Fig. 15.1
Assessment, complementary interventions, pharmacological treatment, and effective implementation fit together like puzzle pieces for the effective management of pain in neonates
Assessment of Distress and Pain in Neonates
Limitations of Assessment of Distress and Pain in Neonates
Although this is still an area of active research, there are at present no easily applied, widely accepted, uniform techniques to assess either pain or distress in neonates. The gold standard of pain assessment (i.e., verbal report) cannot be used in preverbal patients: neonates can only display their distress or pain. It is up to the caregiver to read and recognize these signs [3, 21]. To structure this evaluation and to make this more objective, pain assessment tools have been constructed. However, assessing pain or distress in neonates remains one of the most challenging issues that caregivers, clinical researchers, and parents have to address. In the absence of a universally accepted, valid, reliable, and bedside-useful single biologic measure, we need to rely on pain assessment tools. Such assessment techniques are based on behavioral observations and/or physiological and hormonal measurements. In general, multidimensional assessment tools (i.e., both behavioral and physiological items) are used. Pain assessment tools that quantify pain-related behavior include but are not limited to muscle tone, facial expression, position of the eyebrows and mouth, crying, muscular activity, or consolability. Table 15.1 provides a list of commonly used multidimensional pain scales in neonates [22–30]. (Refer to Chaps. 5, 16, 17, and 20.)
PIPP [22] | PIPP = Premature Infant Pain Profile (Stevens et al. 1996). Procedural pain score. Indicators assessed are gestational age, behavioral state, heart rate, saturation, brow bulge, eye squeeze, and nasolabial furrow |
DAN [23] | DAN = Douleur Aiguë du Nouveau Né (Carbajal et al. 2005). Procedural pain score. Indicators assessed are facial expression, limb movement, vocalizations, and attempts to vocalization |
MBPS [24] | Modified Behavioral Pain Scale (Taddio et al. 1995). Procedural pain score. Indicators assessed are facial expression, cry, and body movements |
COMFORT [25] | (van Dijk 2000). Prolonged pain, including postoperative pain. Alertness, calmness/agitation, respiratory response, crying (only in non-ventilated cases), physical movement, muscle tone, facial tension (initially behavioral and physiological measures) |
COMFORT-neo [26] | (van Dijk 2009). Prolonged pain, adapted from the COMFORT score. Similar to the COMFORT score, seven behavioral items are scored, but muscular tone is scored based on observations (clenched toes/fists), while “no movement” was converted to “no or minor movement” to adapt for specific characteristics of neonates. One of the behavioral items is either crying (in non-ventilated cases) or respiratory response (in ventilated cases) |
CRIES [27] | CRIES (Krechel et al. 1995) = Crying, requires increased oxygen, increased vital signs, expression, and sleeplessness. Prolonged pain, including postoperative pain |
FLACC [28] | FLACC (Manworren et al. 2003) = Face, Legs, Activity, Cry, Consolability. Prolonged pain, including postoperative pain |
N-PASS [29] | Neonatal Pain, Agitation, and Sedation Scale (Hummel et al. 2010). Procedural and prolonged pain, including ventilated or postoperative. Indicators assessed are crying/irritability, behavior state, facial expression, extremities (tone), and vital signs (heart rate, respiratory rate, blood pressure, oxygen saturation) |
NIPS [30] | Neonatal Infant Pain Scale (Lawrence et al. 1993). Procedural pain. Indicators assessed are facial expression, cry, breathing patterns, arm movements, leg movements, and state of arousal |
Major limitations of pain scale are the impact of maturation and disease status on these indicators. In general, severe illness or immaturity will result in a less robust expression. In addition, these indicators have a limited specificity and even sensitivity for pain [31]. Distress or agitation (e.g., hunger, cold, wet diaper) will also result in similar behavioral responses, while Slater et al. nicely illustrated that there is a difference between nociception and pain expression (facial nonresponders) in neonates who underwent heel lancing. Pain assessment tools focus on aspects of pain expression, not necessary equal to or reflecting nociception [31, 32]. Finally, most of these assessment tools have been validated in a context of acute procedural pain, and may be less effective to unveil either acute persistent or chronic pain in neonates. Since most research focuses primarily on acute pain, in clinical practice, there remains the challenge of assessing prolonged and/or persisting pain [21].
Research can potentially provide more sophisticated measurement tools such as bispectral index (BIS) monitor, near-infrared spectroscopy (NIRS), or skin conductance to quantify sedation or pain in neonates [33]. BIS is a multifactorial tool derived from electroencephalographic findings and quantifies sedation, but has not been validated in infants in the first year of life. NIRS provides information on regional cerebral blood flow and oxygen extraction [33, 34]. However, this is only a surrogate marker for either sedation or pain. Skin conductance can be influenced by sweat glands and may hereby reflect autonomic activation, but in neonates also relates to differences in humidity of the incubator and maturational changes. Until such equipment becomes available following validation, we need to rely on clinical assessment tools [35, 36].
