Heat conduction from an electric cooker hob plate;
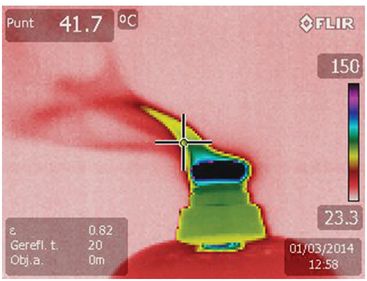
Heat convection from an oil burner.
The Law of Cooling is used by forensic scientists in order to calculate time of death:
A corpse with body temperature = 32 °C is discovered in a room.
Temperature of the room is kept constant at 20 °C.
Three hours later the temperature of the corpse dropped to 27 °C.
The observed temperatures of the corpse can be used to find “k”:
Δt = 3 (hrs)
T0 = 20 (°C)
T1 = 32 (°C)
T2 = 27 (°C)
k = (−1/Δt) ln ((T2−T0)/(T1−T0)) = (−1/3) ln ((27–20)/(32–20)) = 0.1797
Assuming the temperature of a corpse at time of death = 37 °C, and using
k = 0.1797
T0 = 20 (°C)
T1 = 37 (°C)
T2 = 32 (°C)
Δt = (−1/k) ln ((T2−T0)/(T1−T0)) = (−1/0.1797) ln ((32–20)/(37–20)) = 1.94
Time of death = 1.94 hours before the corpse was discovered.
The principles of conduction, convection, and radiation can be applied in order to prevent situations of heat loss, e.g. Fig. 6.2 demonstrates an experiment carried out to investigate the rate of heat loss over a 150 second time period under different holding conditions. Three 30 mm Petri dishes were filled with 2.5 ml of oil, covered with a lid, and equilibrated to 37 °C. The dishes were then removed from the incubator, and a small temperature probe inserted through a 0.5 mm hole in the lid. Readings were taken in three different situations:
1. Dish held with fingers, no contact with palm.
2. Dish left on the bench.
3. Dish held on the palm of the hand: contact with body temperature prevents significant heat loss.
Using these same principles, heat conduction properties of metal can be applied to in vitro culture by using a thermal coin to facilitate dish warming: the metal coin provides direct contact between its heated surface and the bottom of a culture dish (Fig. 6.3). Direct contact with a warm thermal coin helps to prevent temperature losses, and also facilitates more rapid recovery from temperature loss for the culture media in the dish.
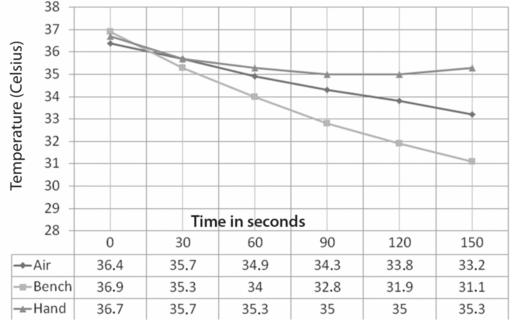
Graph depicting the effect on cooling rate when a 30 mm Petri dish is taken out of an incubator at 37 °C and held at room temperature in three different ways: 1. Dish held with fingers, no contact with palm; 2. Dish left on the bench; 3. Dish held on the palm of the hand: contact with body temperature prevents significant heat loss.
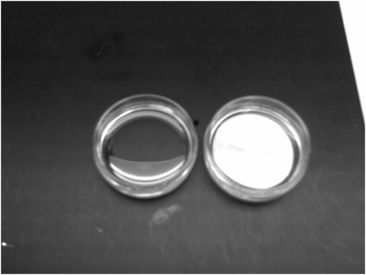
Photograph of a 30 mm Petri dish placed on a bench (left), and with a thermal coin placed underneath (right).
