16.2.2 National Comprehensive Cancer Network Survivorship Guidelines
On January 31, 1995, this national alliance was created to develop and institute standards of care for the treatment of cancer and perform outcomes research. With 13 original NCCN Member Institutions, the goal was to ensure delivery of high-quality, cost-effective services to people with cancer across the country. NCCN became a developer and promoter of national programs to facilitate the fulfillment of NCCN Member Institution missions in education, research, and patient care. Now an alliance of 25 of the world’s leading cancer centers, NCCN develops and communicates scientific, evaluative information to better inform the decision-making process between patients and physicians, ultimately improving patient outcomes. The NCCN Survivorship guidelines were originally released in 2013 and included eight distinct areas: anxiety and depression, cognitive function, exercise, fatigue, immunizations and infections, pain, sexual function, and sleep disorders. A new updated version was published in 2014. These guidelines, however, are not specifically intended to provide guidance for the care of survivors of childhood cancers and refer providers to the Children’s Oncology group survivorship guidelines, as well as the NCCN guidelines for Adolescent and Young Adults available at www.NCCN.org.
16.2.3 American Society of Clinical Oncology
The American Society of Clinical Oncology published their guide to survivorship care in 2014 entitled Providing High Quality Survivorship Care in Practice: An ASCO guide. It further detailed high-quality survivorship care to include the following:
1.
Surveillance for recurrence
2.
Monitoring for and managing psychosocial and medical late effects
3.
Providing screening recommendations for second cancers
4.
Providing health education to survivors regarding their diagnoses, treatment exposures, and potential late and long-term effects
5.
Providing referrals to specialists and resources as indicated
6.
Familial genetic risk assessment (as appropriate)
7.
Guidance about diet, exercise, and health promotion activities
8.
Providing resources to assist with financial and insurance issues
This guide discusses how to build a survivorship care program for clinicians including models of care, implementation of a survivorship program, providing care, and measuring the quality of survivorship care. It also includes resources for transitioning care of survivors and working with primary care providers to continue high-quality health care addressing the unique needs of cancer survivors.
16.3 Treatment-Related Late Effects
16.3.1 Cardiac Toxicity
Cardiac toxicity during or following treatment for pediatric bone tumors is largely due to the inclusion of the anthracycline, doxorubicin, given at relatively high doses. Most treatment regimens for bone sarcomas require a minimum cumulative doxorubicin dose of 375 mg/m2, with some as high as 600 mg/m2. The pathophysiology of doxorubicin-induced cardiac damage is incompletely understood, but one important mechanism is thought to be cardiomyocyte death due to the production of free radicals and oxidative stress (Janeway and Grier 2010). Clinically apparent doxorubicin-associated cardiotoxicity presenting as congestive heart failure has been classified as acute, chronic, and late based on the timing of onset related to the doxorubicin administration (Janeway and Grier 2010). Acute cardiotoxicity, occurring after a single dose or course of doxorubicin is rare, particularly with modern chemotherapy regimens. Chronic doxorubicin-associated cardiotoxicity occurs within weeks to months of completing therapy. There are rare reports of late cardiotoxicity in which clinically apparent doxorubicin-induced heart failure occurs more than 1 year after the completion of therapy, particularly in association with periods of increased cardiac growth and demand such as puberty and pregnancy (Janeway and Grier 2010).
Data from the Childhood Cancer Survivor Study (CCSS) demonstrate that survivors of osteosarcoma have been reported to have a cumulative incidence of 10 % at 20 years for acute congestive heart failure for those patients who received >300 mg/m2 (Nagarajan et al. 2011). The Rizzoli Institute has reported the study with the longest follow-up and most complete reporting of adverse events in patients with osteosarcoma. This study reported a 4 % incidence of severe doxorubicin-induced cardiotoxicity (Oeffinger et al. 2006). Cardiotoxicity was noted 1–12 weeks after the completion of therapy, with the exception of one patient who developed cardiac toxicity during the last trimester of pregnancy 8 years after completing treatment (Oeffinger et al. 2006). The Italian Sarcoma Group also reported their experience in patients treated for osteosarcoma from 1983 to 2006 with a reported incidence of cardiomyopathy of 2 % (Longhi et al. 2012). The median interval from the cessation of chemotherapy to the onset of cardiomyopathy was 2 months with a median total dose of doxorubicin of 480 mg/m2 (Longhi et al. 2012).
