Targeted Therapy and Immunotherapy
COMPONENTS OF THE IMMUNE SYSTEM
While simplistic in its view, the effectors of the immune system are generally divided into those components that support innate immunity and those that support acquired immunity. The classification suggests a dichotomous relationship, but there is necessary and frequent cross-talk between the 2 arms. Innate immunity is active from birth, is the first line of defense against most pathogens, and does not require modification for activity. Acquired immunity largely requires activation of B lymphocytes and T lymphocytes and uses a complex process of activation, modification, expansion, and suppression in response to changing stimuli.
Innate immunity includes physical barriers (skin, mucous membranes), chemical components (hydro-lytic enzymes and complement), and several cellular components. For example, polymorphonuclear lymphocytes are phagocytic cells with lysosomes containing enzymes and generally fight infection. Recent data suggest that neutrophil survival is modulated by T-cell responses, thus illustrating 1 area of cross-communication between the innate and acquired immunity systems. Monocytes are heavily granulated cells that migrate from blood to various tissues and differentiate depending on site. They become Kupffer cells in the liver, microglial cells in the central nervous system, and macrophages in the lung, spleen, and peritoneal surface. Collectively, these differentiated cells (histiocytes and macrophages) become part of the reticuloendothelial system with a major function of phagocytosis of invading entities. The role of the macrophage is much more complex, however, because it produces proinflammatory proteins (cytokines and chemokines) that stimulate the growth of specific immune and inflammatory cells and positions them where needed. They mediate inflammatory responses during wound healing and, after consuming pathogens, can present them to the corresponding T-helper cell. This is another example of communication between traditionally defined innate and acquired immunity effectors. This presentation is done in conjunction with attaching to a major histocompatibility complex (MHC) class II molecule to identify the macrophage as self despite the foreign antigens on its surface. Macrophages can also play a counterproductive role in tumor elimination through the production of molecules that promote tumor growth and angiogenesis (eg, vascular endothelial growth factor [VEGF] and basic fibroblast growth factor [FGF]). Because they promote inflammation, they also release compounds such as tumor necrosis factor (TNF), resulting in nuclear factor-κB activation, which inhibits apoptosis.
Natural killer (NK) cells are granular lymphocytes (10%-15% of total blood lymphocytes) that recognize and destroy tissues that have been altered or stressed, typically by viruses or by malignant transformation. They differ from T lymphocytes in that they do not have antigen-specific receptors. Instead, they have inhibiting receptors that can recognize the MHC class I molecules on normal cells preventing activation. MHC-I expression is absent or aberrant on many virus- and tumor-infected cells. In another example of cooperation between innate and acquired immunity, NK cells participate in the process of antibody-dependent cell-mediated cytotoxicity (ADCC). Immunoglobulin (Ig) G antibodies can bind to infected cells. Fc receptors found on NK cells (also are present on macrophages, neutrophils, and eosinophils) bring the NK cell into contact with the antibody-coated target cell. Upon contact, NK cells release modified cytoplasmic granules containing perforin and granzymes, which promote apoptosis.
The primary effector components of the acquired immune systems are T and B lymphocytes and involve their interaction with antigens. Antigens are any agents that can bind to a component of the immune system, such as binding with an antibody. This is distinct from an immunogen, which also produces an immune response. In general, for an antigen to be immunogenic, it has to be recognized as foreign (ie, tolerance must be broken), has to be large and complex enough (this can sometimes be rectified by using carriers), and for T-cell activation, has to interact with MHC on the antigen-presenting cells. Factors such as dose, schedule, adjuvant therapy, and route of administration have also been shown to have an impact on immunogenicity. A variety of entities may serve as antigens with varying immunogenicity, with common ones including carbohydrates (these can also induce antibody responses without T-cell help), lipids and nucleic acids (both require protein carriers), and proteins.
Biologic Response Modifiers
Cytokines are secreted substances that facilitate a variety of immune response functions. Many are well characterized, and as examples, a few will be discussed here. Classifications are arbitrary because most are pleio-tropic in function. Chemokines are chemoattractant cytokines, which are low molecular weight proteins, that direct the movement of a variety of effector cells to needed locations. Interleukin (IL)-8, for example, which is secreted by macrophages, mobilizes and activates neutrophils and promotes angiogenesis.1 Colony-stimulating factors cause the differentiation of progenitor cells in the bone marrow, with many produced for clinical use (granulocyte colony-stimulating factor, granulocyte-macrophage colony-stimulating factor, erythropoietin, and thrombopoietin).2 Interferons are produced by lymphocytes, macrophages, and dendritic cells and have a wide variety of functions. The interferon-γ produced by TH1 cells activates NK cells and macrophages and induces the expression of MHC class II on many cell types. Many cytokines also promote inflammation such as IL-1, IL-6, IL-23, and TNF-α.3 Despite diverse functionality, cytokines generally bind and induce polymerization of a cell surface receptor, which activates a signal transduction pathway. It is not well understood how 2 cytokines with diverse functions retain specificity when using the same signal transduction pathway.
