The impact of hyperthermia prevention and induced hypothermia to minimize neurologic injury has been evaluated in both animal models and clinical trials across multiple types of brain injury. Both spontaneous hyperthermia and spontaneous hypothermia are associated with worse outcomes following brain injury (1). Therapeutic temperature management has been evaluated in neonates, children, and adults and in multiple etiologies of neurologic injury including: hypoxia ischemia, traumatic brain injury (TBI), cardiac arrest, and stroke. However, to date, the only pediatric population that may benefit from induced hypothermia is neonates following asphyxia.
To safely utilize therapeutic temperature control, whether aiming to achieve controlled normothermia or induced hypothermia, the clinician must understand the pathophysiology of the specific brain injury and the risks and benefits of therapeutic temperature control. It is paramount to consider the etiology of the underlying brain injury, the goal temperature range and duration, and the means by which to achieve the goal temperature and minimize side effects.
■ HISTORY
Some of the first descriptions of the benefits of hypothermia go back to the Baron Larrey (2) who described that during the Napoleonic wars wounded soldiers who were put close to a campfire died earlier than those who were not rewarmed. In the 1950s hypothermia was used in conjunction with anesthesia for neuroprotection during cardiac surgery. Patients were placed in large tubs of ice to reduce the body temperature and metabolic rate. In the early 1960s hypothermia was frequently used following resuscitation from cardiac arrest. At low temperature levels (28°C–32°C), multiple complications were observed including arrhythmia, bleeding, and infection. For comatose pediatric drowning victims, HYPER therapy, which consisted of multiple therapeutic modalities including induced hypothermia to 30 +/− 1°C, was utilized to minimize brain edema (3). Systematic evaluation of this therapy in the late 1970s to early 1980s showed HYPER therapy to be deleterious with worse mortality in those who were cooled (4). Induced hypothermia for treatment of pediatric brain injury fell out of favor due to concerns that it might be harmful.
By the mid 1980s mild induced hypothermia was “rediscovered.” Peter Safar (5–7) and his trainees evaluated multiple animal models of induced hypothermia and repeatedly found functional and histologic benefits in the cooled group (32–34°C). These studies are the foundation for the recent clinical trials evaluating temperature control for the treatment of brain injury.
■ CELLULAR PATHOPHYSIOLOGY
To understand the impact of temperature management on the brain injured patient it is important to understand the cellular pathophysiology of primary brain injury and the impact of secondary brain injury. While TBI, stroke, and global hypoxic-ischemic injury are mechanistically different, the resultant injuries culminate in cellular necrosis and apoptosis. Therapeutic temperature management attempts to minimize these pathologic processes.
Global Hypoxic Ischemic
Global hypoxic-ischemic injury is defined by a period of primary injury when the brain has inadequate oxygen and blood flow. Secondary injury occurs following a period of reperfusion when blood flow and oxygen delivery are restored. Reperfusion injury may be as severe as or even worse than the initial ischemic injury. Clinically, during the initial ischemic injury, there is loss of consciousness and electroencephalography rapidly becomes flat. On the cellular level, intracellular calcium increases, interstitial potassium increases, intracellular adenosine triphosphate (ATP) decreases, cellular pH decreases, and there is an influx of water and calcium into cells (8–9). With reinstitution of blood flow there is initial cerebral hyperemia and then subsequent cerebral hypoperfusion. Intracellular and extracellular electrolytes are rapidly restored. However, ATP is not as readily repleted. Cellular death is potentiated by caspases, which lead to ongoing apoptosis, and calpains, which lead to neuronal necrosis.
Traumatic Brain Injury
TBI consists of primary injury during which the brain parenchyma is directly damaged. While this injury can be prevented through safety measures, it cannot be reversed. Secondary brain injury occurs in the time period following primary injury and may be reversible and preventable. Secondary injury is caused by low cerebral blood flow, excitotoxic injury including glutamate release and calcium influx, oxidative stress, and inflammation causing cytotoxic edema resulting in cellular necrosis and apoptosis (10). Physiologic abnormalities in patients following brain injury such as high or low levels of partial pressure of arterial oxygen or carbon dioxide, hypotension, and temperature derangements may exacerbate and potentiate secondary injuries.