Thermographs that illustrate the effect on thermal equilibrium in 30 mm Petri dishes are shown in Fig. 6.4. The intercalation of the thermal coin allows heat exchange by conduction instead of convection. The dishes reach the same final temperature, but there is a gain of 3 minutes with the use of a thermal coin. This experiment illustrates that the small rim on the bottom of the dishes allows a layer of air to remain between the heating stage and the dish. A thermal coin placed under the dish allows heat to be conducted directly from the heated metal to the dish. The blue line at the border of the dishes indicates that this area is cooler: media droplets placed around the rim of the dish will have a lower temperature.
Two Petri dishes filled with oil at the same temperature were placed on a heating stage set to 37 °C. A preheated Thermocoin (LivingCell) was placed under the dish on the right, and thermographs taken at 3-minute intervals. After 3 minutes (9.03) the dish on the left (a) had lost heat compared with the dish on the right (b). It took a further 3 minutes (9.06) for the dish without Thermocoin to reach the same temperature as the dish with the coin.
The blue circles at the perimeter of both dishes indicate a lower temperature – a factor to be considered when positioning drops in an ICSI dish.
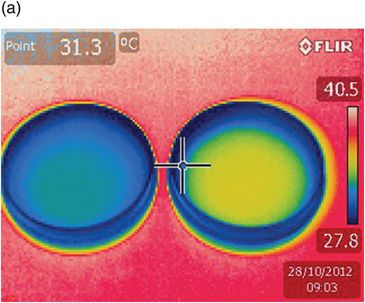
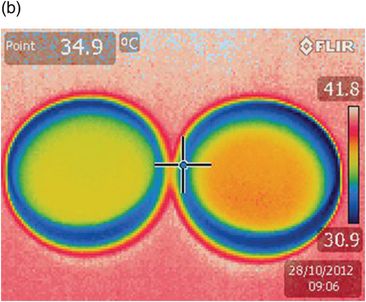
Thermographs must be interpreted with caution, as the colors perceived are subject to artifacts due to reflection of light or incorrect imaging angle: green is not “a priori” good, as demonstrated in Fig. 6.5. Convection due to fluid or gas movements can also cause unequal heat distribution.
Thermographs taken of the same dish, to illustrate artifacts that may influence interpretation of results.
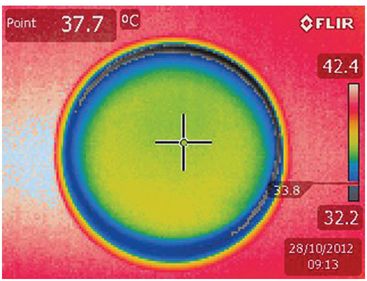
Photo taken at a correct angle.
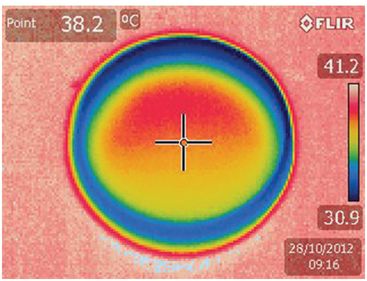
Photo taken with the camera positioned incorrectly; the angle of incidence creates an artifact, so that the upper part of the dish appears to be hotter, when it is not. A similar artifact can be created when light is reflected from a surface that is being measured.
Incubators
A reliable incubator is a crucial component of any IVF laboratory. An optimal culture system is dependent upon an environment that is stable and correct with respect to temperature, air quality, media pH, and osmolality. The IVF incubator has an influence on all of these parameters, and is therefore an integral part of the culture system. All incubators should be protected from power failure via connection to a back-up battery unit and/or generator-protected electrical outlet. Effective incubator management is a crucial cornerstone of quality management, and understanding incubator components and function is paramount in troubleshooting. Modern incubator models, their selection and management have recently been reviewed in great detail (Swain, 2014; Kelly & Cho, 2014), and only salient features relevant to troubleshooting are covered here.
Incubator models specifically designed for IVF culture have a wide range of capacities and features that use different technologies to regulate their internal environment. “Standard” large box-type incubators can accommodate a large number of cases, and independent temperature and gas probes can be placed inside them (see Fig. 8.2, Chapter 8). Many have special features, including HEPA and VOC filters, sterilization cycles, data-logging to monitor temperature, and door opening, as well as new technologies such as time-lapse imaging. Their heating system may involve direct heat (air-jacket, radiant-walled) or air convection (water-jacketed). Air-jacketed incubators recover temperature more quickly after door opening, whereas water-jacketed incubators can maintain temperature for a longer period of time after door opening or in case of a power cut. Box incubators can have the inner door divided into multi-doors (usually three or six).
