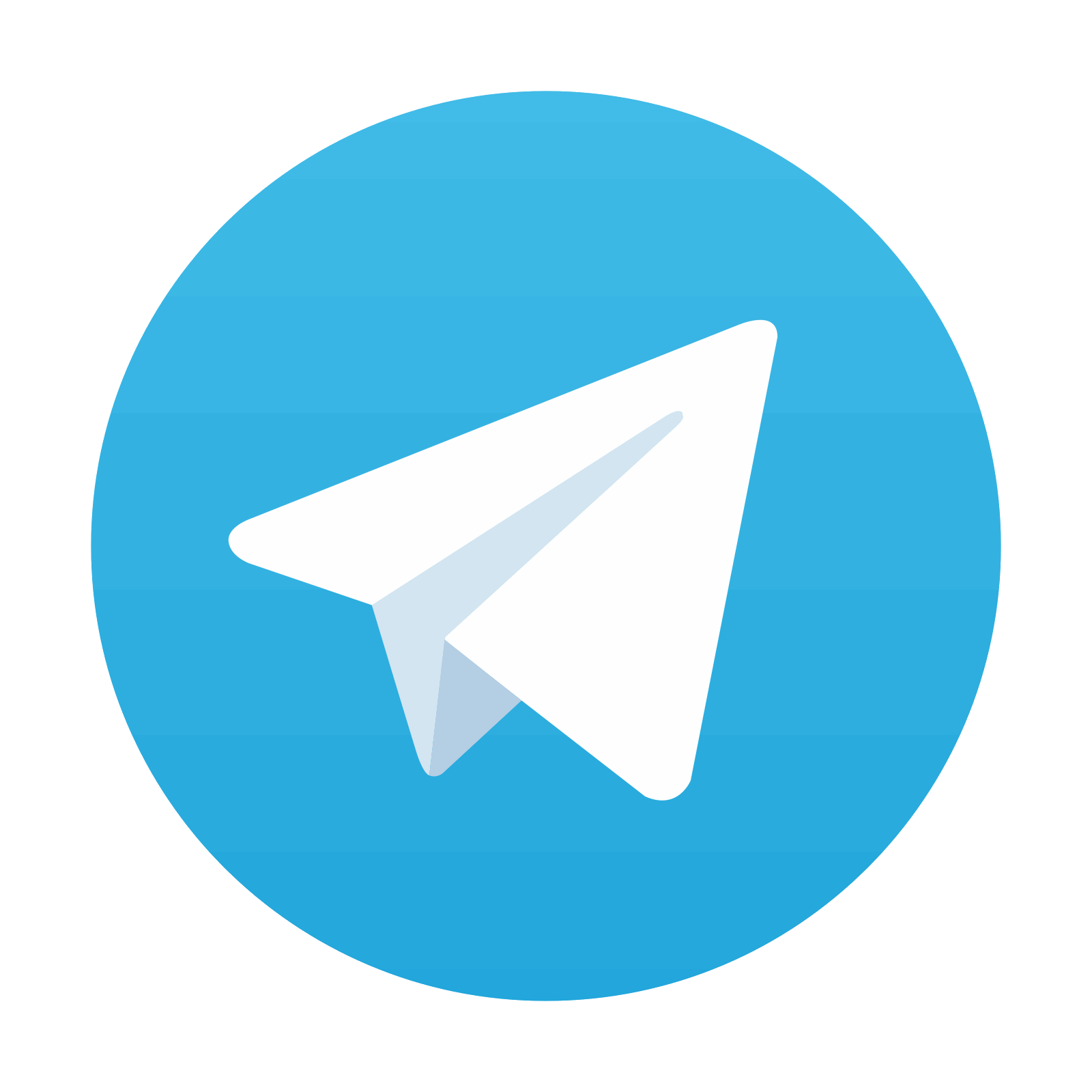
Stay updated, free articles. Join our Telegram channel

Full access? Get Clinical Tree
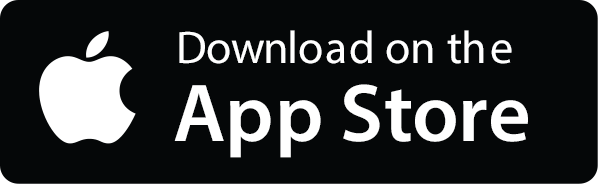
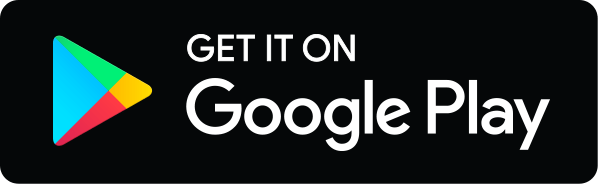