PRINCIPLES OF IMMUNOTHERAPY
Humoral Immunity
B lymphocytes (B cells) produce secreted antibodies that can recognize soluble and cell surface molecules in response to an antigen. Each B cell expresses immunoglobulin against a single antigenic determinant, with the immunoglobulin expressed at the cell surface of the B cell. The diversity of specificities in different B cells is generated by rearrangements of the immunoglobulin genes, and new antibody specificities continue to be generated in response to new antigens.4 The antigen binds to the B-lymphocyte surface receptor and activates the B cell. B lymphocytes are mobile and migrate to the T-cell rich areas where T-cell help can be provided to promote increased antibody diversity and increased affinity through immunoglobulin gene rearrangements and class switching. Many antigens require costimulation from CD4+ helper T cells (T-cell–dependent antigen), but other antigens do not, including nonprotein antigens such as glycolipids (T-cell–independent antigen). Clonal expansion ensues to make multiple copies of the antigen-specific B cell. Differentiation then occurs to form either plasma cells of short duration (antibody-producing cells), memory cells (which are responsible for the amnestic response), or a small population of B cells expressing germline immunoglobulins that have not undergone rearrangement (which are found in the CD5+ B-cell population). Antibodies are produced by the plasma cells of 1 of 5 isotopes (IgG, IgA, IgM, IgE, or IgD). The immunoglobulin variable region (called the Fv region) determines antibody specificity and is located in the Fab domain of immunoglobulins. A conformational change in the Fc portion occurs after binding of antibody to antigen, leading to the activation of several effector mechanisms including complement activation. The early antibody response is IgM, but if T-cell help is available, antibody responses mature through immunoglobulin gene rearrangements into the higher affinity IgG classes. These IgG molecules are capable of improved binding to antigen as well as receptors on the bone marrow–derived cells through their Fc domain, thereby expanding effector functions. The response to most nonprotein antigens are IgM class and generally do not mature to IgG responses. IgM is pentameric and has increased avidity to bind multimeric antigens. It then activates complement as its main effector function.5 The complement system consists of a variety of blood components with different enzymatic properties, which cause opsonization (coating of pathogens by complement components); recognition by complement receptors on macrophages, monocytes, neutrophils, and dendritic cells; and subsequent activation of these cells, leading to phagocytosis and/or killing. In addition, complement can cause local and direct killing by forming a membrane attack complex that creates holes in membranes of target pathogens and cancer cells, producing complement-dependent cytotoxicity.
IgG antibodies are synthesized following immunoglobulin gene rearrangements, with switches in Fc domains, as the B cell matures in response to T-cell help. IgG antibodies usually have higher affinity than IgM antibodies and can be found in the extracellular space and in the blood. Subclasses of IgG antibodies in humans are especially effective at activating complement and also sensitizing pathogens for killing by NK cells, macrophages, and other cells with complement receptors and immunoglobulin Fc receptors.
The cross-linking of Fc receptors can also lead to activation of the cells and can produce ADCC of tumor cells through the production of cytotoxic molecules such as perforin and granzymes by NK cells. Monoclonal antibodies are commonly used for cancer therapy. Antitumor effects can be in part mediated by antibody binding to critical molecules on the surface of tumor cells, for example by inhibiting tumor cell attachment or growth receptors. However, generally more than 1 mechanism is at work, and Fc receptor–mediated effector mechanisms such as ADCC are also activated.
Cellular Immunity
T lymphocytes arise in the bone marrow and migrate to the thymus, where they differentiate and develop T-cell receptors and CD4 and CD8 coreceptors. The coreceptors bind to the MHC complex (CD4 cells bind to MHC class II molecules, and CD8 cells bind to MHC class I complexes), as shown in Figure 21-1. The MHC binding is required as a first step to ensure the immune attack is directed at the appropriate target and self can be recognized. Antigens must be processed by antigen-presenting cells for recognition (dendritic cells are 1 major example). The combined antigen/MHC molecules are then trafficked to the cell surface for recognition by T-cell receptors, which are encoded by genes of the immunoglobulin family. Just as with antibodies, the diversity of T-cell receptors is generated by rearrangements of these immunoglobulin family genes. Each monoclonal T-cell receptor binds to its appropriate antigen/MHC complex presented on the surface of the antigen-presenting cell. Two signals are required for T-cell activation. The first is the binding of the antigen/MHC complex. The second is a costimulatory signal that comes from the T-cell surface molecule CD28. CD28 engages B7 molecules found on the antigen-presenting cells. Therefore, successful activation of a T cell requires engagement of the T-cell receptor by an appropriately presented antigen/MHC complex in conjunction with CD28 engagement, with B7 as a “safety lock” to be certain activation occurs only in the correct setting.6
FIGURE 21-1. Cells expressing major histocompatibility complex (MHC) class II cells interact with CD4+ T cells, which produce cytokines. Cells expressing MHC class I cells interact with CD8+ T cells, which destroy infected host cells.