Smaller benchtop-type incubators heat by direct contact (conduction), and can recover temperature and pH quickly after opening/closing. Numerous studies can be found that compare the features and clinical outcomes associated with the different types of incubators, but ultimately proper management for any type of incubator, ensuring appropriate function and optimal performance, is the most important factor in obtaining clinical success.
Some incubators are designed so that they can be “stacked”, one on top of another, economising on space in small laboratories. However, this may be a “false economy”: shelves that are very low or very high may be physically out of reach for some members of staff, and bending down low or reaching up high for culture dishes incurs the risk that they will be dropped. Incubators that are positioned at benchtop height avoids this risk; automatic bench height controls with dual motors can be fitted that adjust incubator and working height to the individual person: http://www.mynewlab.com/products/laboratory_desks.
Self-contained independent modular airtight chambers (MIC-101, Billups-Rothenberg, Inc., Del Mar, California) are useful as a means of customizing gas mixtures and culture conditions; they can be alcohol sterilized, and can also be convenient for isolating dishes from patients seropositive for blood-borne viruses (see Chapter 9). The chambers are flushed with the appropriate gas mixture and maintained at 37 °C in a routine laboratory incubator or warm room. Each unit has an independent culture environment of desired gas mixture and humidity, without the need for a specialised/gassed “IVF incubator.” Gas ports can be modified to accommodate sensors, and with correct handling, the chambers are less susceptible to fluctuations in temperature and gas than are routine IVF incubators. The chambers can be stacked and interlocked to conserve space and provide stability, and can be a useful back-up as part of a crisis contingency plan (see Chapter 12).
Humidity
“Open” culture, without oil overlay, requires a humidified incubator atmosphere in order to prevent evaporation. This is provided by passive evaporation of water from a reservoir or pan at the bottom of the incubator. Humidity should be maintained at 95–98%. Humidity is not required for oil-overlay culture, provided that the oil has been first appropriately equilibrated (see Chapter 3).
Managing temperature control
The LED display temperature on an incubator, heating block, or heated stage must never be accepted as the actual temperature in a dish or tube. With temperature demand set at 37.0 °C, the thermal mass of media in a culture dish can be considerably lower (Yeung et al., 2004). Establishing an instrument’s LED display temperature that will maintain dishes/fluids at their optimal temperature requires careful calibration, using the appropriate independent device/system to record temperature.
Temperature mapping
A temperature gradient can often be found inside incubators, and the temperature on an upper shelf can differ by 2 °C from that on a lower shelf – the ideal location must be found by recording temperature at several different locations (Higdon et al., 2007; Stoddart et al., 2003; Anifandis, 2013; Walker et al., 2013). All incubators (both box-type and benchtop) should be temperature mapped over a 24-hour period to determine the temperature distribution and confirm that there are no changes with time and under different conditions of use. Constant temperature mapping can be maintained, or mapping can be repeated as part of an annual validation check. Temperature mapping at different times of the year is good practice, in order to cover seasonal variations. Mapping can be carried out by placing thermocouples on every shelf of a box-type incubator, or in each compartment of a benchtop incubator in order to identify:
hotter or cooler areas within the incubator
variation in a single location over a period of time
impact of regular daily interventions (e.g. opening the incubator door).
The results can be stored in portable USB temperature loggers so that the data can be downloaded. The appropriate number of probes used and their locations depend upon the way that the incubator is used. The front and back of the shelf/compartment should be measured, as well as the sites of droplets within each dish type. Figure 6.6 illustrates temperature mapping in order to validate overnight incubator function (a), and as a troubleshooting exercise during oocyte collection into a transport incubator (b).
Incubator temperature mapping.
