DEFINITION OF THE COMPLAINT
Seizures, a common neurologic disorder that occurs in childhood, affect 4%-6% of all children. A seizure is defined as a transient, involuntary alteration of consciousness, behavior, motor activity, sensation, and/or autonomic function caused by an excessive rate and hypersynchrony of discharges from a group of cerebral neurons. Epilepsy is diagnosed after two or more unprovoked seizures. In other words, recurrent seizures only occurring with precipitating events such as illness, fever, or acute head trauma are typically not considered epilepsy, even though antiepileptic drugs may be used to treat the seizures acutely. The first step in formulating a differential diagnosis in a child who presents with “seizures” is to characterize the type of event. Seizures are classified into generalized seizures (such as a tonic-clonic seizure affecting the entire body simultaneously) or focal seizures (arising from a specific area or areas of the cortex, with or without spread to the entire brain). The underlying cause is divided into three categories: genetic, structural/metabolic, and unknown cause. Genetic epilepsy is defined by seizures caused by a known or presumed genetic defect (e.g., SCN1A mutation). Structural/metabolic seizures are due to a lesion, such as stroke or tumor, but may also include infection. Tuberous sclerosis complex, while caused by a genetic mutation, is classified in this category because it is the structural changes in the brain, rather than being directly caused by the mutation itself, that result in epilepsy. The etiology of epilepsy that does not have a recognized genetic or structural/metabolic cause is classified as unknown.
This chapter will discuss possible causes of seizures. However, seizures should be differentiated from other childhood paroxysmal events that can mimic seizure activity. For example, prolonged syncope and clonic jerks occurring in the setting of a syncopal event may resemble a generalized tonic-clonic seizure. Syncope is the most common alternative diagnosis assigned to otherwise healthy patients who fall to the ground without warning. Atonic seizures or “drop attacks” in which a child loses muscle tone are rare and typically occur in children with other seizure types as well (e.g., myoclonic and tonic seizures). Gastroesophageal reflux with opisthotonic posturing (Sandifer syndrome) frequently mimics seizures in infancy. Breath-holding spells, which occur in approximately 4% of infants, can resemble seizures and are also associated with cyanosis. A variety of movement disorders, such as benign myoclonus of infancy and Tourette syndrome, may also be mistaken for seizures. Additionally, seizure activity is often subtle, making seizures difficult to diagnose. For example, in the neonatal period seizures may present with horizontal eye movements, repetitive sucking, or pedaling and stepping motions that are difficult to distinguish from normal newborn infant activity. In older children, childhood absence epilepsy is characterized by a brief impairment of consciousness manifesting as a stare or behavioral arrest and is often mistaken for attention deficit disorder.
COMPLAINT BY CAUSE AND FREQUENCY
It is important to remember that a seizure does not constitute a diagnosis but is a symptom of an underlying pathologic process that requires a thorough evaluation. The causes of seizures and development of epileptic syndromes in childhood vary by age (Table 19-1). Many of the diagnoses in this table, such as infections and drug toxicities, are not specific to one age group. However, they were included with certain age categories due to increased susceptibility within that age group. While the etiology of seizures should be classified as genetic, structural/metabolic, or unknown, it is often helpful to develop a differential diagnosis by classifying the cause of seizures by category (Table 19-2).
TABLE 19-1. Selected causes of seizures or epilepsy in childhood by age.
CLARIFYING QUESTIONS
Thorough history taking is essential to arrive at an accurate diagnosis in a child who presents with seizures. Consideration of seizure type, precipitating factors, and associated clinical features provides a useful framework for creating a differential diagnosis. The following questions may help to provide clues to the diagnosis:
• Did the seizure involve the entire body or only a portion?
—Focal (or partial) seizures reflect initial involvement from a localized lesion or lesions in the cerebral hemisphere(s). Focal seizures were previously classified on the basis of whether consciousness was impaired (complex partial seizure) or unaffected (simple partial seizure); however, these terms have been removed from the most recent classification recommendations for epilepsy. Although focal seizures are likely due to localized lesions, such structural causes are only found on magnetic resonance imaging (MRI) in 30%-50% of cases.
• Was there a preceding illness or fever?
—Febrile seizures occur most commonly in children 6 months to 5 years old. Febrile seizures occur in the setting of a systemic illness, such as a respiratory infection. Central nervous system infections, such as meningitis or encephalitis, are not considered febrile seizures but rather a separate category of primary central nervous system infection.
• Is there an ingestion or toxin exposure?
—Many medications and environmental toxins can lead to seizures including anticonvulsant medications, hypoglycemic agents, isoniazid, lithium, methylxanthines, heavy metals (e.g., lead), and tricyclic antidepressants.
• Is there recent headache, vomiting, lethargy, weakness, or alteration in gait?
—These symptoms suggest underlying central nervous system pathology and the need for neuroimaging. In neonates, the early onset of lethargy, vomiting, and seizures should prompt an evaluation for an underlying metabolic disorder, such as an aminoacidopathy, organic aciduria, and urea cycle defect; examples include phenylketonuria and maple syrup urine disease.
• Is there a history of previous seizures, febrile or afebrile, or neurologic abnormality?
—One-third of children with a simple febrile seizure will have a second episode. A previous afebrile seizure or existing neurologic abnormality increases the likelihood of a seizure disorder or epileptic syndrome.
• Is there a history of head trauma?
—Head trauma can result in epilepsy at any age. Seizures can occur within 1-2 weeks after the injury (early posttraumatic seizures), as an acute reaction to head trauma, or after intervals of several months or even years (late posttraumatic seizures). The risk of developing seizuresis related to the severity of the head injury. The child with a mild head injury (transient loss of consciousness without evidence of skull fracture or neurologic abnormality) is not at a significantly higher risk than the general population. Moderate head injuries are associated with an increased risk of epilepsy ranging from 2% to 10%. Children with severe head injuries, such as intracerebral hematoma or a history of unconsciousness more than 24 hours, have a 30% risk of developing epilepsy.
• Is there a history of a remote neurologic insult?
—A history of anoxic birth injury, cerebral palsy, stroke, intracranial hemorrhage, or meningitis places the child at increased risk for a seizure disorder. Intrauterine infection with cytomegalovirus, toxoplasma, or rubella is known to cause abnormal brain development and predispose the child to seizures.
• Are there skin abnormalities on physical examination, such as café-au-lait or ash leaf spots, or cutaneous vascular malformations?
—These findings suggest an underlying neurocutaneous disorder including neurofibromatosis, tuberous sclerosis complex, and Sturge-Weber syndrome.
• What is the child’s head morphology?
—Microcephaly suggests an underlying neurologic abnormality. A full or bulging fontanelle can signify elevated intracranial pressure due to meningitis, trauma, malignancy, or hydrocephalus.
• Is there a family history of seizures?