Ox40 and 4-1BB are examples of 2 other costimulatory molecules that are upregulated on the surface of activated T cells, which promote survival of T cells and generate T-cell memory responses.7 Multiple effector functions are available to T cells once appropriately activated by professional antigen-presenting cells (primarily dendritic cells), including the production of cytokines and cytotoxic molecules, which lead to death of target cells.
The immune response must be suppressed when the threat has resolved. Natural CD4+/CD25+ T regulatory cells constitute approximately 10% of CD4+ cells and participate in this function. Cytotoxic T-lymphocyte antigen 4 (CTLA-4) is expressed on these cells. CTLA-4 also binds B7 molecules, but with much higher affinity, thus displacing the required CD28 activation signal. CTLA-4 signaling leads to downregulation of the T-cell response. The manipulation of CTLA-4 expression is being investigated as a novel therapeutic maneuver, with recent data supporting its usefulness in treating patients with melanoma.8 Depletion of T regulatory cells may be particularly useful in enhancing the response to tumor vaccines. The multiple opportunities for paired interactions during the T-cell activation process are illustrated in Figure 21-2.
FIGURE 21-2. Selected paired interactions required at the surface of an antigen-presenting cell (APC) and a CD4+ T cell that lead to activation and proliferation with cytokine production. MHC, major histocompatibility complex; TCR, T-cell receptor. (Reproduced, with permission, from Dubinett SM, Lee JM, Sharma S, Mule JJ. Chemokines: can effector cells be redirected to the site of the tumor? Cancer J. 2010;16(4):325-335.)
Immunosurveillance
The notion that the immune system may identify and destroy tumors is long-standing. William B. Coley first observed regression in some patients with sarcoma contracting “accidental erysipelas” as early as 1891. Because the rate of spontaneous regression for tumors is rare and patients with functioning immune systems still develop cancer, the confidence in the ability of the immune system to control cancerous growths has varied over the decades. More sophisticated animal models showing the development of carcinoma in animals deficient in various immunologic components, however, allowed the concept of immunosurveillance to grow. Observations noting CD4+ and CD8+ tumor-infiltrating lymphocytes (TILs) in patients with epithelial ovarian cancer further support its role, as illustrated in Figure 21-3. For example, the 5-year overall survival in epithelial ovarian cancer has been related to the presence or absence of TILs (38% vs. 4.5%, ).9 A second study also showed improved survival in patients with increased frequencies of intraepithelial CD8+ TILs (55 months vs. 26 months; hazard ratio, 0.33; 95% confidence interval [CI], 0.18-0.60;
). In contrast, patients with increased numbers of immune-suppressive CD4+CD25HI regulatory T cells have reduced survival.10 The process is made more complex when we recognize that not only can the immune system protect the host against tumor development, but also some select cancer cells of lower immunogenicity can escape early immunity due to changes in gene expression. This actually leads to an outgrowth of tumors with the capacity to escape recognition, and this process has been termed immunoediting. The immunoediting or “immune sculpting” process, therefore, is responsible for shaping the immunogenicity of the tumors that will eventually form. Considering the effectors of the immune system in the context of both of these processes is important to develop immune-directed therapies with the greatest chance of success.
FIGURE 21-3. Tumor-infiltrating lymphocytes found in epithelial ovarian cancer specimens. (Images contributed by R. Soslow [Memorial Sloan-Kettering Cancer Center, Pathology]).