Survivors of Ewing sarcoma reported in the CCSS were more likely than siblings to have arrhythmias (7.4 % vs. 2.9 %) and other serious cardiac conditions (4.5 % vs. 0.5 %) (Ginsberg et al. 2010). After adjusting for age, sex, and race/ethnicity, the relative risk of a survivor having an arrhythmia or serious cardiac event 5 or more years after diagnosis is 2.3 (95 % CI = 1.4–3.9) and 7.5 (95 % CI = 3.1–18.7), respectively (Ginsberg et al. 2010). The Scandinavian Sarcoma Study Group also demonstrated an increased incidence in heart disease and hypertension in Ewing sarcoma survivors compared to age- and gender-matched individuals from the general population, reporting an odds ratio for heart disease of 7.9 (95 % CI = 2.5–25.3; p = 0.001) (Aksnes et al. 2009). They described two patients treated before the age of 4 who developed cardiotoxicity, and concluded that age of exposure along with cumulative dose were independent risk factors (Aksnes et al. 2009). In the Italian Sarcoma Group report, the cardiotoxicity incidence was 1.3 %, with a median interval from the cessation of chemotherapy to the onset of cardiomyopathy of 3 months (Longhi et al. 2012). The median doxorubicin dose was 400 mg/m2.
Cumulative dose is the most important factor affecting the risk of doxorubicin-associated cardiotoxicity, with a significant increase in the incidence of heart failure occurring after the administration of 550 mg/m2 (Janeway and Grier 2010). Additional risk factors for doxorubicin-induced cardiotoxicity include older (greater than 40) and very young (less than 4 years) age, and female sex (Chrischilles et al. 2014). The number of patients who have doxorubicin-associated heart failure is much lower than the number of patients who develop echocardiographic abnormalities after treatment with doxorubicin.
Dexrazoxane is a topoisomerase 2 inhibitor that scavenges free radicals and chelates heavy metals. It is thought to protect the heart from doxorubicin-induced cardiotoxicity by forming complexes with iron, preventing both tissue damage and the formation of free radicals (Janeway and Grier 2010). Two randomized controlled studies in children show that dexrazoxane decreases the risk of short-term, subclinical cardiac toxicity without increasing infectious complications (Wexler et al. 1996; Moghrabi et al. 2007). Additional studies are required to determine whether dexrazoxane decreases the risk of late cardiotoxicity. A recent study reported an increased incidence of secondary malignancies in patients with Hodgkin’s disease who were randomized to receive dexrazoxane, but concerns have been raised about the methodology of the study (Hellmann 2007; Lipshultz et al. 2007; Tebbi et al. 2007). A trial in which children with leukemia were randomized to receive dexrazoxane did not show an increase in secondary leukemias in patients who received dexrazoxane (Barry et al. 2008). Dexrazoxane has not been systematically assessed in any bone sarcoma studies, so it is not possible at this time to make an evidence-based guideline for or against its use.
The Children’s Oncology Group long-term follow-up guidelines recommend detailed history of shortness of breath, orthopnea, chest pain, palpitations, or if under 25 years abdominal symptoms including nausea or vomiting. Physical exam should include assessment of cardiac murmur, S3, S4, increased P2 sound, pericardial rub, rales, wheezes, jugular venous distension, and peripheral edema. Echocardiogram at entry into long-term follow-up is recommended, and then periodically based on age at treatment, radiation dose, and cumulative anthracycline dose. EKG is recommended at entry into long-term follow-up, repeat as clinically indicated (COG 2013) (Table 16.2).