—Both febrile and afebrile seizures can be hereditary.
The following cases represent less common causes of seizures in childhood.
CASE 19-1
Eight-Day-Old Girl
HISTORY OF PRESENT ILLNESS
An 8-day-old girl presented to the emergency room after an episode of irregular, rapid breathing followed by stiffening of her body and shaking of her extremities that lasted several seconds. On arrival, the infant was lethargic, cyanotic, and brady-cardic with minimal spontaneous respirations. She underwent emergent endotracheal intubation and received multiple boluses of normal saline, with improvement in her perfusion and heart rate. She then had a generalized seizure and received intravenous lorazepam. Ampicillin and cefotaxime were administered after a blood culture was obtained. According to the family, she had fed poorly that day and had been sleeping more than usual. The infant had been afebrile and had normal stooling and urine output. There was no vomiting, diarrhea, or rashes. There were no ill contacts.
MEDICAL HISTORY
The infant weighed 3400 g at birth and was the product of a full-term gestation. She was born by spontaneous vaginal delivery after an uncomplicated pregnancy. Maternal serology was negative. The infant’s postnatal course was remarkable only for mild jaundice that did not require phototherapy. The mother denied a history of genital herpes simplex virus (HSV) infection. There was no family history of seizures.
PHYSICAL EXAMINATION
T 39.0°C; RR 20/min; HR 180 bpm; BP 86/45 mmHg; SpO2 100% in room air
Weight 25th percentile; Head Circumference 50th percentile
Examination revealed a mechanically ventilated infant. She was sedated but withdrew to painful stimuli. The fontanelle was bulging. There were no head lacterations or skull depressions. The sclera were anicteric, and the pupils were 1.5 mm and symmetrically reactive. There were no cardiac murmurs, and the femoral pulses were weakly palpable. The lungs were clear to auscultation. The abdomen was soft, and the umbilical stump was well healed without erythema or discharge. There were two pustules in the perineal area.
DIAGNOSTIC STUDIES
Laboratory results were as follows: sodium, 132 mEq/L; potassium, 3.3 mEq/L; chloride, 99 mEq/L; bicarbonate, 23 mEq/L; glucose, 73 mg/dL; calcium, 8.9 mg/dL; and magnesium, 2.1 mg/dL. The complete blood count revealed 8000 WBCs/mm3, including 33% band forms, 18% segmented neutrophils, 35% lymphocytes, and 10% monocytes. The hemoglobin and platelet count were normal. On cerebrospinal fluid (CSF) examination, there were 879 WBCs/mm3 (48% segmented neutrophils, 19% lymphocytes, and 33% monocytes) and 1739 RBCs/mm3; no organisms were seen on Gram staining. The CSF glucose was 36 mg/dL, and the protein concentration was 400 mg/dL. CSF was sent for bacterial culture and detection of HSV by polymerase chain reaction (PCR). There were no abnormalities on chest radiograph.
COURSE OF ILLNESS
In the intensive care unit, the patient received ampicillin, gentamicin, and acyclovir. A head computed tomogram (CT) was normal, and the patient’s neurologic examination improved quickly during the next day. She was extubated on the second day of hospitalization but required replacement of the endotracheal tube due to multiple episodes of apnea. Electroencephalogram (EEG) revealed status epilepticus. Sustained seizure control was observed only after the addition of phenobarbital and phenytoin. Because HSV was not detected in the CSF by PCR, acyclovir was discontinued. Growth of an organism from the CSF on the third day of hospitalization guided additional therapy.
DISCUSSION CASE 19-1
DIFFERENTIAL DIAGNOSIS
Seizures are a feature of almost all brain disorders in the newborn. The time of onset of the first seizure is helpful in determining the cause. The cause of neonatal seizures occurring after the first 72 hours of life includes intracranial infection, intracranial hemorrhage, metabolic abnormalities, developmental defects, and drug withdrawal. Intracranial infections occur in 5%-10% of neonatal seizures. Common bacterial causes in the age group include group B Streptococcus (GBS) and Escherichia coli; Listeria monocytogenes is less common. E. coli may cause ventriculitis or brain abscesses (Figure 19-1). Early-onset GBS infection typically includes bacteremia, pneumonia, and meningitis. Late-onset GBS infection (infection beyond the first week of life) may include meningitis with other foci of infection, such as osteomyelitis (Figure 19-2), arthritis, and cellulitis-adenitis syndrome. Seizures with HSV typically occur during the second week of life and 30% of infected infants present with a vesicular rash. Congenital infections, particularly cytomegalovirus and Toxoplasma gondii, may present with seizures in the context of intracranial calcifications. The calcifications tend to be periventricular for cytomegalovirus and diffuse for toxoplasmosis (Figure 19-3). Intracranial hemorrhages are frequently associated with hypoxic-ischemic or traumatic birth injury. Intraventricular hemorrhages principally occur in the premature infant, and subarachnoid and subdural hemorrhages usually occur in the term infant. Metabolic abnormalities include disturbances of glucose, calcium, magnesium, and sodium. Hypocalcemia is associated with low birth weight, asphyxia, maternal diabetes, transient neonatal hypoparathyroidism, and micro-deletions of chromosome 22q11. Other metabolic abnormalities include inborn errors of metabolism, especially aminoacidurias, because protein and glucose feedings have been initiated. Aberrations of brain development are usually related to a disturbance of neuronal migration such as lissencephaly, pachygyria, and polymicrogyria. Passive addiction of the newborn and drug withdrawal may involve narcotic-analgesics (methadone), sedative-hypnotics (shorter-acting barbiturates), cocaine, alcohol, and tricyclic antidepressants. In the case described, the results of CSF analysis suggested an intracranial infection, but interpretation of the Gram stain was misleading.
FIGURE 19-1. Brain MRI of an infant with meningitis caused by Escherichia coli. The MRI shows a large multiseptated cystic lesion within the right cerebral hemisphere with irregular peripheral enhancement. There is inflammation of the adjacent lateral ventricle and choroid with mass effect.
FIGURE 19-2. Lower leg radiograph of an infant with late onset group B Streptococcal osteomyelitis. The radiograph demonstrates an area of focal bony erosion (arrow) at the medial metaphyseal region of the proximal right tibia associated with faint periosteal reaction.
FIGURE 19-3. Computed tomography of the head in an infant with congenital toxoplasmosis. There are scattered intracranial calcifications, predominantly involving the cortex and subcortical white matter.
DIAGNOSIS
The diagnosis is meningitis due to L. monocytogenes, a Gram-positive rod. On the fourth day of hospitalization, the organism was noted to have only intermediate susceptibility to ampicillin. The patient was switched to intravenous vancomycin, and gentamicin was continued. CSF from the lumbar puncture, repeated on the sixth day of hospitalization, was sterile. Head CT was repeated on the eighth day of hospitalization and revealed bilateral frontal, parietal, and temporal lobe infarcts but no ventriculomegaly. Mechanical ventilation was required until the ninth day of hospitalization. The infant was discharged after 21 days of antibiotic therapy.