Escape From Surveillance
An alteration in the function of almost every process discussed herein to facilitate immune activation has been postulated to participate in the ways tumors can evade immune detection. In some cases, antigen presentation is downregulated, or gene deletions or rearrangements may cause reduced expression of the MHC-I complex, thus preventing T-lymphocyte activation. Tumors can also secrete proteins that inhibit T-cell effector action or that promote the development of regulatory T cells that suppress immune function. A recent novel observation showed that certain melanomas can actually remodel their stromal microenvironment so it resembles lymphoid tissue, which recruits regulatory cells to promote tolerance and allow tumor progression.11 Other mechanisms include the down-regulation of intracellular adhesion molecules, changes in molecules responsible for apoptosis signaling, and the development of peripheral tolerance. Based on the increasing number of interacting mechanisms with putative activity that allow immune escape, approaches directed against multiple mechanisms will likely be needed to eradicate immune-tolerant tumor cells.12
Immunoprophylaxis
The development of most immunotherapeutic strategies for investigation requires deciding which of the tumor-associated antigens one will target (often multiple). The next step is to select the strategy that will be used (such as dendritic cell vaccination or autologous cell lysates) to generate the proposed effector cells. Some assessment that the effector cells are indeed directed at the target is then prudent. Finally, the clinical merit of the approach must be assessed.
Ongoing advances using newer technologies including serologic analysis of recombinant cDNA expression libraries (SEREX), robust applications of bioinformatics, and seromic profiling techniques have allowed the further characterization of tumor-associated antigens in multiple tumor types.13,14 There are more than 2000 candidate tumor-associated antigens, and they are generally classified as follows: (1) differentiation antigens, (2) mutational antigens (that are altered forms of proteins), (3) amplification antigens, (4) splice variant antigens, (5) glycolipid antigens, (6) viral antigens, and (7) cancer-testis antigens. Representative examples of each group are provided in Table 21-1.
Table 21-1 Tumor-Associated Antigen Categories With Representative Examples
In addition to selecting the appropriate targets, the next step is to determine the strategy to be used for vaccination. Multiple approaches have been considered, including: a variety of antigens can be given alone or with adjuvant treatment; modified or unmodified tumor cell lysates can be administered (autologous or allogeneic); dendritic cells can be primed with a variety of agents; tumor hybrids with antigen-presenting cells can be made; or DNA alone or in a recombinant fashion can be administered. A variety of cancer vaccine strategies are listed in Table 21-2.
Table 21-2 Cancer Vaccine Strategies
It is important to evaluate the effector cells for the ability to interact with the target. This may be simple and straightforward, or a variety of surrogate approaches may be used to identify whether the biologic end point is being achieved. This may be the most challenging area in immunotherapy because a traditional dose-limiting toxicity finding model does not apply. Radio-immunoassays (enzyme-linked immunosorbent assays [ELISA]) are used to measure antigens, antibodies, or antigen–antibody complexes if appropriate. Antibodies are generally tested for specificity against certain cell surface antigens using fluorescence-activated cell sorting techniques (FACS), or following stimulation with specific antigens in vitro, they can be cultured in the enzyme-linked immunosorbent spot (ELISPOT) assay. Assays for T-cell proliferation and activation are more complex. A cytotoxicity assay (cytotoxic T lymphocytes) measures the ability of cytotoxic T or NK cells to kill radiolabeled target cells (often chromium-51) expressing the appropriate antigen that was initially targeted. The percentage of chromium release can be measured.
Possible effector responses (depending on the strategy selected) are varied and include enhancing phagocytosis, antibody and complement activation, loss of adhesive properties of tumor cells promoted by antibody administration, cytotoxic and helper T lymphocytes, ADCC, activated macrophages, neutrophils, NK/T cells, lymphokine-activated killer cells, and NK cells. More than 1 mechanism is generally at work through direct stimulation or via cross-talk between systems.
CLINICAL PRACTICE IN GYNECOLOGIC ONCOLOGY
Suitability for Immunotherapy
Opportunities to improve the outcome for patients exist by making primary therapy more effective or by exploring the application of “consolidation” or “maintenance” approaches to patients in a complete primary or subsequent remission. One important issue in evaluating immunotherapeutic approaches in ovarian cancer is deciding where in the disease course the novel agent should be evaluated. In general, the minimal disease state is sought, and the remission populations are best suited. The value of treatment in clinical complete remission was first established in acute leukemia, and additional “consolidation” or “maintenance” chemotherapy dramatically improved the outcome for some of these patients. These concepts have not found a place in solid tumor therapy, and the nomenclature remains confusing. Strictly speaking, “consolidation” is best applied to those strategies that are of limited duration, such as a fixed immunization course, and “maintenance” is best used to describe interventions that continue for years (or until progression) such as with trastuzumab. In ovarian cancer, no randomized consolidation study has provided a statistically significant improvement in overall survival, although many attempts have been made. Negative randomized consolidation approaches include both subcutaneous and intraperitoneal interferon-α, high-dose chemotherapy, continued intravenous carboplatin versus whole abdominal radiotherapy (WART), chemotherapy versus observation versus WART, intraperitoneal radioactive phosphorus (phosphorus 32), “non–cross-resistant” chemotherapy in the form of cisplatin and 5-fluorouracil for 3 cycles or topotecan for 4 cycles, the monoclonal antibody oregovomab, which targets CA-125, and the SMART study.15–17 Consolidation strategies have generally been used in the first remission population; investigational strategies in the second and third remission groups have been rare and all likewise negative to date.18 Patients with ovarian cancer in remission are ideal candidates for an immunotherapeutic strategy. Recent data highlight the homogeneity of the second and third remission groups who have a progression-free survival (PFS) interval of less than 12 months so that hints of efficacy from a given immunotherapeutic approach could be recognized with a shorter follow-up interval than that required in first remission.