Table 16.2a
Recommended frequency of echocardiogram (or comparable cardiac imaging) following anthracyclines
Age at treatment | Radiation with potential impact to the heart | Anthracycline dose | Recommended frequency |
---|---|---|---|
<1 year old | Yes | Any | Every year |
No | <200 mg/m2 | Every 2 years | |
≥200 mg/m2 | Every year | ||
1–4 years old | Yes | Any | Every year |
No | <100 mg/m2 | Every 5 years | |
≥100 mg/m2 to <300 mg/m2 | Every 2 years | ||
≥300 mg/m2 | Every year | ||
≥5 years old | Yes | <300 mg/m2 | Every 2 years |
≥300 mg/m2 | Every year | ||
No | <200 mg/m2 | Every 5 years | |
≥200 mg/m2 to <300 mg/m2 | Every 2 years | ||
≥300 mg/m2 | Every year | ||
Any age with decrease in serial function | Every year |
Table 16.2b
Recommended frequency of echocardiogram (or comparable cardiac imaging) following radiation
Age at treatment | Radiation dose | Anthracycline dose | Recommended frequency |
---|---|---|---|
<5 years old | Any | None | Every 2 years |
Any | Every year | ||
≥5 years old | <30 Gy | None | Every 5 years |
≥30 Gy | None | Every 2 years | |
Any | <300 mg/m2 | Every 2 years | |
≥300 mg/m2 | Every year | ||
Any age with decrease in serial function | Every year |
16.3.2 Renal Toxicity
The treatment-related nephrotoxicity for bone tumor patients is primarily due to the chemotherapeutic agents, namely, cisplatin, methotrexate, and ifosfamide. Cisplatin and methotrexate have been a standard of care in the treatment of osteosarcoma since the 1970s, and remaining two of the three drug combination routinely utilized for localized osteosarcoma. Ifosfamide was introduced in first-line therapy of childhood soft tissue sarcomas and bone sarcomas since the 1980s and continues to be used for bone sarcomas today.
The nephrotoxicity of cisplatin is considered dose-related and includes a variable reduction of glomerular filtration rate (GFR) along with tubular dysfunction. Cisplatin directly damages the tubular epithelial cells, resulting in a pathology resembling acute tubular necrosis that leads to increased magnesium loss in the urine expressed as increased renal magnesium (Mg) excretion and hypomagnesaemia (Janeway and Grier 2010). Cisplatin-induced hypokalemia and hypocalcemia are the result of both altered renal processing in the presence of hypomagnesaemia and increased kidney losses. In 12–20 % of patients, cisplatin-associated hypomagnesaemia persists after the completion of chemotherapy (Stohr et al. 2007). About 60 % of children receiving median cisplatin doses of 500–600 mg/m2 have decreased glomerular function at the completion of therapy (Skinner et al. 1998). However, these deficits in glomerular filtration seem to be mild, and improvement is common. In two long-term follow-up studies of osteosarcoma survivors receiving ifosfamide in addition to standard therapy with methotrexate/adriamycin/cisplatin therapy, the incidence of glomerular impairment was low and the extent of dysfunction was minimal (Koch Nogueira et al. 1998; Arndt et al. 1999). The risk of cisplatin-associated nephrotoxicity is associated with higher dose rates and greater dose intensity. Consequently, prolonged infusions and fractionated doses administered over several days have been recommended (Launay-Vacher et al. 2008).
Methotrexate and its metabolites can precipitate in the renal tubules causing acute renal insufficiency and failure (Janeway and Grier 2010). Early studies of high-dose methotrexate done in the 1970 demonstrated that nephrotoxicity was common, and the mortality rate following high-dose methotrexate was as high as 6 % (Von Hoff et al. 1977). The use of aggressive hydration and alkalization of urine as well as the use of leucovorin rescue along with routine monitoring of methotrexate levels has decreased the incidence of nephrotoxicity following high-dose methotrexate to 1–8 % (Janeway and Grier 2010). Unfortunately, severe and fatal cases of high-dose methotrexate-associated nephrotoxcity still occur in patients with osteosarcoma (Widemann et al. 2004). Methotrexate-induced nephrotoxicity is typically an acute treatment side effect and rarely causes long-term renal damage as a single agent. However, it may contribute to the cumulative effects of other nephrotoxic agents.