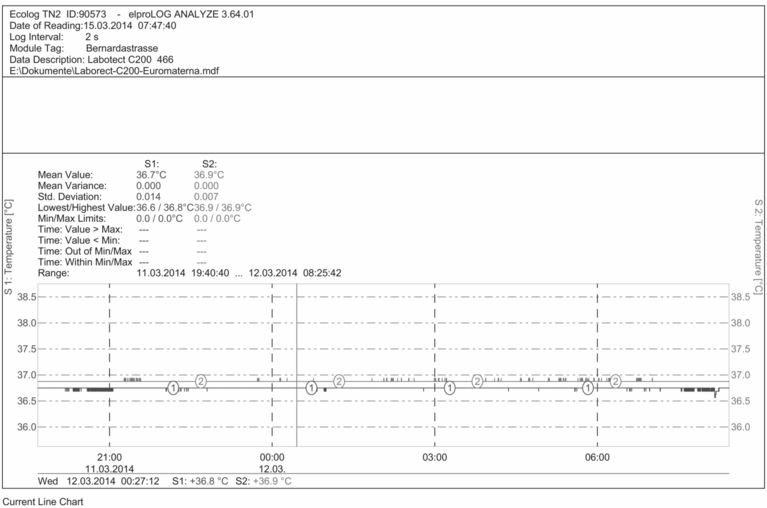
Temperature survey to validate correct overnight incubator function.
PT100 temperature probes were placed on the lower (1) and upper (2) shelves of a 150 l incubator set to 37 °C. A datalogger recorded temperature readings taken every 2 seconds between 8 pm and 9 am the following morning. The temperatures in the two locations differed by 0.2 °C: (1) = 36.6 °C – 36.8 °C; (2) = 36.7 °C – 36.9 °C.
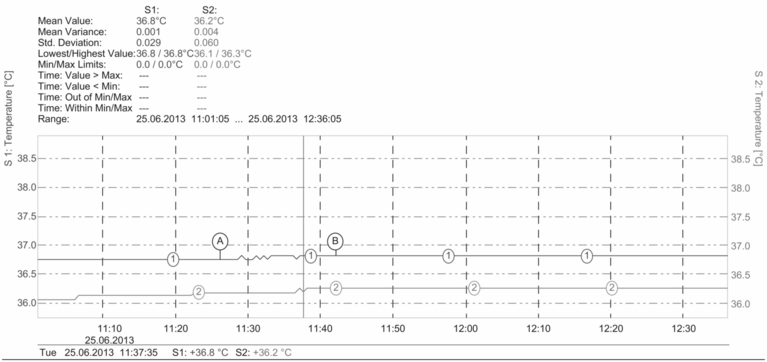
Survey undertaken as part of a troubleshooting exercise. PT100 temperature probes were used to test the function of a transport incubator during follicle aspiration and oocyte collection. The box was opened and closed each time that a tube containing fluid was removed from or placed in the box. 1 = temperature sensor in the metal heating block; 2 = temperature sensor in a cell culture tube with 5 ml medium taken from the 37 °C incubator and placed in the box 30 minutes before starting the oocyte collection. A = start, B = end of the follicle aspiration procedure. There is very little fluctuation in temperature in the heating block and in the tube with medium, but the temperature in the tube with medium was sub-optimal, never rising above 36.3 °C. The transport incubator was re-calibrated and revalidated in order to ensure optimal temperature control.
The degree of acceptable variation must be considered in the data analysis; if necessary, a decision can be made to avoid using some areas of the incubator, labeling these shelves or compartments clearly as “not for use”. Certain models of both box-type and benchtop incubator have been demonstrated to have unacceptable variation in temperature: the manufacturer should be contacted if this is found to be the case. If the problem cannot be corrected, the incubator may need to be decommissioned. Temperature mapping is always advisable when a new incubator is installed, so that any necessary corrective action can be carried out without incurring additional costs.
Temperature mapping may be affected by the incubator’s location within the laboratory, giving results that may differ from those provided by the manufacturer. Air flow within the laboratory should be considered when deciding on the location of an incubator, including air conditioning units, HEPA-filter devices, door openings, etc. (NB: overheating can be just as deleterious to results as underheating – possibly even more so).