INCIDENCE AND EPIDEMIOLOGY
Listeria monocytogenes, a motile Gram-positive rod, was first isolated in 1926 during an investigation of epidemic perinatal infection among a colony of rabbits. It is a common veterinary pathogen that causes meningoencephalitis in sheep and cattle. It is widespread in the environment and is found commonly in soil and decaying vegetation. Many foods are contaminated with this organism; it has been recovered from raw vegetables, fish, poultry, beef, prepared meats, unpasteurized milk, and certain types of cheese. The organism has been isolated from the stools of 5% of healthy adults, and higher rates of recovery have been reported for household contacts of patients with clinical infection. Infection in humans is uncommon but occurs most frequently in neonates, pregnant women, and elderly or immunosup-pressed patients. Human infection occurs most commonly following ingestion of contaminated food, but it can also occur through direct animal contact, as has been documented in veterinaries and farmers, and through vertical transmission from mother to neonate, either transplacentally or through an infected birth canal. Approximately 30% of all L. monocytogenes infections occur in neonates.
CLINICAL PRESENTATION
Neonatal L. monocytogenes infection, like group B streptococcal infection, manifests in both an early-and late-onset form. Clinical manifestations of L. monocytogenes infection are similar to those of other neonatal bacterial infections. Signs of infections include temperature instability, respiratory distress, irritability, lethargy, and poor feeding.
In early-onset disease, transplacental transmission after maternal bacteremia or ascending spread from vaginal colonization leads to intrauterine infection with L. monocytogenes. The neonate can also acquire the infection during passage through an infected birth canal. Pregnancy complications, including preterm labor, spontaneous abortion, and stillbirth, are common among infants with early-onset L. monocytogenes infections; length of gestation is less than 35 weeks in approximately 70% of cases. There is often evidence of an acute febrile maternal illness, with symptoms of fatigue, arthralgias, and myalgias preceding delivery by 2-14 days. Blood cultures are positive for L. monocytogenes in 35% of mothers of infants with early-onset listeriosis.
Early-onset infection classically develops within the first or second day of life. Bacteremia (75%) and pneumonia (50%) are usually seen with early-onset infection. Meningitis is seen in 25% of early-onset cases. In severe infection, a granulomatous rash is associated with disseminated disease (granulomatosis infantiseptica). The mortality rate, including stillbirths, is 20%-40% in early-onset infections.
In late-onset infection, the mode of transmission is poorly understood but mechanisms unrelated to maternal carriage may be involved. Late-onset infection develops during the second to eighth week of life, often in full-term infants following uncomplicated delivery. The most common form of L. monocytogenes infection during this period is meningitis, which is present in approximately 95% of cases. Bacteremia (20%) and pneumonia (10%) are less common. Mortality of late-onset infection is generally low (15%) if the infection is diagnosed early and treated appropriately. The presentation of late-onset infection can be subtle, and may be characterized by temperature instability, irritability, poor feeding, and lethargy.
A nosocomial outbreak occurred when nine newborn infants were bathed in mineral oil contaminated with L. monocytogenes. The affected infants developed bacteremia (two cases), meningitis (two cases), or both (five cases); one infant died. Signs of infection developed within 1 week after exposure to the mineral oil.
DIAGNOSTIC APPROACH
Lumbar puncture. Isolation of the organism from culture of CSF is the only reliable means of diagnosing meningitis due to L. monocytogenes. The finding of short, sometimes coccoid, Gram-positive rods on microscopic examination of the CSF strongly supports the diagnosis of L. monocytogenes meningitis. However, because of the low concentrations of organisms, most (60%) Gram-stained smears of CSF from infants with L. monocytogenes meningitisdo not reveal bacteria, as occurred with the infant in this case.
Furthermore, L. monocytogenes sometimes does not stain clearly as Gram positive. In such cases, variable decoloration on Gram staining may cause the organism to appear as a Gram-negative rod and be confused with H. influenzae, especially with longstanding disease or when the patient has received prior antibiotics. In other instances, Listeria has been mistaken for Streptococcus pneumoniae or Corynebacterium spp. CSF glucose is normal in more than 60% of cases of L. monocytogenes meningitis. CSF is often purulent with mononuclear cells predominance in one-third of cases. CSF protein is often elevated, with increasing levels correlated with a poor prognosis.
Additional studies. PCR probes and antibodies to listeriolysin O, the major virulence factor of the organism, have not proved useful for acute diagnosis of invasive disease.
TREATMENT
Ampicillin is the preferred agent in the treatment of L. monocytogenes infections. Based on synergy studies in vitro and in animal models, most authorities suggest adding gentamicin to ampicillin for the treatment of meningitis due to L. monocytogenes. There appears to be partial synergy with combinations of ampicillin or vancomycin with rifampin. Vancomycin alone has been used successfully in a few penicillin-allergic adult patients, but others have developed listerial meningitis while receiving the drug. Trimethoprim-sulfamethoxazole is effective in penicillin-allergic patients, but should not be used in neonates because of the concern for bilirubin toxicity. Cephalosporins are not active against L. monocytogenes. Once susceptibility studies become available, changes in therapy may be necessary. The recommended duration of therapy for L. monocytogenes meningitis is 14-21 days.
Corticosteroids should be avoided, if possible, because impairment of cellular immunity due to corticosteroid therapy is a major risk factor for the development of listeriosis. A maternal history of a previous infant with perinatal listeriosis is not an indication for intrapartum antibiotics.
SUGGESTED READINGS
1. Lorber B. Listeria monocytogenes. In: Long SS, ed. Principles and Practice of Pediatric Infectious Diseases. 4th ed. Philadelphia, PA: W.B. Saunders Company; 2012;762-767.
2. Lorber B. Listeriosis. Clin Infect Dis. 1997;24:1-11.
3. Posfay-Barbe KM, Wald ER. Listeriosis. Semin Fetal Neonatal Med. 2009;14:228-223.
4. Schuchat A, Lizano C, Broome CV, et al. Outbreak of neonatal listeriosis associated with mineral oil. Pediatr Infect Dis J. 1991;10:183-189.
5. Southwick FS, Purich DL. Mechanisms of disease: intra-cellular pathogenesis of listeriosis. New Engl J Med. 1996; 334:770-776.
CASE 19-2
Ten-Day-Old Boy
HISTORY OF PRESENT ILLNESS
The patient is a 10-day-old boy who was well until the day of admission when he had a sudden onset of left arm and leg shaking while sleeping. The episode lasted about 1 minute and was accompanied by eyelid fluttering. After spontaneous cessation of the episode, the infant continued sleeping but was aroused easily. He was brought to the emergency department for evaluation. He did not have fevers or cyanosis. There was no recent vomiting or diarrhea. His oral intake had been unchanged during the past several days, consisting exclusively of cow-milk-based formula every 2.5-3 h. The parents were uncertain about urine output since the maternal grandmother had cared for the infant the day prior to admission.