Many studies have demonstrated that tubular toxicity develops as a result of treatment with ifosfamide. Both glomerular and tubular function may be affected by ifosfamide, and a variety of tubular disorders, such as Fanconi syndrome and hypophosphatemic rickets, have been described. The combination of cisplatin and ifosfamide is particularly hazardous, since evidence suggests that ifosfamide nephrotoxicity is possibly potentiated by cisplatin. Ifosfamide-related proximal tubular damage is typically observed with a reduction in glomerular filtration rate, hypophosphatemia, and glycosuria (Oberlin et al. 2009). In one study by the Children’s Oncology Group, in which the cumulative dose of ifosfamide was 71 g/m2, Fanconi syndrome was described in approximately 7 % of patients (Goorin et al. 2002). Fanconi syndrome is a global proximal tubule defect in which resorption of glucose, amino acids, phosphate, and bicarbonate in the proximal tubule is impaired. Excessive urinary losses result in metabolic acidosis, hypophosphatemia, and hypokalemia. Although less prevalent, glomerular dysfunction can also develop, usually in patients who have evidence of tubular toxicity (Janeway and Grier 2010). When measured 6 months after the completion of therapy, 9 % of patients who received a median ifosfamide dose of 62 g/m2 had a glomerular filtration rate below 60 ml/min/1.73 m2. Although glomerular dysfunction is usually mild, acute renal failure can also occur, particularly at high cumulative doses. In most patients, ifosfamide-associated nephrotoxicity remains stable over time. A small proportion of patients will have significant improvements or worsening of both tubular and glomerular function (Heney et al. 1991). The risk of ifosfamide-associated nephrotoxicity is associated with higher cumulative doses, younger age at the time of administration, preexisting renal dysfunction, exposure to other nephrotoxic drugs, and treatment with cisplatin (Loebstein et al. 1999). Other than aggressive hydration, there are no specific therapies or modifications that are known to protect against the development of ifosfamide-related nephrotoxicity.
The COG long-term follow-up guidelines recommend annual blood pressure monitoring, annual urinalysis, and serum chemistries including BUN, creatinine at baseline and as clinically indicated for patients who received cisplatin and/or ifosfamide to monitor for renal toxicity (COG 2013).
16.3.3 Ototoxicity
Hearing loss as a late effect of therapy can occur after exposure to cancer-therapeutic agents such as platinum compounds and cranial radiation. Platinum agents cisplatin and carboplatin have improved cure rates in many childhood cancers including bone sarcomas, but their use may result in irreversible high-frequency sensorineural hearing loss. The deficit is progressive with increasing cumulative dosing (Grewal et al. 2010). In general, approximately 50 % of children treated with cisplatin-based regimens reportedly develop some degree of permanent hearing loss (Knight et al. 2005). With cumulative doses in excess of 400 mg/m2, typical for osteosarcoma treatment, up to 90 % of young children may suffer moderate-to-severe deficits, with severe hearing loss seen in up to 25 % (Knight et al. 2005).
The mechanism of platinum cochlear toxicity is through interference with signal transduction from the Organ of Corti in the cochlea (Grewal et al. 2010). Three sites of damage occur: the outer hair cells (effector cells), the spiral ganglion (main nerve supply to the cochlea), and the stria vascularis (primary blood supply) (Rybak et al. 2007). Chemotherapy-related damage begins in the first row of outer hair cells at the base of the cochlea, where high-frequency sounds are processed. Therefore, the use of platinum compounds can result in bilateral sensorineural hearing loss, which initially involves the higher frequencies (4,000–8,000 Hz) (Schell et al. 1989). With increasing doses of chemotherapy, or when compounded by other ototoxic factors such as radiation or aminoglycoside use, loss of hair cells can progress apically in the cochlea to involve the speech frequencies. High-frequency hearing sensitivity is critical for the understanding of speech. The speech frequencies are considered to be 500–2,000 Hz.