Temperature mapping of refrigerators and heated stages/working areas is also good practice. Any variations from the recommended storage temperature in media refrigerators may impact their effectiveness when used at 37.0 °C. Significant variations on microscope heated stages and heated surfaces of laminar flow hoods can be revealed by placing a dish with a thermocouple on different heated areas: the temperature within heated areas in flow hoods is notoriously inconsistent. Microscope stages with an open aperture above the light source may sometimes maintain temperature better than transparent stages that cover the aperture; this can only be revealed by rigorous and considered temperature mapping.
Door opening in large incubators causes immediate heat loss, and should always be kept to a minimum: smaller internal multi-doors are an advantage. Internal doors can be further divided into mini-doors, which allow access to one area of an incubator only, without disturbing other shelves/areas of the incubator. It is useful to label the outside of the external door and the internal doors with the patient’s name, so that the location of a specific dish can be immediately recognized before opening the incubator door. Placing multiple dishes from too many patients into one incubator is not advisable, since constant opening of the incubator door causes fluctuation of temperature/gas, with potentially detrimental impact on other patients’ dishes. A risk assessment should be performed for the number of dishes used per incubator, and used to determine the optimal number of patients treated on a daily basis. Freshly prepared culture dishes and tubes should not be placed on shelves so that they are adjacent to gametes or embryos in culture: introducing cooler plasticware and media will create a temperature gradient that may draw heat away from the pre-warmed dishes.
An IVF lab located in a new clinic in Africa had no air conditioning. The incubator over-heated (LCD registered 40 °C) and “blew”. Fortunately, a second incubator (same model) had been installed, and the embryos were moved to the second incubator. Being reluctant to open the door, which would change the gas levels, staff “fanned” the outside of the overheated incubator in an attempt to bring the temperature down. Engineers were called in to try to fix the problem, but they had never seen an IVF incubator before. They did manage to fix the broken incubator…but as soon as they finished, the second one blew. This continued for 24 hours, with alternating incubators, and movement of embryos between the two. An air conditioning unit was installed the following day.
The main refrigerator for culture media storage in a busy IVF lab had temperature logging, which was checked every morning. On one occasion, the temperature log revealed that the refrigerator temperature had reached 12 °C at 3 o’clock in the morning, with no apparent cause found. Although the refrigerator temperature had corrected itself and returned to 4 °C when staff arrived in the morning, a decision had to be made as to whether the media could be used. The lead embryologist took the decision to throw away the media and order a fresh supply. This involved a significant cost, but the risk of using media that had not been stored correctly was considered to be unacceptable.
Removing dishes from incubators and placing them on a heated stage can involve a significant reduction in temperature, as the heated stage may not be able to maintain the appropriate temperature in the dish. When a dish is removed from the incubator and placed on a heated microscope stage set at 37.0 °C, the thermal mass of the media in the dish can fall to as low as 34.5 °C depending on the size of the dish used and the volumes of media/oil. The time taken to re-gain temperature can be as long as 10 minutes (Cooke et al., 2002; Swain, 2014). The media temperature also varies with the type of culture system used, the volume of media, whether or not a lid is used, etc. Some types of four-well dish have a base that is raised from a heated stage by around 1.5 mm, so that there is no direct heat transfer between the two surfaces; heating the air under a dish in trying to achieve heat transfer can cause a localized temperature increase of up to 41.0 °C. Newer four-well culture dishes are now available to counter this problem, so that the base of the dish is in direct contact with the heated surface, minimizing heat loss.
Oil has a greater heat capacity compared with aqueous media, and using an oil overlay delays the response time in media, dampening the level of fluctuation. When gametes and embryos are manipulated outside the incubator, an oil layer prevents the immediate effects of a decrease in outside temperature from being observed in the media layer; however, heat loss effects are noticeable after the dish is returned to the incubator. Repeated opening of an incubator door has the same effect.