MEDICAL HISTORY
The infant weighed 3600 g, born by spontaneous vaginal delivery after an uncomplicated pregnancy. The infant required phototherapy briefly on the second day of life for hyperbilirubinemia with a peak total bilirubin level of 15.5 mg/dL. The mother had vaginal colonization with group B Streptococcus and received two doses of penicillin during labor. She also had a history of genital herpes simplex virus infection. Although no lesions were noted at delivery, she did develop lesions on the seventh postpartum day.
PHYSICAL EXAMINATION
T 37.5°C; HR 124 bpm; RR 40/min; BP 75/45; SpO2 100% in room air
Weight 50th percentile; Length 25th percentile; Head Circumference 25th percentile
The infant appeared alert. There were no vesicles on the scalp or skin. His anterior fontanelle was open and flat. His conjunctivae were pink and anicteric. Red reflex was present bilaterally. There was no murmur on cardiac examination and femoral pulses were strong. The spleen tip was just palpable and there was no hepatomegaly. The Moro reflex was symmetric. The remainder of the examination was normal.
DIAGNOSTIC STUDIES
Complete blood count revealed 8800 WBCs/mm3 (16% segmented neutrophils, 70% lymphocytes; 11% monocytes; and 3% atypical lymphocytes); hemoglobin, 13.4 g/dL; and platelets, 511 000/mm3. Serum chemistries included sodium, 139 mmol/L; potassium, 5.5 mmol/L; chloride, 104 mmol/L; and bicarbonate, 28 mmol/L. The blood urea nitrogen and creatinine were normal. Serum alanine and aspartate aminotransferases were normal. Serum albumin was 3.3 g/dL. Examination of the cerebrospinal fluid revealed the following: WBCs, 12/mm3; RBCs, 1834/mm3; glucose, 45 g/dL; and protein, 124 g/dL. There were no bacteria on Gram stain.
COURSE OF ILLNESS
They infant was treated empirically with ampicillin, cefotaxime, and acyclovir while awaiting the results of CSF bacterial culture and CSF HSV PCR. An ECG (Figure 19-4) suggested a cause of the seizures, which was confirmed by additional blood tests in both the infant and his mother.
FIGURE 19-4. Electrocardiogram.
DISCUSSION CASE 19-2
DIFFERENTIAL DIAGNOSIS
Many neonatal seizures are idiopathic. The most common definable etiologic agents include asphyxia, intracranial infection, trauma, nontraumatic hemorrhage, strokes, metabolic disorders, central nervous system malformations, and maternal drug abuse. Seizures due to perinatal asphyxia typically occur within the first 24 hours of life. Common infectious causes in the first week of life include bacterial meningitis due to group B Streptococcus and Escherichia coli. Neonates with herpes simplex meningitis typically present during the second week of life but up to 40% develop symptoms within the first 5 days of life. Intracranial hemorrhage of any cause can provoke seizures. Neonatal seizures related to birth trauma with subsequent subarachnoid hemorrhage or subdural and epidural hematomas usually occur within the first 72 hours of life. Non-traumatic causes of intracranial hemorrhage including ruptured arteriovenous malformations and underlying disorders of coagulation can occur at any time. Metabolic disorders include hypocalcemia, hypoglycemia, and pyridoxine dependency. Neonatal hypocalcemia occurring after the third day of life is usually due to transient relative hypoparathyroidism. The immature neonatal parathyroid may be unable to handle an excessive phosphate load, particularly when the infant is fed a formula with a relatively low ratio of calcium to phosphorus. Rarely, prolonged phototherapy induces hypocalcemia. Phototherapy decreases melatonin secretion, which in turn decreases glucocorticoid secretion, which leads to an increase in bone calcium uptake with subsequent hypocalcemia. Multiple defects in urea cycle and organic acid metabolism may cause seizures during the neonatal period. Infants with these disorders usually have unexplained stupor, coma, and vomiting in addition to seizures. Infants born to mothers who have used heroin or metha-done may have seizures, although other symptoms such as poor feeding, diarrhea, sweating, jitteriness, and irritability are more common.
DIAGNOSIS
Bacterial cultures and HSV PCR of the CSF were negative. The ECG demonstrated QTc prolongation (QTc = 0.47 seconds) characteristic of hypocalcemia (Figure 19-4). The infant’s serum calcium was 6.6 mg/dL (normal range 8.8-10.1 mg/dL); ionized calcium, 0.83 mmol/L (normal range 1.00-1.17 mmol/L); phosphate, 10.6 mg/dL (normal range 4.8-8.2 mg/dL); and magnesium, 1.1 mg/dL (1.5-2.5 mg/dL). Additional testing included intact parathyroid hormone, 9.7 pg/mL (normal range 10-55 pg/mL); 25-hydroxy vitamin D, 7 ng/mL (normal range 5-42 ng/mL); and active vitamin D (1-alpha-25-dihydroxy-cholecalciferol) (1,25(OH)2D), 114 pg/mL (normal range 8-72 pg/mL). See Table 19-3 for the differential diagnosis of hypocalcemia in an infant. Although the mother was asymptomatic, her calcium level was elevated to12.8 mg/dL. The mother was subsequently diagnosed with hyperparathyroidism related to a parathyroid adenoma. The infant was diagnosed with transient neonatal hypoparathyroidism secondary to maternal hyperparathyroidism. The infant was initially treated with intravenous calcium gluconate followed by oral calcium and vitamin D supplementation, which were weaned over the subsequent 3 weeks.
TABLE 19-3. Differential diagnosis of neonatal hypocalcemia.
INCIDENCE AND EPIDEMIOLOGY OF TRANSIENT NEONATAL HYPOPARATHYROIDISM
Hyperparathyroidism has a prevalence rate of 0.15% with a peak incidence between 30 and 50 years of age. Approximately 80% are due to a solitary adenoma that requires resection and 15% are due to chief cell hyperplasia. Maternal symptoms are not apparent until the serum calcium level exceeds 12-13 mg/dL. However, even mild maternal hypercalcemia leads to chronic fetal hypercalcemia, which in turn suppresses fetal PTH production. After birth, calcium levels decrease but PTH production cannot be rapidly increased. In this condition, neonatal hypoparathyroidism is transient, lasting only several days to several weeks. Eventually, as the parathyroids become more active, increasing PTH levels stimulate vitamin D production and extra calcium absorption from the plentiful supply in the gut. Clinically detectable hypocalcemia develops in 15%-25% ofinfants born to mothers with hyperparathyroidism. As in this case, neonatal seizures or tetany often lead to a search that identifies a maternal parathyroid adenoma.