Despite the limitations discussed, there has been an extremely fast growth in the number of clinical assessment tools to quantify pain in neonates [21]. This proliferative growth likely reflects the dilemma related to the current absence of a universally accepted, valid, reliable, and bedside-useful single biologic measure. In the neonatal clinical setting, we suggest that the Premature Infant Pain Profile (PIPP) [22], the Douleur Aiguë du Nouveau-né (DAN/EDIN) score [23], and the COMFORT score [25, 26] are the most commonly used pain assessment tools. The Modified Behavioral Pain Scale (MBPS) has also been frequently used to assess pain expression in young infants [24]. Table 15.1 provides an overview of the variables included in these specific pain scores [22–30].
Despite the name, the PIPP score has been developed to measure procedural pain in both preterm and term neonates, but does consider gestational age (≥36, 32–35, 28–31, or <28 weeks, respectively) as one of the indicators to quantify the pain expression, hereby reflecting the fact that pain expression is less robust in more immature preterm infants. The PIPP score is based on seven indicators—three behavioral (all facial actions: brow bulge, eye squeeze, nasolabial furrow), two contextual (age, behavioral state), and two physiological (heart rate, oxygen saturation)—each with a four-point scale, resulting in a range of 0–28. The behavioral state is classified based on 15 s of observations, while (changes in) heart rate, oxygen saturation, brow bulge, eye squeeze, and nasolabial furrow are observed in a 30-s time interval [22]. The Douleur Aiguë du Nouveau-né (DAN/EDIN) score is a multidimensional behavioral pain assessment tool, initially developed to assess procedural pain in preterm and term neonates without a priori differentiation between both subpopulations [23]. It hereby combines issues related to facial expression (0–4 points), limb movements (0–3 points), and vocal expression (0–3 points) characteristics, resulting in a maximum total DAN score of 10. The scoring on vocal expression does contain specific instructions for intubated newborn infants.
The reliability and validity of the COMFORT scale as a postoperative pain instrument has been assessed in 158 neonates and toddlers following major abdominal or thoracic surgery [25]. Trained nurses rated the children’s pain at 3, 6, and 9 h postoperatively in the Pediatric Surgical Intensive Care Unit using the COMFORT and a Visual Analogue Scale (VAS) for pain. Inter-rater reliability of the COMFORT items proved to be good for all items with the exception of the item “respiratory response,” which was moderate (Kappa 0.54). Further analysis showed that the structure of the COMFORT data was best represented by three latent variables: COMFORT “behavior” with loadings from the behavioral items (alertness, calmness, respiratory response/crying, physical movement, muscle tone, and facial tension) and separate latent variables for “heart rate baseline” (HR) and “mean arterial blood pressure baseline” (MAP). Factor loadings of the items were invariant across time, indicating stability of the structure. The latent variables COMFORT “behavior” and VAS pain were highly interrelated, indicating congruent validity. Stability of COMFORT “behavior” and VAS pain was moderate [25]. Because prolonged pain in neonates remains a challenge, a modified version of the COMFORT-Behavior scale (COMFORT-neo) for its psychometric qualities in the NICU setting has more recently been assessed [26]. In a clinical observational study, nurses assessed patients with COMFORT-neo and Numeric Rating Scales (NRS) for pain and distress, respectively. Based on almost 3,600 triple ratings in 286 neonates, inter-rater reliability turned out to be good. Concurrent validity was demonstrated by adequate and good correlations, respectively, with NRS-pain and NRS-distress (r = 0.52, 95 % confidence interval 0.44–0.59) and (r = 0.70, 95 % confidence interval 0.64–0.75, respectively). COMFORT-neo cutoff scores of 14 or higher (score range is 6–30) had good sensitivity and specificity (0.81 and 0.90, respectively) using NRS-pain or NRS-distress scores of 4 or higher as criterion [26]. The Modified Behavioral Pain Scale (MBPS) quantifies facial expression, limb movements, and vocalizations or attempt at vocalizations and has mainly been developed and applied for procedural pain expression in young infants (2–6 months) (e.g., immunizations) [24].