The number of therapeutic strategies under investigation for immunotherapy in patients with ovarian cancer is large. Most trials are pilot studies or phase 1 trials with the goal of assessing safety and immunogenicity. Some have correlated improved outcome with a surrogate such as antibody or T-cell response, and most current trials aim to produce cellular responses. The number of adequately powered randomized trials is few, however, and none has shown definitive efficacy to date. We will consider several examples of immuno-therapeutic approaches that are being evaluated in the clinics, but the list is not exhaustive, and many other approaches have merit.
Antibodies Used as Immunogens
Although some antibodies are administered in the treatment of patients with cancer to convey passive immunity, they may also be used as immunogens and can elicit a complex immune response. Oregovomab (MAb B43.13), which is an IgG1k subclass murine monoclonal antibody that binds with high affinity (1.16 × 1010/M) to circulating CA-125, has been evaluated. Both cellular and humoral immune responses have been seen with the production of anti-oregovomab antibodies (Ab2), T-helper cells, and cytotoxic T cells in addition to the human anti-mouse antibody (HAMA) response. Nonrandomized clinical studies to date have consistently associated a longer overall survival with immune response. A randomized placebo-controlled trial in patients with stage III or IV epithelial ovarian cancer in first clinical remission receiving oregovomab or placebo showed no benefit using the intent-to-treat population. However, a favorable subgroup of patients (≤2 cm residual at debulking, U/mL before third cycle, and
U/mL at entry) showed a time to progression advantage favoring vaccination of 24 months versus 10.8 months (hazard ratio, 0.543; 95% CI, 0.287-1.025). This subgroup was appropriately considered to be hypothesis generating, and a follow-up study enrolled 354 patients using the characteristics of this group as eligibility criteria. The median time to progression was 10.3 months (95% CI, 9.7-13.0 months) for the oregovomab group and 12.9 months (95% CI, 10.1-17.4 months) for the placebo group
, showing no benefit to oregovomab immunotherapy.17
Another antibody strategy is immunization with an anti-idiotype vaccine. The hypothesis is that the antigenicity of the immunogen (in this case, the antibody) can be increased by presenting the desired epitope to the now tolerant host in a different molecular environment. The “immune network hypothesis,” which provided the foundation for this approach, was first proposed in the early 1970s and describes an interconnected group of idiotypes expressed by antibodies. The proposed mechanism assumes that immunization with a given antigen will generate the production of antibodies against this antigen (termed Ab1). Ab1 can generate anti-idiotype antibodies against Ab1, classified as Ab2. Some of the anti-idiotypic antibodies (Ab2β) express the internal image of the antigen recognized by the Ab1 antibody and can be used as surrogate antigens. Immunization with Ab2β (the anti-idiotype antibody) can cause the production of anti-anti-idiotype antibodies (classified as Ab3) that recognize the corresponding original antigen identified by Ab1. Ab3 antibodies are also denoted Ab1’ to show that they may differ in their other epitopes compared with Ab1. The relationships of these antibodies to each other are illustrated in Figure 21-4. A previous phase ½ study of abagovomab, the anti-idiotype monoclonal antibody whose epitope mirrors CA-125, suggested that Ab3 production was associated with overall survival. Other studies have shown an increase in interferon-γ expression of CA-125–specific CD8+ T cells following immunization, but there has been no specific correlation between the induction of Ab3 and frequencies of CA-125–specific cytotoxic T lymphocytes and T-helper cells. The efficacy of abagovomab in patients in first remission is currently being evaluated in an international phase 3, randomized, double-blind, placebo-controlled study ongoing in approximately 120 study locations (MIMOSA Trial). Outcomes are recurrence-free survival, overall survival, and safety. Preliminary blinded immunogenicity results were reported with 888 patients enrolled onto the study and showed that 68% and 69% of all patients were positive for Ab3 (median values, 62,000 ng/mL and 337,000 ng/mL, respectively), whereas 53% and 63% of patients were positive for HAMA (median values, 510 ng/mL and 644 ng/mL, respectively). Efficacy results will be available in early 2011.19
FIGURE 21-4. The relationship of antibodies in an anti-idiotypic vaccine strategy. A. The injection of a tumor-associated antigen (TAA) binding antibody (Ab1) leads to an immune response containing antibodies (Ab2) mimicking the structure of the original antigen. B. Vaccination with a TAA-mimicking antibody (Ab2) leads to an immune response directed to the original antigen.