CLINICAL PRESENTATION OF TRANSIENT NEONATAL HYPOPARATHYROIDISM
Signs of hypocalcemia usually develop within the first 3 weeks of life. Signs of neonatal hypocalcemia are often nonspecific and may be seen in a variety of other conditions. Tremors and jitteriness are most commonly seen. Other signs include irritability, hyperreflexia, facial twitching, carpalpedal spasm, seizures, cyanosis, and, rarely, laryngospasm. More importantly, other disorders that can present with hypocalcemia should be considered. Features of 22q11 deletion syndromes include cleft palate, micrognathia, ear anomalies, bulbous nasal tip, and conotruncal heart defects. Findings associated with Albright hereditary osteodystrophy (pseudohypoparathyroidism type Ia) include round face, short distal phalanges of the thumbs, subcutaneous calcifications, and a family history of developmental delay and dental hypoplasia. Sensorineural deafness, renal dysplasia, and mental retardation are also associated with syndromes that include hypoparathyroidism.
DIAGNOSTIC APPROACH
Serum calcium and ionized calcium. Both calcium and ionized calcium levels are low with symptomatic hypocalcemia.
Serum albumin. Since approximately 45% of serum calcium is protein bound, low serum albumin levels lead to low serum calcium levels but normal ionized levels. Symptoms of hypocalcemia develop only when ionized calcium is low. The following correction factor approximates whether a low measured serum calcium level is due solely to hypoalbuminemia: Corrected serum calcium = measured serum calcium + [(Normal serum albumin – measured serum albumin) × 0.8].
When the corrected serum calcium is less than normal (<8.8 mg/dL), the ionized calcium may also be low, increasing the likelihood of symptomatic hypocalcemia. In this patient, the corrected serum calcium was calculated as follows: [6.6 mg/dL + (4.0 mg/dL – 3.3 mg/dL) × 0.8] = 7.1 mg/dL = Corrected calcium.
Serum magnesium. Magnesium deficiency can lead to neonatal hypocalcemia through functional hypoparathyroidism and pseudohypoparathyroidism. In most cases, it is seen in neonates born to magnesium-deficient mothers, such as those with poorly controlled diabetes mellitus. In magnesium deficiency, magnesium replenishment leads to increases in both calcium and PTH levels. In hypoparathyroidism of any other cause, magnesium administration does not lead to changes in the calcium and PTH levels.
Serum phosphorus. Phosphorus levels are elevated with both phosphate-induced neonatal hypocalcemia and with hypoparathyroidism.
Serum PTH. PTH levels are low with hypoparathyroidism. However, in phosphate-induced neonatal hypocalcemia, serum PTH is appropriately elevated.
Active vitamin D. Levels of 1,25(OH)2D are low with hypocalcemia due to vitamin D deficiency but normal or high with underlying hypoparathyroidism.
Other tests. Infants who were treated with bicarbonate or other alkali to correct acidosis can develop very significant hypocalcemia, therefore, an arterial blood gas should be considered. A chest radiograph can document a normal thymic shadow in neonates when 22q11 deletion syndromes are a concern. When neonatal risk factors for hypocalcemia are absent, consider measuring maternal serum calcium, phosphorus, and PTH levels.
TREATMENT
Emergency treatment for neonatal hypocalcemia consists of intravenous 10% calcium gluconate infusion with continuous electrocardiographic monitoring. Additionally, 1,25-dihydroxycholecalciferol (vitamin D3; calcitriol) should be given. Once the QTc interval on ECG is normal, therapy can be continued with oral calcium and vitamin D2 (ergocalciferol), which is less costly than calcitriol. Serum calcium levels should be measured frequently in the early stages of treatment to determine the appropriate dosing. If hypercalcemia occurs, therapy should be discontinued and resumed at a lower dose after the serum calcium level has returned to normal. When maternal hyperparathyroidism is the cause of neonatal hypoparathyroidism and hypocalcemia, supplementation with calcium and vitamin D analogs is only required for 3-4 weeks.
SUGGESTED READINGS
1. Hsieh YY, Chang CC, Tsai HD, et al. Primary hyperparathyroidism in pregnancy: report of three cases. Arch Gynecol Obstetrics. 1998;261:209-214.
2. Kaplan EL, Burrington JD, Klementschitsch P, Taylor J, Deftos L. Primary hyperparathyroidism, pregnancy, and neonatal hypocalcemia. Surgery. 1984;96:717-722.
3. Rubin, LP. Disorders of calcium and phosphorus metabolism. In: Taeusch HW, Ballard RA, eds. Avery’s Diseases of the Newborn. 7th ed. Philadelphia, PA: WB Saunders Company; 1998:1189-1191.
4. Mimouni FB, Root AW. Disorders of calcium metabolism in the newborn. In: Sperling MA, ed. Pediatric Endocrinology. Philadelphia, PA: W.B. Saunders Company; 1996:95-115.
5. Morrison A. Neonatal seizures. In: Pomerance JJ, Richardson CJ, eds. Neonatology for the Clinician. Norwalk, CT: Appleton & Lange; 1993:411-423.
6. Romagnoli C, Polidori G, Cataldi L, Tortorolo G, Segni G. Phototherapy induced hypocalcemia. J Pediatr. 1979;94: 815-816.
CASE 19-3
Eight-Month-Old Boy
HISTORY OF PRESENT ILLNESS
The patient is an 8-month-old boy who was well until 1 week prior to admission when he was found by his mother having a “seizure.” He had shaking and jerking of all extremities that did not stop when his extremities were held. He did not respond to touch or stimulation. There was no cyanosis. The episode lasted approximately 15 minutes. On arrival of Emergency Medical Services, the patient was alert and feeding from a bottle. He was not taken to the hospital. His last feeding was approximately 3 hours prior to the event. Two days later the patient was evaluated by his primary physician who performed the following laboratory evaluation: Glucose (during feeding), 121 mg/dL; alanine aminotransferase (ALT), 73 U/L; aspartate aminotransferase (AST), 93 U/L; gamma glutamyl transferase (GGT), 28 U/L; and cholesterol, 423 mg/dL. These labs were repeated 2 days later with similar results except that the glucose was 16 mg/dL. Head CT and EEG were normal. He was hospitalized for additional evaluation.
MEDICAL HISTORY
The patient was born at 38 weeks gestation with a birth weight of 3400 g. His delivery was complicated by meconium aspiration. He was treated with supplemental oxygen and empiric antibiotics for 3 days. He also had hypoglycemia requiring intravenous dextrose and bottle feedings every 1.5 hours. This resolved and he was discharged home on the fourth day of life. He received oral antibiotics for otitis media diagnosed at 3 months of life. There is no family history of seizures or mental retardation.