Major risk factors for hearing loss are younger age, higher cumulative dose of chemotherapy, central nervous system tumors, and concomitant CNS radiation. Individual susceptibility to cisplatin is variable. Dolan et al. demonstrated that 38–47 % of human variation in susceptibility to cisplatin-induced ototoxicity is due to genetic variables (Dolan et al. 2004). Children are more susceptible to ototoxicity from platinum agents than adults. For cisplatin, the risk of significant hearing loss involving the speech frequencies (500–2,000 Hz) usually occurs with cumulative doses that exceed 400 mg/m2 in pediatric patients, whereas adults may tolerate doses up to 600 mg/m2 before significant hearing loss involving the speech frequencies occurs (Schell et al. 1989). For carboplatin, ototoxicity has been reported to occur at similar cumulative doses in excess of 400 mg/m2. Other factors that may contribute to hearing loss include medications such as aminoglycoside antibiotics and loop diuretics, impaired renal function, and coexisting ear pathology such as chronic otitis or middle ear effusions (Grewal et al. 2010).
Clinically detectable hearing loss may require more than one cycle of a platinum agent to occur. Knight et al. reported a series of children who were receiving platinum compounds for a variety of oncologic diagnoses found a median time to observation of ototoxicity to be 135 days (Knight et al. 2005). No patient in that series showed improvement in hearing, suggesting that hearing loss is permanent. Progressive hearing loss as long as 136 months from the end of therapy with platinum compounds has been reported in a cohort of survivors of pediatric solid tumors (Bertolini et al. 2004).
Several otoprotectants are in clinical and preclinical trials, although their efficacy is unclear at present. Because cisplatin ototoxicity alters the antioxidant system of the outer hair cells, several agents that reduce oxidative stress in the cochlea have been tested (Rybak and Ramkumar 2007). Sodium thiosulfate is currently being tested by the Children’s Oncology Group in an intervention trial of pediatric patients treated with cisplatin (Hyppolito et al. 2006). Preclinical data indicated that this antioxidant confers otoprotection without affecting cytotoxicity (Neuwelt et al. 2006). After encouraging results in adult patients with ovarian cancer, amifostine as an otoprotectant was studied in children with high-risk germ cell tumors and osteosarcoma who received platinum-based regimens. Amifostine unfortunately did not lessen the risk of unacceptable hearing loss in either group and was limited by emesis in the patients with osteosarcoma (Gallegos-Castorena et al. 2007). In a retrospective review of patients with osteosarcoma, the incidence of ototoxicity was lower in patients who received cisplatin in two divided doses of 60 mg/m2/dose given 24 h apart than those who received a single dose of 120 mg/m2 (Lewis et al. 2009). The current standard treatment for osteosarcoma uses divided dosing of 60 mg/m2/dose daily over 2 days.