Impact of Temperature on the Injured Brain
Fever following brain injury has been shown to potentiate secondary brain injury. Fever increases metabolic rate, neuronal excitotoxicity, proinflammatory cytokines, glutamate levels, intracellular acidosis, free radical production, and apoptosis (11–15). These processes can increase damage in ischemic tissue leading to infarction (16). Animal models of induced hyperthermia following either focal ischemia or global asphyxial injury resulted in worse outcomes (16–18). In contrast, prevention of fever by maintaining normothermia can decrease the severity of these processes (19–22).
Induced hypothermia may decrease brain injury by ameliorating the cascade of events that are ongoing during secondary injury. Induced hypothermia decreases cerebral metabolism by 6% to 10% for each 1°C decrease in body temperature (23). Following ischemia and reperfusion injury, hypothermia decreases glutamate release and neuronal injury (24), minimizes free radical production and decreases inflammation and apoptosis (25).
Hyperthermia further exacerbates reperfusion injury from stroke and hypoxia ischemia and secondary injury following TBI. Maintaining normothermia to prevent fever can minimize the ongoing processes that result in apoptosis and necrosis. Inducing hypothermia, even prior to restoration of blood flow following cardiac arrest, may substantially decrease reperfusion injury. Delayed initiation of hypothermia, even up to 20 minutes after achievement of return of spontaneous circulation in an animal model of cardiac arrest, was associated with worse outcomes (6,26).
■ CLINICAL IMPACT OF TEMPERATURE
Hyperthermia’s Ill Effects
Fever is common following brain injury and may occur spontaneously or when trying to warm the hypothermic patient. Up to 70% of neurologically injured patients have fever in the days following the primary injury, but only half of these patients have an infectious cause (27,28). Fever is common following TBI, intracerebral hemorrhage, focal stroke, and subarachnoid hemorrhage and may be due to thermoregulatory centers damage, inflammation, or blood within the intraventricular and cerebral spinal fluid containing spaces (29–30).
Following pediatric cardiac arrest, hyperthermia is common (1,31). The 2010 American Heart Association cardiopulmonary resuscitation (CPR) and emergency cardiovascular (ECC) guidelines recommend that “providers should closely monitor patient core temperature after return of spontaneous circulation and actively intervene to avoid hyperthermia” (32). In one study, following in-hospital cardiac arrest, almost half of children had one temperature > 38°C in the first 24 hours following return of spontaneous circulation (1). Fever was associated with unfavorable outcome in these patients. Following resuscitation from adult cardiac arrest, patients with hyperthermia were more likely to progress to brain death, and in those who survived, neurologic outcome was worse (33–37). Following neonatal asphyxia injury, for every 1°C increase in temperature above normothermia, infants had a 3.6 to 4 times increase in disability and mortality (38).
Following TBI, fever worsens intracranial pressure, prolongs intensive care unit hospitalization, and is associated with worse outcomes (39–42). Following pediatric TBI, early hyperthermia is associated with worse discharge neurologic outcomes (43).
To date, the most extensively studied impact of fever and fever prevention on neurologic outcome has been in the ischemic stroke population. Following focal ischemic stroke in adults, fever within the first 24 hours of injury is associated with worse neurologic outcome and larger cerebral infarcts (44). Two meta-analyses of ischemic stroke patients found that fever significantly increased morbidity and mortality (42,45). Similar findings have been reported in patients with subarachnoid hemorrhage and other forms of intracranial hemorrhage (42,46).
The high incidence of fever following neurologic injury and its association with morbidity and mortality have lead to the conclusion that fever should be treated and preferably prevented. Despite these conclusions, patients often become febrile following injury as clinicians are often more reactive than proactive. Steps to prevent fever and maintain normothermia should be taken. Because hyperthermia is detrimental to potential cerebral recovery, hypothermia to mitigate ongoing injury has been clinically evaluated in certain populations.
Induced Hypothermia’s Potential Benefits
Induced hypothermia to improve neurologic outcome and prevent secondary injury has been evaluated following cardiac arrest, perinatal asphyxia, TBI, and ischemic stroke. Adult and pediatric populations have been evaluated separately because the etiology of events and age-dependent physiology differ. We will review the clinical investigations performed for these disease processes and how they have impacted current clinical care.