Figure 6.7 plots the temperature rise in a 30 mm Petri dish with 2.5 ml oil overlay at 34.5 °C after being placed into a CO2/O2 incubator: a period of nearly an hour was required before a stable temperature of 36.6 °C was reached. Figure 6.8 demonstrates the effect of using a thermal coin: two dishes with oil at RT (25 °C) were placed into a 37 °C incubator, with and without a thermal coin. The thermal coin curve is 1 °C ahead of the other curve, indicating that the use of the coin decreased the time required to reach a constant temperature plateau.
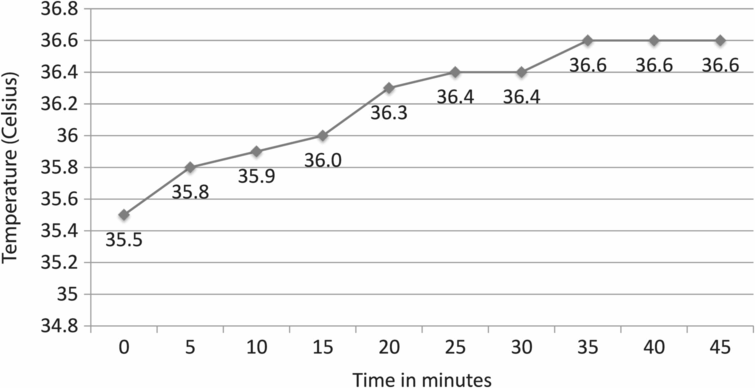
Plot of time in minutes vs. temperature in °C; a 30 mm Petri dish filled with 2 ml light paraffin oil at 34.3 °C placed in an incubator set at 37.0 °C required 55 minutes to reach a stable temperature of 36.6 °C.
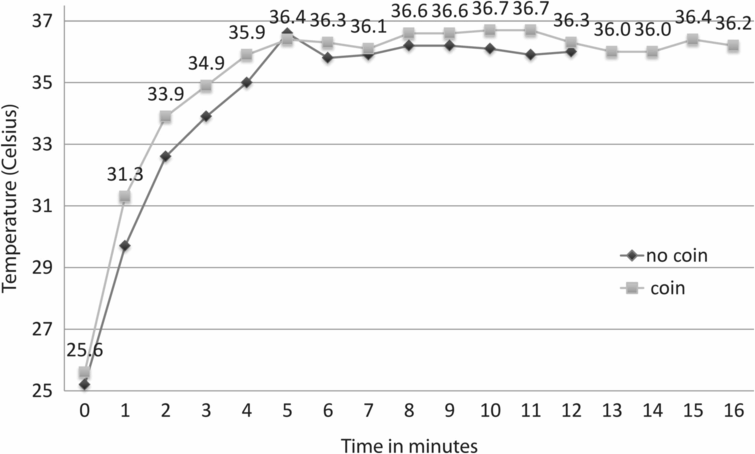
Graph comparing the effect of using a thermal coin on time required for a 30 mm Petri dish filled with 2 ml light paraffin oil to reach 37 °C after being placed in the incubator.