Cancer-Testis Antigen Vaccines
The cancer-testis antigens are a distinct class of differentiation antigens. The family has grown from the original melanoma-associated antigen 1 (MAGE-1) identified in a melanoma cell line to 100 cancer-testis genes or gene families identified in a recent database established by the Ludwig Institute for Cancer Research.20 These antigens share several characteristics, including preferential expression in normal tissues on the testis and expression in tumors of varying histology (including ovarian cancer), and many are members of multigene families that are mostly encoded on chromosome X. Cancer-testis antigen expression has been correlated with clinical and pathologic parameters in a variety of tumors. MAGE-A4 expression shows an inverse correlation between expression and patient survival, for example, in ovarian cancer .
The NY-ESO-1 antigen, initially defined by SEREX in esophageal cancer, is expressed in several tumors, including 40% of epithelial ovarian cancers. NY-ESO-1 MHC class I and II restricted epitopes (recognized by CD8+ cytotoxic and CD4+ helper T cells) have been characterized, including those recognized in conjunction with human leukocyte antigen (HLA)-A2 as well as with other haplotypes. Both NY-ESO-1 peptides and full recombinant protein have been administered to patients on protocols with immunogenicity as the primary end point with various adjuvants. Vaccination has been shown to induce both humoral and T-cell responses.21 In a phase 1 trial in patients with epithelial ovarian cancer in first remission immunized with HLA-A*0201–restricted NY-ESO-1b peptide with montanide ISA-51 as the adjuvant,22 treatment was well tolerated. Seven (77%) of 9 patients showed T-cell immunity by tetramer and ELISPOT analyses. Multiple approaches have been used to try and enhance the inherently limited immunogenicity of these peptide vaccinations. Some have included amino acid substitution at the anchor positions of Melan-A/MART-126-352L; terminal modification of MART-127-35; substitution of cysteine residues for NY-ESO-1; modification of T-cell receptor interacting amino acid residues for carcinoembryonic antigen; and loading of peptides onto autologous dendritic cells. In addition, cytokines and costimulatory molecules have been administered.
Dendritic Cell–Based Vaccines
Dendritic cells are professional antigen-presenting cells. They endocytose, process, and then present tumor antigens to T cells. Many strategies are currently under way to manipulate the dendritic cell for use in immunotherapy. Dendritic cells have been pulsed with tumor-associated peptides or proteins and mRNA-encoded receptors such as folate receptor-α.23 Other vaccines have been developed by the viral transduction of dendritic cells with tumor-specific genes or through transfection with liposomal DNA or RNA. Another strategy that has been tried to avoid the need to specifically define the effective tumor-associated antigens is to pulse them with tumor lysates or tumor protein extracts. In many cases, preclinical models have suggested protective immunity to subsequent tumor challenge, which supports further interest in investigating the approach.
A specific example includes a study by Czerniecki et al,24 in which advanced breast and ovarian cancer patients were treated with dendritic cells pulsed with HER-2/neu or MUC-1–derived peptides. In 50% of patients, peptide-specific cytotoxic T-cell lymphocytes were generated. Side effects were minimal. Gong and colleagues25 fused human ovarian cells to human dendritic cells and likewise showed the proliferation of autologous T cells, including cytotoxic T-cell activity with lysis of autologous tumor cells by an MHC class I restricted mechanism (ie, demonstrating that the effector cells had the desired activity). Heat shock proteins, which are molecular chaperones that facilitate protein folding, have also been isolated, along with accompanying peptides, and used as immunogens. Heat shock peptide complexes have been shown to interact with dendritic cells via the CD91 receptor. The heat shock proteins are taken up by endocytosis, are cross-presented by MHC-I molecules on the dendritic cells, and result in activation of naïve CD8+ cells along with upregulation of costimulatory molecules and the production of cytokines.
Many reported studies have similar immunologic end points, but the clinical interpretation is often difficult from phase 1/2 trials without comparators. Further issues under study include the choice of dendritic cell subtype and maturational status at immunization, antigen loading method, preparation of the cells loaded on the dendritic cells, and route and frequency of administration.