Adult Cardiac Arrest
In 2010 the American Heart Association, following the International Liaison Committee on Resuscitation’s evidence evaluation process, recommended cooling all unconscious adults following successful resuscitation from an out-of-hospital ventricular fibrillatory (VF) cardiac arrest (47). These guidelines were formed based on two landmark papers published in 2002 evaluating the use of induced hypothermia following witnessed adult out-of-hospital VF arrest. Bernard et al (48) randomized 77 patients to either normothermia or hypothermia (33°C for 12 hours). Forty-nine percent (21/43) of patients in the hypothermia group had a good outcome (discharge to home or a rehab facility) as compared to 26% (9/34) patients in the control group (odds ratio [OR], 5.25; 95% confidence interval [CI], 1.47–18.76; P = .046). The hypothermia after cardiac arrest (HACA) study randomized 273 patients to hypothermia (32°C–34°C) for 24 hours or normothermia (49). Good neurologic outcome was defined as a 6 month Cerebral Performance Category of 1 or 2 (normal or mild dysfunction). Fifty-three percent (75/136) of subjects in the hypothermic arm had a good outcome as compared to 36% (54/137) of subjects in the control group (OR, 1.4; 95% CI, 1.08–1.81; P = .009). Of note, approximately 25% of subjects in the normothermic arm had bladder temperatures above 38°C. Some experts have questioned whether this was a testament to the benefits of hypothermia or harms of hyperthermia. Despite these studies’ shortcomings, induced hypothermia following adult VF arrest is now considered standard of care (47).
Induced hypothermia for treatment of adult cardiac arrest due to nonshockable rhythms has not been as rigorously evaluated and results from retrospective evaluations are equivocal. One large retrospective evaluation of out-of-hospital cardiac arrest patients treated with induced hypothermia showed improvement in neurologic outcomes in those with an initial shockable rhythm, but no benefit in those with initial asystole/pulseless electrical activity (PEA) (50) Another retrospective study of induced hypothermia to treat adults following asystole/PEA concluded that hypothermia improved neurologic outcome and mortality (51). One small prospective randomized controlled trial evaluating induced hypothermia following adult asystole/PEA showed an outcome benefit, however, it was underpowered and not designed to evaluate an outcome difference (52). A Cochrane Review of induced hypothermia following adult cardiac arrest concluded the hypothermia was beneficial for adult VF/VT arrest. Due to small numbers, no recommendations could be made regarding in-hospital arrest, nonshockable rhythms, and cardiac arrest of noncardiac origin (53).
Pediatric Cardiac Arrest
To date, there are no published randomized controlled trials evaluating the efficacy of induced hypothermia following pediatric cardiac arrest. Current published evaluations are limited and show no benefit of induced hypothermia following pediatric cardiac arrest. The American Heart Associations’ 2010 recommendation is: “Therapeutic hypothermia (32°C–34°C) may be considered for children who remain comatose after resuscitation from cardiac arrest. It is reasonable for adolescents resuscitated from sudden, witnessed, out-of-hospital VF cardiac arrest” (54). This statement is in part extrapolated from the adult data presented above.
Two recent retrospective studies compared induced hypothermia and normothermia in children successfully resuscitated from pediatric cardiac arrest (55,56). Both studies showed that there was no benefit to treating patients with induced hypothermia once controlling for potential confounders. Doherty et al (56) performed a multicenter retrospective cohort study across five centers, of which only three utilized hypothermia. Eighty-eight percent of the study population was children with underlying heart disease and 94% of the arrests were in-hospital. There was variability in the temperature patients were cooled to (33.7 +/− 1.3°C) and the duration they were maintained at that temperature (20.8 +/− 11.9 hours). Despite baseline differences between the hypothermia and normothermia groups, there were no was difference in outcome after controlling for multiple variables. Fink et al (57) evaluated a single center’s retrospective experience with induced hypothermia for primarily asphyxia-associated cardiac arrests. Only 8% of patients had underlying heart disease and only 9% suffered in-hospital arrests. There was no standard protocol for induction, maintenance, or rewarming. While they observed no significant difference in outcome between the normothermic and hypothermic groups, it was evident that patients treated with hypothermia had suffered more severe injury, as reflected in their longer durations of cardiac arrest and more epinephrine doses required to obtain return of spontaneous circulation. Both of these studies provide important initial assessments of induced hypothermia following pediatric cardiac arrest, but are limited by their retrospective approach and lack of explicit protocols.