Implementation of Assessment
Among others, the American Academy of Pediatrics states that ongoing assessment of pain is essential for adequate pain treatment. Despite this, there remains a gap between the available knowledge and the effective implementation of pain assessment in neonates [37]. The use of pain monitoring scales and the subsequent pain management undergo evaluation as part of the European project in neonates. To further illustrate the relevance of such epidemiological studies, we refer to three recently published observational studies from Italy, Australia, and the Netherlands [14, 19, 38, 39]. A recent report from Italian NICUs suggest that systematic assessment of pain is routinely applied in only 20 % of neonates on mechanical ventilation, in 12 % of neonates on nasal CPAP, and only 14 % of neonates in a postoperative setting [14, 19]. Similar observations were reported from Australia, based on data available from 196 hospitals. A clinical practice guideline informed the management of neonatal pain in 76 (39 %) of the hospitals. There was wide variation in their use between the states and a significantly higher use of such a guideline in higher-level care units. A pain assessment tool was only used in 21 (11 %) of the units with greater use in the higher-level care NICUs (50 %) and surgical NICUs (80 %). Awareness of breastfeeding for procedural pain was reported by 90 % of the 196 respondents, while 78 % reported that it was actually used. Awareness of sucrose for procedural pain was lower than breastfeeding at 79 %, with 53 % reporting that they used sucrose in their unit. Overall, 89 % of the respondents reported that either breastfeeding or sucrose was used for the management of procedural pain in their units [38]. Finally, Ceelie et al. assessed compliance to a pain management protocol in a cohort of 200 postoperative infants in the Rotterdam unit [39]. A mean of 11 assessments in the first 72 h postoperatively per patient had been recorded. A total of 2,103 pain assessments were retrieved, of which 1,675 (79.7 %) suggested comfort. Compliance to the protocol (reassessment and correct medication) was provided in 66 (15.4 %) of the 428 assessments suggesting pain or distress. Consequently, the authors concluded that the postoperative pain protocol applied in their ICU appeared to be effective, while full compliance to the protocol remained only marginal, possibly leading to undertreatment of pain.
Take-Home Messages on Pain Assessment
Assessment of pain in neonates is an essential part of effective pain treatment [21].
Multidimensional pain scales such as the PIPP, DAN, and COMFORT (neo) are the most commonly used pain assessment tools (Table 15.1) [22–30].
Currently available assessment tools are suboptimal, since they are based on pain expression, not necessary reflecting nociception [31, 32].
Not the assessment, but the implementation of assessment is the problem: strategies to optimize the implementation of systematic objective assessment of pain are urgently needed [37].
Preventive Strategies
Several complimentary interventions as well as adaptations of procedural techniques may be used to prevent pain and stress in newborn infants. In this way, such interventions may either reduce the need for pharmacological interventions or improve their effectiveness. Such strategies include light and noise reduction, nesting or swaddling, rationalizing and minimizing patient handling (e.g., preserving free periods for sleep, avoid consecutive blood sampling, clustered care), consider the use of central venous catheters instead of multiple peripheral perfusions, individualized monitoring techniques (vital signs registration, blood pressure measurement interval), tailoring nursing techniques (e.g., frequency endotracheal suctioning, skin and wound care, tape and wound dressing), and promoting skin-to-skin contact between the newborn and its parents. The growing body of evidence on specific non-pharmacological (complimentary) interventions is discussed later. We here would like to stress the relevance to consider methodological, procedural aspects as a potential powerful tool to reduce the need for analgo-sedation. This is illustrated by endotracheal suctioning and venous blood sampling.
Endotracheal suctioning is a painful and stressful procedure, commonly associated with pronounced fluctuations in vital signs in ventilated newborn infants. Cordero et al. compared two endotracheal suctioning frequencies in preterm neonates and concluded that there was no benefit of systematic, routine suctioning compared to suctioning as needed [40]. Based on these findings, an evidence-based protocol whereby ventilated newborn infants were suctioned only as needed based on clinical indicators was developed. This protocol was subsequently introduced as part of the collaborative quality improvement initiative earlier mentioned [41] and resulted in a significant decrease in the number of procedures performed. Four-handed care to facilitate containment during endotracheal suctioning was also associated with a decrease in stress and defense behavior and an increase in self-regulatory behavior [42]. Besides frequency of endotracheal suctioning or complimentary interventions, technical issues such as disconnection or deep versus shallow endotracheal suctioning have been evaluated in two recent Cochrane meta-analyses [43, 44]. Based on observations in 252 infants and using a crossover design in which suctioning with or without disconnection was compared, it was concluded that suctioning without disconnection resulted in a reduction in episodes of hypoxia (RR 0.48), and fewer infants experienced episodes where the transcutaneous partial pressure of oxygen (TcPO2) decreased by >10 % (RR 0.39). Endotracheal suctioning without disconnection resulted in a more limited change in heart rate (weighted mean difference 6.77) and a reduction in the number of infants experiencing a decrease in heart rate by >10 % (RR 0.61). The number of infants having episodes of bradycardia was also reduced during closed suctioning (typical RR 0.38). There is evidence to suggest suctioning without disconnection from the ventilator improves the short-term outcomes when focusing on vital signs, likely reflecting reduced stress response [43]. In contrast, there is no evidence on the benefits or risks of deep versus shallow suctioning of endotracheal tubes in ventilated neonates [44].