PHYSICAL EXAMINATION
T 36.2°C; RR 20/min; HR 90-110 bpm; BP 120/55 mmHg; SpO2 100% in room air
Height 25th percentile; Weight 10th percentile; Head Circumference 25th percentile
On examination, he was thin but playful. The anterior fontanelle was open and flat. The heart sounds were normal and the lungs were clear to auscultation. His abdomen was slightly protuberant with a liver edge that was firm and palpable 6 cm below the right costal margin. The spleen tip was just palpable below the left costal margin. There was no ascites or palpable abdominal mass. The infant was circumcised and had normal male genitalia. The neurological examination was normal. The child was awake and alert and interactive with the parents and examiner. He made excellent eye contact and babbled during the examination. Pupils were equal, round, and reactive to light. Funduscopic examination was normal. He tracked in all directions without nystagmus. Facial movements were symmetric. The gag reflex was intact. He had antigravity movements and normal axial and appendicular tone with passive range of motion and vertical and horizontal suspension. The infant was able to sit without support. He withdrew to pain in all extremities. Deep tendon reflexes were 2+ throughout and symmetric. The plantar responses were flexor (upgoing Babinski, normal for age). There were no hyper- or hypopigmented skin lesions.
DIAGNOSTIC STUDIES
Serum chemistries included sodium, 137 mmol/L; potassium, 5.5 mmol/L; chloride, 100 mmol/L; bicarbonate, 13 mmol/L; calcium, 10.5 mg/dL; phosphorous, 6.5 mg/dL; and serum glucose 20 mg/dL. The cholesterol and triglycerides were 465 mg/dL and 4070 mg/dL, respectively. Hepatic function tests included AST, 125 U/L; ALT, 155 U/L; GGT, 564 U/L; total bilirubin 0.6 mg/dL; and albumin 4.0 g/dL. Serum and urinary ketones were present. White blood cell count, hemoglobin, and platelet count, as well as prothrombin and partial thromboplastin times were normal. Blood, urine, and stool cultures were obtained.
COURSE OF ILLNESS
The patient underwent a fasting study which revealed the diagnosis within approximately 4 hours.
DISCUSSION CASE 19-3
DIFFERENTIAL DIAGNOSIS
This infant had seizures related to hypoglycemia. Hypoglycemia in an infant, defined as a blood glucose concentration ≤40 mg/dL, warrants immediate treatment followed by appropriate investigation. Many inborn errors of metabolism are responsible for hypoglycemia present in the first year of life, while milder defects of glycogen degradation and gluconeogenesis manifest only in childhood after prolonged periods of fasting. Causes of hypoglycemia in an infant include hyper-insulinism, hormone deficiency, and defects in branched-chain amino acid metabolism, fatty acid oxidation, and hepatic enzymes.
Urinary ketones are absent or low in children with hyperinsulinism and fatty acid oxidation defects who present with hypoglycemia. Hypoglycemia secondary to hyperinsulinism most commonly appears during the first year of life. It is usually associated with islet-cell dysplasia and rarely with islet-cell adenomas. Insulin level are elevated (>5 μU/mL) and injection of glucagon elicits a rapid rise in blood glucose levels. Children with disorders of fatty acid metabolism can present with hypoglycemia and profound disturbance of consciousness that may not improve when the plasma glucose is normalized. In addition to hyperketonemia, they have high plasma free fatty acid concentrations, elevated ALT and AST, rhabdomyolysis, cardiomyopathy, and cerebral edema.
The presence of urinary ketones usually suggests hormone deficiency, glycogen storage disease, or defects in gluconeogenesis. Hypoglycemia is a common presentation for an infant with panhypopituitarism, isolated growth hormone deficiency, and absolute (adrenal hypoplasia, Addison disease, adrenoleukodystrophy) or relative (congenital adrenal hyperplasia) glucocorticoid deficiency. Midline defects such as cleft lip or palate, optic dysplasia, and microphallus suggest anterior pituitary hormone deficiency. Hyperpigmentation associated with Addison disease rarely occurs in young children. Addison disease is occasionally associated with hypoparathyroidism (hypocalcemia). Severely compromised adrenal function, as in congenital adrenal hyperplasia, may lead to serum electrolyte disturbances or ambiguous genitalia.
Children with branched-chain ketonuria (maple syrup urine disease) excrete urinary ketoacids that impart the characteristic odor of maple syrup. Clinically, these infants have frequent hypoglycemic episodes, lethargy, vomiting, and muscular hypertonia. Glycogen storage diseases are inherited autosomal recessive defects characterized by either deficient or abnormally functioning enzymes involved in the formation or degradation of glycogen. Hepatomegaly, growth failure, hyper-lipidemia, and hyperuricemia are common clinical features. Other disorders to consider include galactosemia, especially in children with hepatosplenomegaly, jaundice, and mental retardation, and fructose- 1,6-diphosphatase deficiency, in children with hepatomegaly due to lipid storage but only mildly abnormal liver function studies.
DIAGNOSIS
After 4 hours, the glucose was 16 mg/dL; lactate, 32 mg/dL (normal range 5-18 mg/dL); and uric acid 14.2 mg/dL (normal range 2-7 mg/dL). He received intravenous glucagon (30 mcg/kg) after which the blood glucose concentration was 22 mg/dL and the lactate level increased to 44 mg/dL. He then received oral glucose and his blood glucose increased to 65 mg/dL and the lactate decreased to 24 mg/dL. These findings suggested type IA glycogen storage disease (von Gierke disease). Liver biopsy demonstrated increased glycogen content and deficient G6P enzyme activity (2 nmol/min/mg protein; normal range, 20-70 nmol/min/mg protein).
INCIDENCE AND EPIDEMIOLOGY OF GLYCOGEN STORAGE DISEASE
The glycogen storage diseases (GSDs) or glycogenoses comprise several inherited diseases caused by deficiency in one of the enzymes that regulate the synthesis or degradation of glycogen. The end result is abnormal accumulation of glycogen in various tissues. GSD type I has an estimated incidence of 1 in 200 000 births. GSD type IA is due to deficiency of the enzyme glucose-6-phosphatase (G6P), which catalyzes the breakdown of stored glycogen into glucose for use by the body. At least 56 different mutations in the gene for G6P enzyme (chromosome 17q21) have been found in patients with GSD type Ia. GSD types IB and IC are caused by failure of the G6P transporter (type IB) and microsomal phosphate transporter (type IC), which ultimately impair G6P activity. The three types of GSD result in similar clinical and biochemical disturbances. G6P is expressed in the liver, kidneys, and intestines.
CLINICAL PRESENTATION OF GLYCOGEN STORAGE DISEASE
GSD type I is characterized by severe hypoglycemia within 3-4 hours after a meal. Although symptomatic hypoglycemia may appear soon after birth, most patients are asymptomatic as long as they receive frequent feeds that contain sufficient glucose to prevent hypoglycemia. Symptoms of hypoglycemia appear only when the interval between feedings increases, such as when the child begins to sleep through the night or when an intercurrent illness disrupts normal feeding patterns.