Vaccines Designed to Generate Antibody Responses
Most current vaccines seek to generate cellular responses (often with an accompanying humoral response), but a vaccine is currently in a phase 2 randomized trial in ovarian cancer (Gynecology Oncology Group [GOG] Study 255) that evaluates a vaccine approach primarily designed to augment antibodies. Techniques for the chemical and enzymatic synthesis of carbohydrate and glycopeptide antigens have permitted the development of a variety of synthetic vaccines that depend on antibody production and ADCC as the primary effectors. A variety of options such as different adjuvant therapies, schedules, and methods of conjugation have been tried to enhance immunogenicity. A proposed optimal construct has consisted of an antigen (single or multiple) with the carrier protein keyhole limpet hemocyanin (KLH) and the saponin adjuvant QS-21 (or OPT-821).26 GOG 255 is a randomized trial in patients with second or third complete clinical remission receiving either the adjuvant therapy alone (OPT-821) or a multivalent antigen construct plus OPT-821. The end point is the proportion of patients disease free at 12 months, and accrual is ongoing.
Adoptive Cellular Therapy
Using the adoptive cellular therapy approach, one selects and activates large numbers of lymphocytes and introduces them into a manipulated host environment with a selected target. One way T cells may be modified to recognize tumor-associated antigens is to introduce ex vivo a gene encoding artificial T-cell receptors termed chimeric antigen receptors (CARs) against a specific tumor-associated antigen. The first phase 1 study in patients with epithelial ovarian cancer using gene-modified autologous T cells with reactivity against the ovarian cancer–associated antigen α-folate receptor (FR) has been reported.27 Cohort 1 received T cells with IL-2, and cohort 2 received dual specific T cells followed by allogeneic peripheral blood mononuclear cells. No reduction in tumor burden was seen in any patient. Polymerase chain reaction analysis showed that gene-modified T cells were present in the circulation 2 days after transfer but then declined. An inhibitory factor developed in the serum of 3 of 6 patients tested over the period of treatment that significantly reduced the ability of gene-modified T cells to respond against FR-positive tumor cells. Future studies need to use strategies to increase T-cell persistence. The chimeric receptor approach continues to evolve in specificity against targets expressed in ovarian cancer such as the LeY carbohydrate antigen (expressed on 70% of ovarian cancer cells) or HER-2/neu. Most recently, receptors have been engineered to target the extracellular domain (termed MUC-CD) of MUC16 (CA-125), which is expressed in most ovarian carcinoma.28 In vitro, these CAR-modified, MUC-CD–targeted T cells showed MUC-CD–specific cytolytic activity against ovarian cell lines, and infusion into severe combined immunodeficiency (SCID)-beige mice bearing orthotopic human MUC-CD–positive ovarian carcinoma tumors showed delayed disease progression or eradication. Clinical trials are planned. One necessary challenge to overcome is how to circumvent the multiple mechanisms in the tumor microenvironment that inhibit tumor-targeted T cells. Options under investigation include administering T cells after lymphodepleting chemotherapy, antibody-based blockade of inhibitory ligands, and infusion of proinflammatory cytokines such as IL-12.
Whole Tumor Antigen Vaccines
This strategy seeks to overcome some of the potential problems associated with trying to generate specific immune responses. In the latter case, the response may simply miss the target, it can be limited to only the epitopes provided on the stimulating antigen and actually drive variants of tumor cells that can evade the immune response (immunoediting), or it may be restricted to small numbers of patients of a certain HLA type, as in the case of using HLA-restricted peptides.22 The reason for using whole tumor antigen vaccines is that it allows one to immunize without needing to define the tumor-associated antigens. They can be derived from autologous tumor cells or using an allogeneic strategy. One obvious challenge in using whole tumor antigen vaccines is that the tumor is currently residing in a host where tolerance to the tumor is already present. This tolerance is likely produced in multiple ways, including the production of IL-10 and transforming growth factor (TGF)-β to inhibit T-cell and dendritic cell functions, VEGF to inhibit dendritic cell maturation and differentiation, and soluble Fas ligand, which induces lymphocyte apoptosis. The whole tumor immunogen, therefore, is processed or modified in some way in an attempt to overcome this. Strategies have included using apoptotic whole tumor cells (developed with a lethal dose of irradiation), using necrotic tumor cell lysates (created with repetitive freezing and thawing and often administered as pulsed dendritic cells), and constructing dendritic cell/tumor fusion vaccines.29 The issue of how to increase the immunogenicity of whole tumor vaccines remains a priority. One effective approach has been the use of a replication-deficient herpes simplex virus to infect tumor cells, which are subsequently engulfed and show enhanced ability to both activate NK cells and provide a costimulatory signal for T cells.