A third study systematically evaluated the feasibility of a standard algorithm for induction and maintenance of induced hypothermia following pediatric cardiac arrest using a surface cooling protocol (58). Following resuscitation from cardiac arrest children were cooled to 32°C to 34°C using a standardized surface protocol and achievement of goal temperatures, and side effects were observed. Rectal temperature of ≤ 34°C was achieved in 1.5 hours from cooling initiation. Approximately 80% of the time patients were maintained between 32°C and 34°C. Overshoot hypothermia (< 32°C) was observed in 15% of measurements, a finding that was common in an adult study as well (58,59). Other expected and observed side effects of hypothermia were hypokalemia (67%) and bradycardia (< second percentile for age in 58%). However, there were no episodes of bleeding or ventricular tachyarrhythmia that required treatment. Six of 12 (50%) patients survived to discharge (58). This study was small and was not designed to evaluate the efficacy of induced hypothermia to improve neurologic outcome after cardiac arrest.
Despite protocols to induce and maintain hypothermia in children following cardiac arrest, to date, it has not been shown to be an efficacious therapy to prevent neurologic morbidity and mortality. A multicenter randomized clinical trial (Therapeutic Hypothermia After Pediatric Cardiac Arrest, www.thapca.org) is underway comparing induced hypothermia (32ºC–34°C) to controlled normothermia (36°C–37.5°C).
Perinatal Asphyxia
Induced hypothermia has been most rigorously studied in the neonatal population following birth asphyxia. Shankaran et al randomized moderately or severely encephalopathic neonates to induced hypothermia (33.5°C esophageal) versus usual care for 72 hours. Forty-four percent (45/102) of subjects treated with hypothermia had a poor outcome (death or disability at 18–22 months) compared to 62% (64/106) of patients in the control group (relative risk (RR), 0.72; 95% CI, 0.54–0.95, P = .01). When death was evaluated as an isolated outcome there was no difference between the hypothermic and control groups (P = .08).
A second study evaluated the selective head cooling of neonates with moderate and severe encephalopathy. Severity of brain injury was stratified based on amplitude electroencephalogram (EEG) recordings within 5.5 hours of birth. Neonates were randomized to receive head cooling to a target rectal temperature of 34°C to 35°C for 72 hours or usual care. Fifty-five percent (59/108) of neonates treated with hypothermia had a poor outcome (death or disability at 18 months) compared to 66% (73/110) treated with usual care (P = .1) (61). Interestingly, a subanalysis evaluating only patient’s with moderately abnormal amplitude integrated EEG background patterns showed a decrease in poor outcome for those treated with hypothermia vs usual care (48% vs 66% [OR, 0.47; 95% CI, 0.22–0.8; P =.009]).
Most recently, a third trial of induced hypothermia following neonatal asphyxia randomized 325 infants to hypothermia or usual care. There was no difference in the primary outcome of severe disability or death (62). Despite these mixed findings, induced hypothermia for the treatment of perinatal hypoxic-ischemic encephalopathy is standard of care.
Traumatic Brain Injury
TBI is the leading cause of mortality and severe morbidity in children. According to the Centers for Disease Control and Prevention, approximately 475,000 children under the age of 14 sustain TBI annually (63). Induced hypothermia has been systematically evaluated in the adult TBI population and while initially found to be beneficial in a small single institution study, a large systematic review of induced hypothermia showed no benefit (64,65). For pediatric TBI, three early phase clinical trials showed the implementation of induced hypothermia to be feasible (66) and safe (67,68), but were not powered for efficacy. Subsequently, a randomized controlled trial of induced hypothermia (32°C–33°C) for 24 hours following severe pediatric TBI found hypothermia to be no more effective than normothermia. Subjects were randomly assigned to hypothermia or normothermia within 8 hours of brain injury, cooled for 24 hours and rewarmed by 0.5°C every 2 hours back to normothermia. There was a trend for higher mortality in the cooled group and there was no difference in neurologic outcomes between the normothermia and hypothermia groups. Subjects in the hypothermia group had more hypotension in the rewarming period which may be independently associated with worse outcome following TBI (69). Criticisms of this study were that cooling was initiated too late after initial injury and not maintained through the period of peak swelling (approximately 48 hours), and hypotension was not adequately controlled during the rewarming period.