Patients may have hyperpnea from lactic acidosis. Untreated patients have poor weight gain and growth retardation. Most patients have a protuberant abdomen and hepatomegaly due to glycogen deposition and fatty infiltration. Social and cognitive development are normal unless the infant suffers neurologic impairment after frequent hypoglycemic seizures. Xanthomas may appear on the extensor surfaces of the extremities and buttocks. Older children develop gout.
DIAGNOSTIC APPROACH
Fasting study. In GSD, the liver fails to release sufficient glucose from hepatic stores to meet peripheral tissue demands. The consequence of this “fasting state” is hypoglycemia, which causes lipolysis and protein breakdown. Therefore, in GSD, hypoglycemia is accompanied by elevated lactic acid, uric acid, and metabolic acidosis. Serum insulin level is low but serum and urinary ketones are markedly elevated. Glucagon does not significantly alter glucose level and actually increases lactic acid levels. An oral glucose load increases serum glucose and decreased lactic acid levels. At the time of hypoglycemia, serum should be collected for insulin, C-peptide, growth hormone, beta-hydroxybutyrate, lactate, and free fatty acids. Urine may be analyzed for organic acids, ketones, and reducing substances. This combination of studies allows for the diagnosis of GSD as well as the exclusion of other disorders that present with hypoglycemia.
Liver function tests. Mild elevations of AST and ALT occur.
Lipid profile. Markedly elevated serum triglycerides, free fatty acids, and apolipoprotein C-III. Infants with triglyceride levels greater than 1000 mg/dL are at high risk for developing acute pancreatitis. Despite the hypertriglyceridemia, the risk for cardiovascular disease is not increased.
Complete blood count. Neutropenia develops with GSD type IB but not with type IA.
Bleeding time. Although this test is not routinely performed, most children with GSD type I have impaired platelet function due to systemic metabolic abnormalities. This bleeding tendency, manifested by recurrent epistaxis and prolonged bleeding after surgery, resolves with correction of the metabolic abnormalities.
Urinalysis. Glycosuria and proteinuria indicate proximal renal tubular dysfunction that improves with correction of metabolic abnormalities.
Abdominal ultrasound. Hepatic adenomas occur in the majority of patients by the second decade of life but may be noted before puberty. Women also usually have polycystic ovaries, a finding whose clinical significance remains unclear.
Other studies. Measurement of G6P enzyme activity in a fresh liver biopsy specimen can be used to diagnose GSD IA. Molecular analysis to identify mutations on the G6P gene is a reliable alternative to liver biopsy.
TREATMENT
Treatment consists of providing a continuous dietary source of glucose to prevent hypoglycemia. When hypoglycemia is prevented, the biochemical abnormalities and growth improve and liver size decreases. Infants require frequent feeding, approximately 2-3 hours during the day and every 3 hours at night. A variety of methods can be followed to provide a continuous source of glucose at night in older children, including intravenous dextrose infusion, continuous intragastric feeding via a nasogastric or gastrostomy tube, and the use of low glycemic index foods such as cornstarch. Orally administered uncooked cornstarch seems to act as an intestinal reservoir of glucose that is slowly absorbed into circulation. It has been used successfully in infants as young as 8 months of age and may obviate the need for continuous intragastric infusion of formula overnight. It can be mixed with water, formula, or artificially sweetened fluids in 4-6-hour intervals overnight. The optimal schedule requires validation by serial glucose monitoring. Allopurinol and lipid-lowering agents are used for severe uric acid and lipid abnormalities. Hepatocyte infusion and liver transplantation may be curative, but the long-term complications in children with GSD are not yet known.
SUGGESTED READINGS
1. Lee PJ, Patel A, Hindmarsh PC, Mowat AP, Leonard JV. The prevalence of polycystic ovaries in the hepatic glycogen storage diseases: its association with hyperinsulinism. Clin Endocrinol. 1995;42:601-606.
2. Rake JP, ten Berge AM, Visser G, et al. Glycogen storage disease type Ia: recent experience with mutation analysis, a summary of mutations reported in the literature and a newly developed diagnostic flow chart. Eur J Pediatr. 2000;159:322-330.
3. Sperling MA, Finegold DN. Hypoglycemia in the child. In: Sperling MA, ed. Pediatric Endocrinology. Philadelphia, PA: W.B. Saunders Company; 1996;265-279.
4. Willi SM. Glycogen storage diseases. In: Altschuler SM, Liacouras CA, eds. Clinical Pediatric Gastroenterology. Philadelphia, PA: Churchill Livingstone; 1998:377-383.
5. Wolfsdorf JI, Holm IA, Weinstein DA. Glycogen storage diseases. Endocrinol Metabol Clinics. 1999;28:801-823.
6. Taub KS, Abend NS. Seizure disorders. In: Stockwell JA, Preissig CM, eds. Comprehensive Critical Care: Pediatric. Mount Prospect, IL: Society of Critical Care Medicine; 2012:447-468.
7. Berg AT, Berkovic SF, Brodie MJ, et al. Revised terminology and concepts for organization of seizures and epilepsies: report of the ILAE Commission on Classification and Terminology, 2005-2009. Epilepsia. 2010;51(4):676-685.
Three-Year-Old Boy
HISTORY OF PRESENT ILLNESS
The patient was a 3-year-old boy with a history of developmental delay who was in his usual state of health until 1 day prior to admission when he developed a tactile fever and had one episode of nonbloody, nonbilious emesis. On the day of admission, he was noted to have poor oral intake and decreased activity. The evening of admission he was found lying on the kitchen floor. He had abnormal eye movements and twitching of his mouth. According to his mother, this episode lasted for 20 minutes and was followed by a period of somnolence. He was taken to a nearby hospital where he was lethargic, responding only to noxious stimuli. There he had several generalized seizures that were treated with lorazepam. He was noted to have very poor respiratory effort. An arterial blood gas obtained at that time revealed pH 6.9, PaCO2 146 mmHg, PaO2 311 mmHg, and base deficit of 6.4 mmol/L. The patient was transferred to our institution after endotracheal intubation. There was no history of trauma or ingestion. Additional history revealed that he required evaluation by his primary physician for irritability and poor appetite approximately 2 weeks prior to admission but was otherwise well.
MEDICAL HISTORY
The patient was a full term 3100 g product of an uncomplicated pregnancy. He had speech delay and a history of pica. He received mineral oil periodically during the past 2 months due to constipation but he did not require any other medication. His immunization status was not known. There was no family history of mental retardation or seizures.