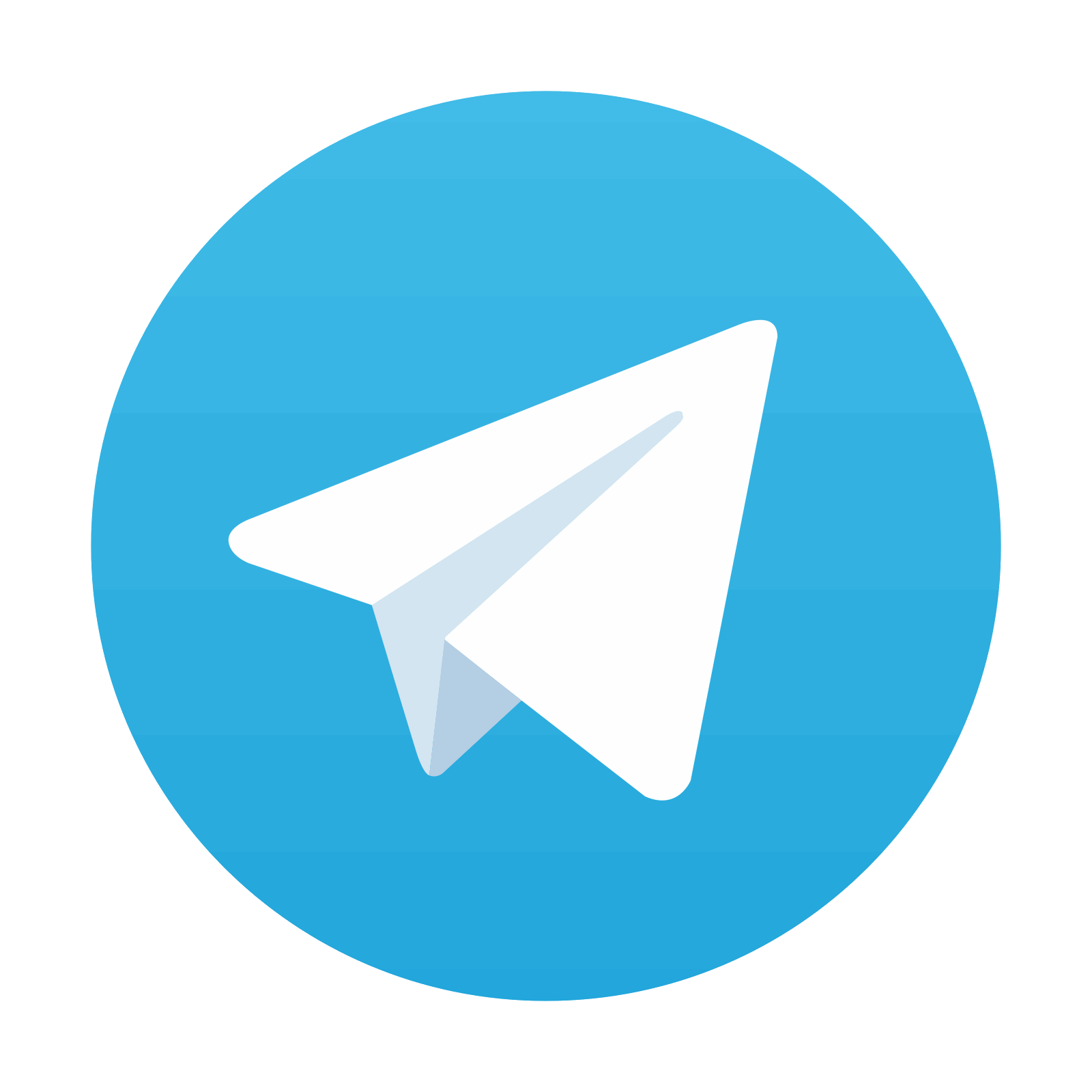
Stay updated, free articles. Join our Telegram channel

Full access? Get Clinical Tree
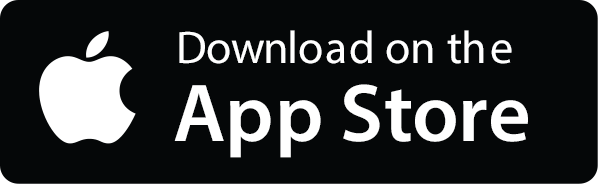
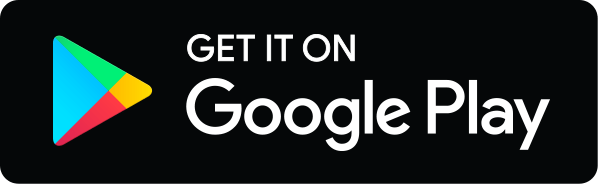