Adult Stroke
Induced hypothermia for the treatment of adult stroke has been evaluated in multiple small pilot studies and several small randomized controlled trials that were not powered to determine the impact on neurologic mortality and morbidity (70). These trials evaluated the safety and feasibility of inducing and maintaining hypothermia in stroke patients utilizing either an endovascular cooling catheter or external cooling devices (71–74). The cooling for acute ischemic brain damage (COOL AID) trials evaluated the feasibility of induced hypothermia for the treatment of adult ischemic stroke, and while they were able to cool patients safely, there was no significant difference in outcomes between groups (75). Prospective evaluation of hypothermia to minimize neurologic morbidity and mortality after adult ischemic stroke is underway. To date there is no pediatric data evaluating the efficacy of this therapy.
■ CLINICAL IMPLEMENTATION OF TEMPERATURE CONTROL
Implementation of temperature control, whether it be controlled normothermia (goal 36°C–37.8°C) or induced hypothermia (32°C–34°C) requires appropriate equipment, monitoring, trained personnel, and expertise to minimize the side effects of controlled normothermia and induced hypothermia. Both pharmacologic and nonpharmacologic methods of temperature control have been evaluated. Pharmacologic interventions may be effective at preventing fever but have not been effective for inducing hypothermia (30). In children, nonpharmacologic temperature control is most commonly performed with an external cooling blanket (55). Other potential, but less commonly used techniques are bladder irrigation, cold intravenous fluid administration, and gastric lavage. Below we will describe the equipment needed, the available protocols, and the common side effects that must be considered to safely maintain normothermia, induce and maintain hypothermia, and rewarm patients back to normothermia.
Pharmacologic Intervention