PHYSICAL EXAMINATION
T 37.5°C; RR 10/min; HR 110 bpm; BP 130/80 mmHg
Height 25th percentile (estimated); Weight 50th percentile
On examination, the patient was sedated, endotracheally intubated, and minimally responsive to stimulation. His pupils were sluggishly reactive. The optic discs were sharp and there was no papilledema. The left tympanic membrane was mildly erythematous without bulging or retraction. The oropharynx was moist. The neck was supple. Heart sounds and femoral pulses were normal. Auscultation of the lungs revealed symmetric air entry without wheezing or rales. The Glasgow coma score was 7. His gag reflex was intact. Deep tendon reflexes were 3+ but symmetric. There was sustained left ankle clonus and toes were upgoing on Babinski.
DIAGNOSTIC STUDIES
The complete blood count revealed a WBC count of 11 300 cells/mm3 (74% segmented neutrophils, 20% lymphocytes, 5% monocytes, and 1% eosinophils), a hemoglobin of 6.6 g/dL, and 473 000 platelets/mm3. The reticulocyte count was 5.1%. Serum electrolytes and calcium were normal. The blood urea nitrogen was 15 mg/dL and creatinine 0.3 mg/dL. Serum glucose was 170 mg/dL. Serum alanine and aspartate aminotransferases were 83 U/L and 118 U/L, respectively. Ammonia was mildly elevated at 64 mcg/dL. Urinalysis revealed moderate amounts (2+) of glucose and protein, 5-10 WBCs/hpf, 0 RBCs/hpf, and no ketones. Head CT showed diffuse cerebral edema and decreased gray/white differentiation but no masses or intracranial hemorrhage. Opening pressure measured during lumbar puncture was 46 mm H2O. Cerebrospinal fluid analysis revealed 15 WBCs/mm3 and 15 RBCs/mm3. CSF glucose was 85 mg/dL and protein was 42 mg/dL. No bacteria were seen on Gram stain. Urine and serum toxicology screens were negative.
COURSE OF ILLNESS
Electroencephalogram showed paroxysmal epileptiform activity with generalized slowing of the background electrical activity. The seizures were controlled with phenytoin infusion. Examination of the abdominal radiograph suggested a diagnosis (Figure 19-5).
FIGURE 19-5. Abdominal radiograph
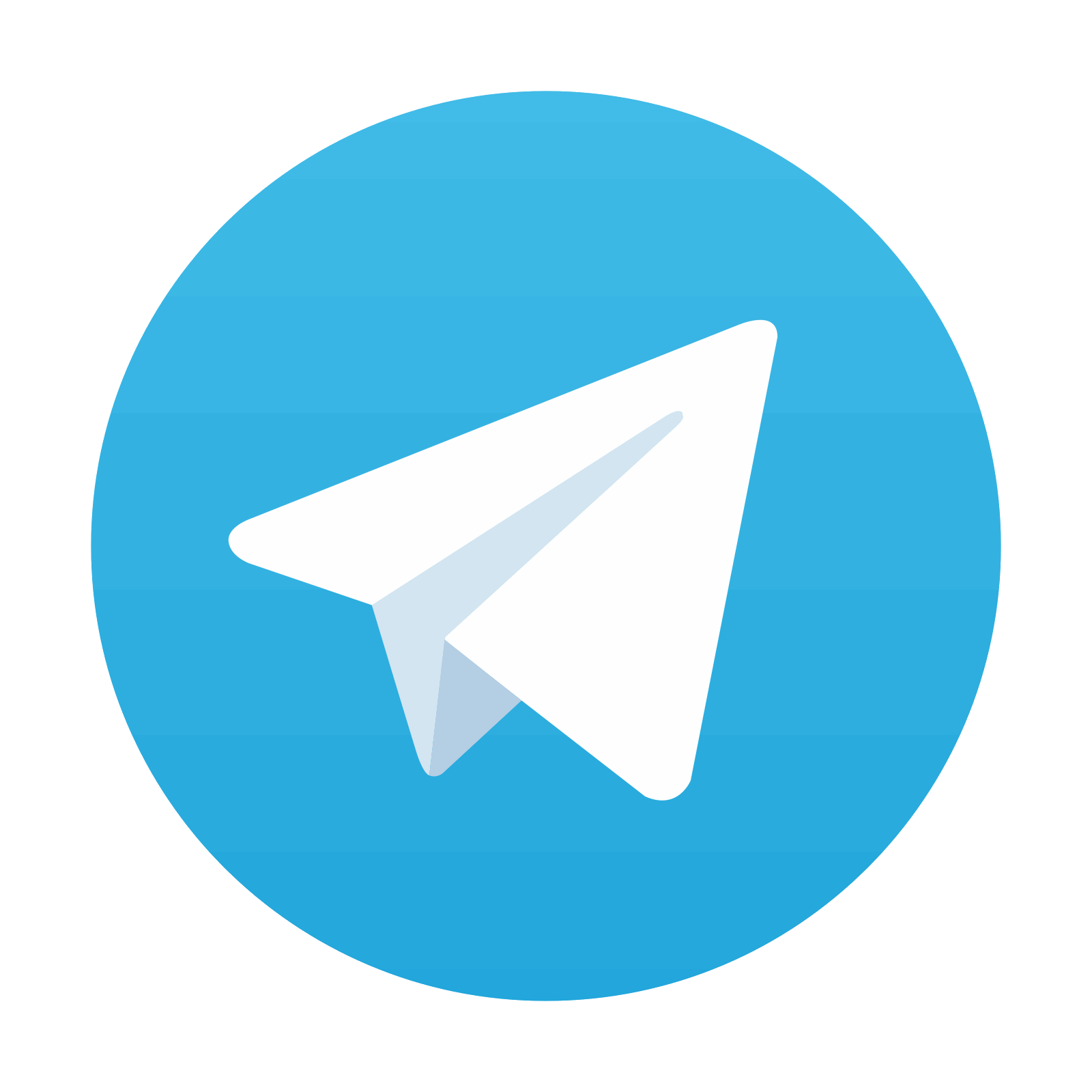
Stay updated, free articles. Join our Telegram channel

Full access? Get Clinical Tree
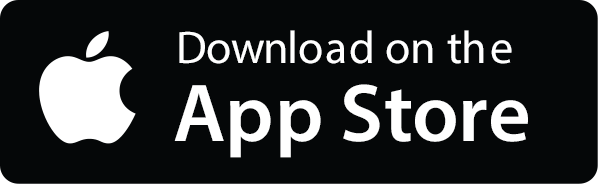
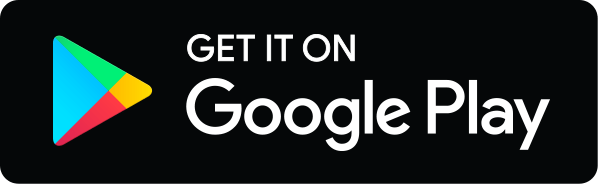