TARGETED THERAPIES
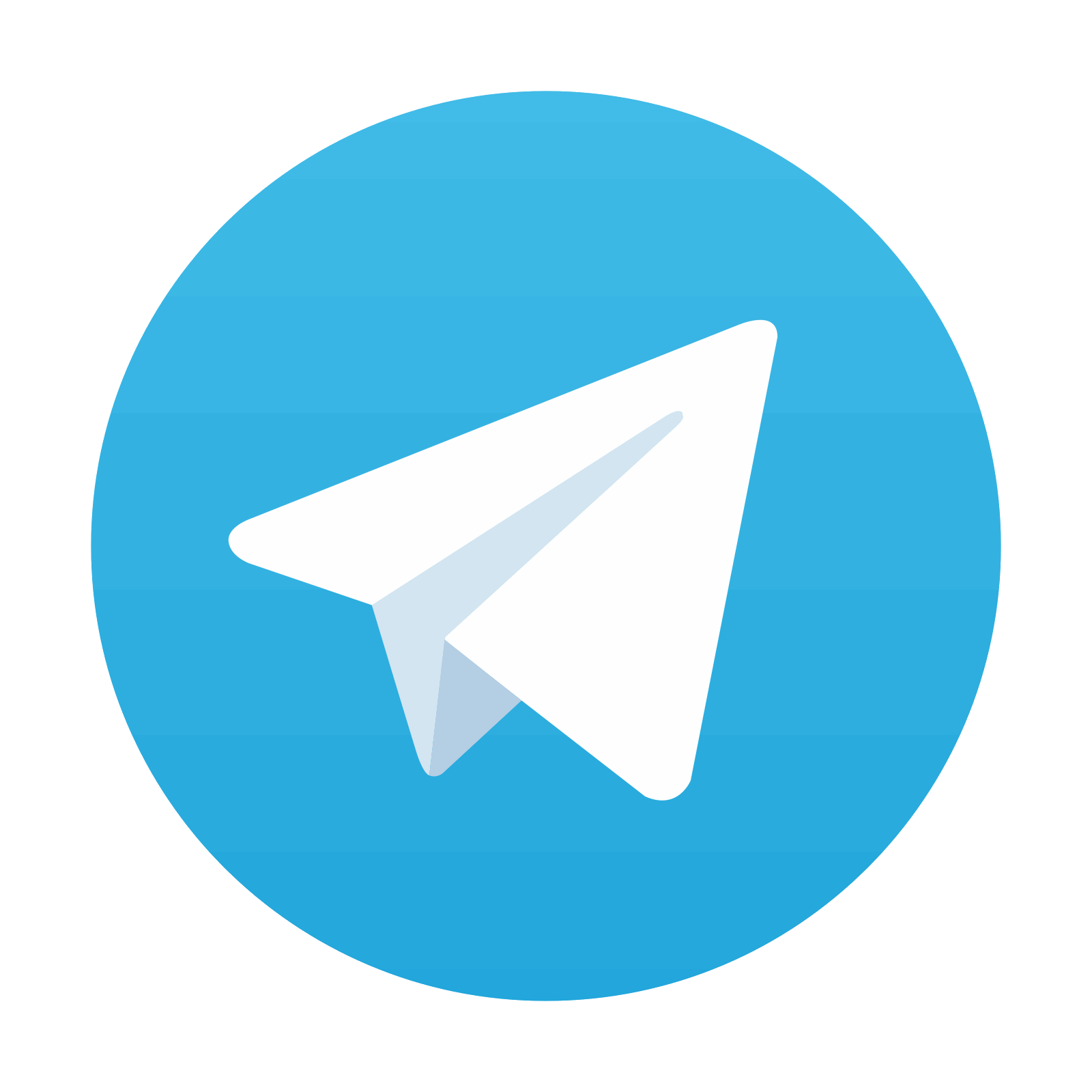
Stay updated, free articles. Join our Telegram channel

Full access? Get Clinical Tree
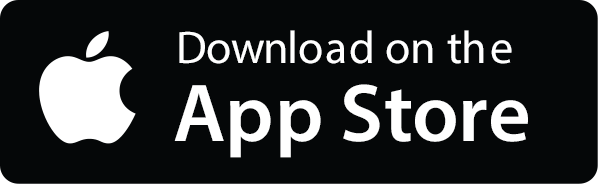
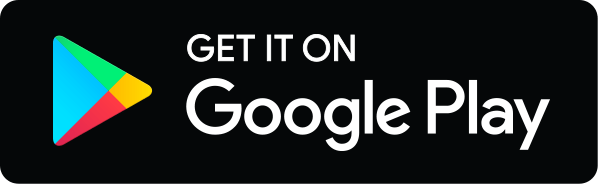