If the demand temperature of the heated stage is increased in order to compensate for heat loss in a particular type of culture dish, it is important to continue using only this type/brand of dish, i.e. the stage demand temperature for a four-well dish will differ from that of round Petri dishes, with the result that samples may be overheated. Similarly the temperature of media droplets in a dish prepared for micromanipulation will differ according to whether the dish base is made from plastic or glass. The real temperature within a media droplet in a dish will be affected by:
Distance between the base of the dish and the heated stage
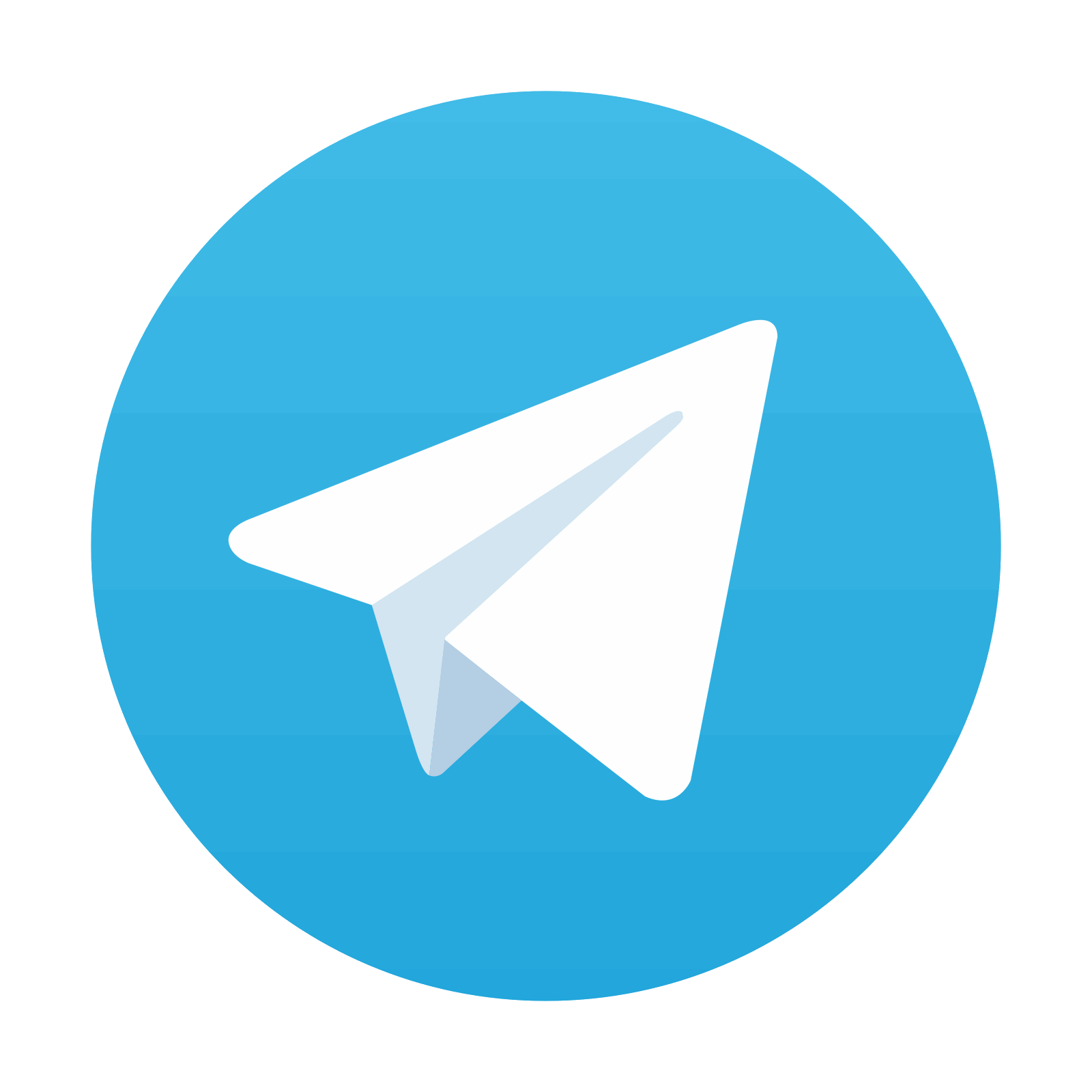
Stay updated, free articles. Join our Telegram channel

Full access? Get Clinical Tree
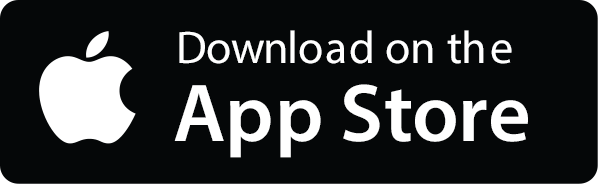
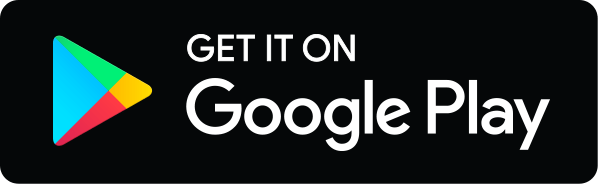