Both fever treatment and fever prevention are performed utilizing antipyretic agents such as acetaminophen and nonsteroidal antiinflammatory medications such as ibuprofen. While these drugs have standardly been used for fever prevention and treatment they are not always effective at achieving normothermia following brain injury. Acetaminophen for adults following ischemic stroke may be effective for fever prevention (76). A randomized controlled trial of high dose acetaminophen vs low dose acetaminophen vs placebo following adult ischemic stroke resulted in a 0.4°C decrease in rectal temperature in the high dose group but no difference in the low dose and placebo groups (77). When ibuprofen was compared to these groups, there was no difference in temperature reduction between ibuprofen and placebo (78). Therefore, when temperature control is desired, external devices should be utilized independently or in conjunction with antipyretics.
Nonpharmacologic Intervention
Equipment and Monitoring
To control a patient’s temperature it is necessary to have (1) two core temperature probes and (2) a cooling device (such as an external cooling blanket and machine) (Table 6.2). Core temperature probes are most commonly placed in the rectum, esophagus, or bladder. The esophageal probe is placed directly behind the left atrium in the distal esophagus and measures changes in temperature more rapidly than bladder and rectal probes. One temperature probe, preferably the esophageal probe, should be connected to the cooling machine (primary probe). A second temperature probe should continuously monitor temperature. The two temperature probes should have temperature measurements that are within 1ºC of each other. It is important to have two temperature probes to ensure that the measured temperatures are reliable.
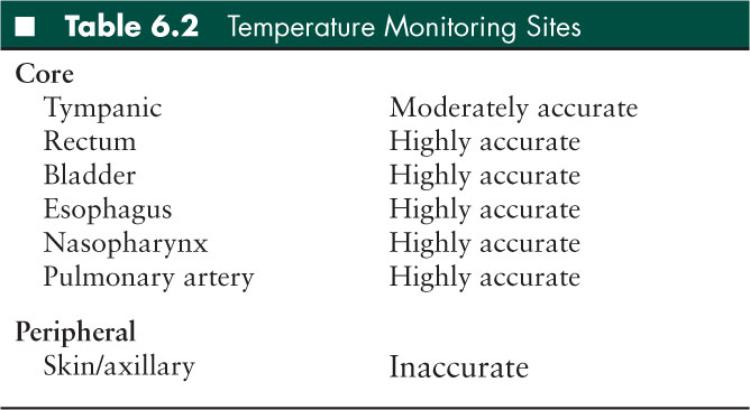
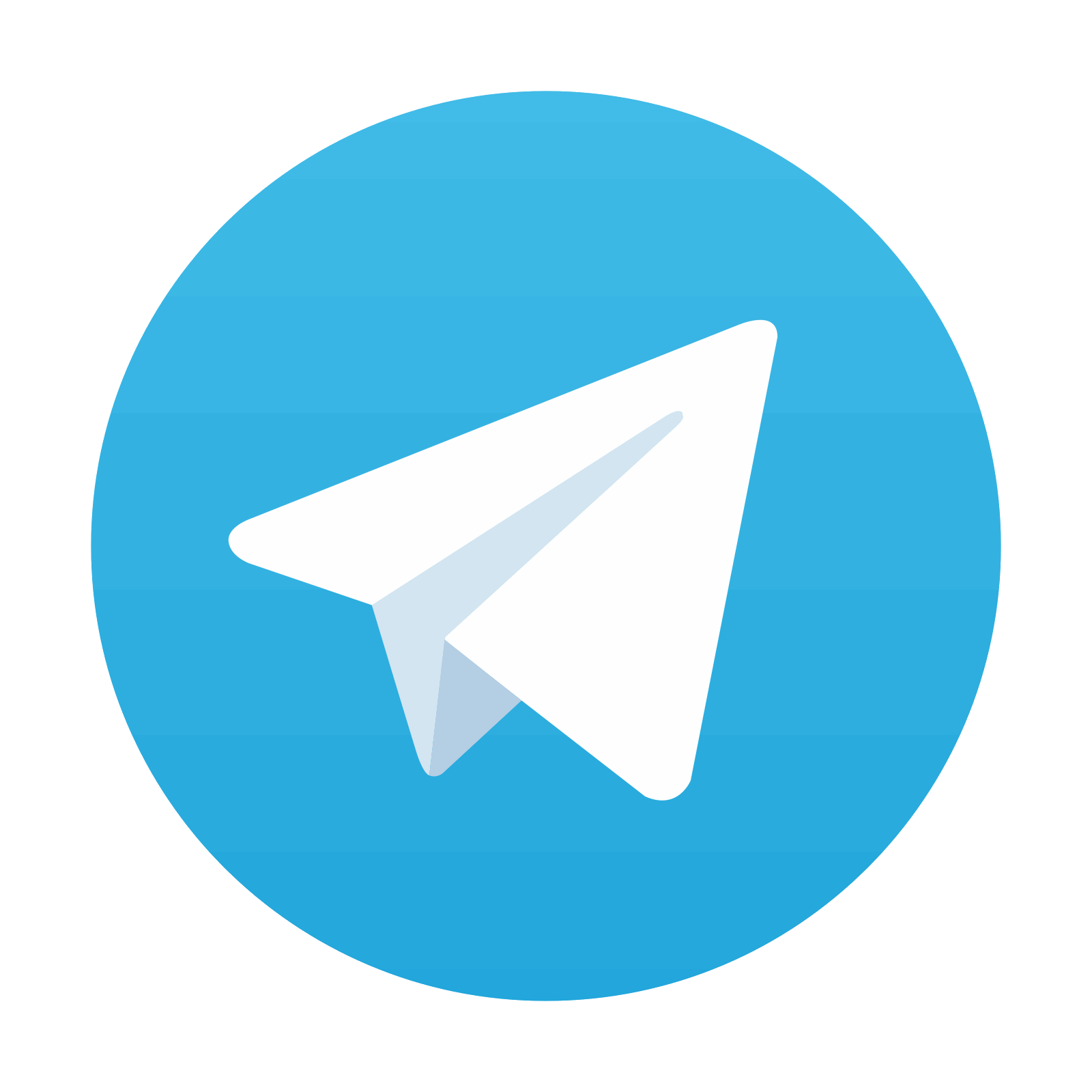
Stay updated, free articles. Join our Telegram channel

Full access? Get Clinical Tree
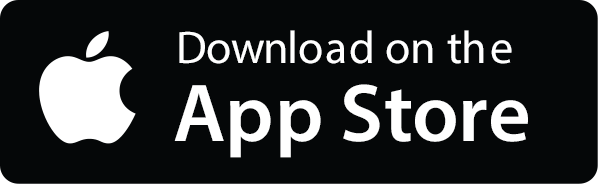
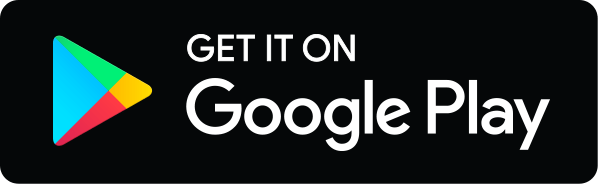