Venous blood sampling is a commonly performed procedure in neonates. Besides complementary interventions such as nonnutritive sucking, sucrose, or containment, the technique used for blood sampling is also of relevance as illustrated in two studies in 120 and 100 healthy term neonates, respectively. In the study of Larsson et al. venipuncture was compared to a small or large lancet, respectively, in neonates who underwent testing for phenylketonuria. Successful sampling with only one skin puncture was successful in 86, 19, and 40 % of the cases, while median time to finalize collection was 191, 419, and 279 s, respectively. This also resulted in lower pain scores in the venipuncture group (Neonatal Facial Coding Score [NFCS]) (247) compared to both heel lancing techniques (333 and 460, respectively) [45]. Similar observations were reported by Ogawa et al. [46]. A population of 100 healthy term neonates were randomly allocated to 1 of 4 groups (venipuncture versus heel lancing, oral sucrose versus water). Using this design, the NFCS was significantly lower in the venipuncture group (230 versus 580). The lancing group with sucrose even still had higher scores compared to the venipuncture without sucrose (470 versus 230).
Take-Home Messages
Complementary Interventions
Increased awareness of a persistent high number of painful procedures routinely performed in neonates during their stay in the unit, combined with concerns regarding potential adverse effects of pharmacological agents, and the desire to actively involve parents in the care of their newborns resulted in a surge and evaluation of alternative, non-pharmacological interventions for acute, procedural pain in neonates [47, 48].
Non-pharmacological, such as environmental and behavioral, interventions have a wide applicability for neonatal pain management alone, or in combination with pharmacological treatments. These interventions are not necessarily substitutes or alternatives for pharmacological interventions but are complimentary. Non-pharmacological interventions can reduce neonatal pain indirectly by reducing the total amount of noxious stimuli to which infants are exposed and directly by blocking nociceptive transduction or transmission or activation of descending inhibitory pathways or by activating attention and arousal systems that modulate pain. In neonates, nonnutritive sucking, including sucrose, glucose, or human milk, swaddling and containment procedures, sensory stimulation, and the kangaroo method can be considered as complementary interventions.
Nonnutritive Sucking, Sucrose, Glucose, and Human Milk
There is limited evidence to support the use of nonnutritive sucking in preterm and high-risk full-term infants as an intervention to promote behavioral outcomes and gastrointestinal function or feeding tolerance, but it has been linked to a reduced length of hospital stay and improved pain management. Nonnutritive sucking in preterm and high-risk full-term infants does not appear to have any short-term negative effects, but data on long-term outcome in high-risk full-term and preterm infants are not available. Based on the available results, it seems reasonable to utilize pacifiers for pain management in high-risk full-term and preterm infants [49, 50].
The most extensively evaluated non-pharmacological intervention for procedural pain relief in neonates is the oral administration of sucrose (12–24 %), glucose (30 %), or mother’s milk, either or not combined with nonnutritive sucking (pacifier). It is believed that the effects of sucrose and nonnutritive sucking are mediated by both endogenous opioid and non-opioid systems. There is meta-analytical evidence in support of the use of oral administration of sucrose 24 %, glucose 30 %, or mother’s milk in combination with a pacifier shortly before a painful procedure (e.g., blood sampling, nasogastric tube placement, immunization/vaccination) as an effective tool for procedural analgesia in neonates [51–56]. The observations on the use of sucrose during heel lancing hereby are much more common compared to other interventions or procedures.
Consequently, it became the most frequently applied intervention for procedural analgesia in neonates and, to a more limited extent, in infants. To make this more effective, this should be combined with the use of a pacifier, and the sweet solution should be administered on the tongue shortly (2 min) before the initiation of the procedure. This time interval is thought to coincide with the endogenous opioid release. When compared with local analgesia/EMLA or systemic acetaminophen (paracetamol) or morphine, glucose/sucrose results in the most prominent decrease in pain scores [51–56]. More moderate positive results were obtained during immunization in infancy (2–6 months), resulting in the guidelines to use sweet solution with a pacifier (or other facility to maintain suctioning) only up to the age of 4, max 6 months [57].
All these studies used neonatal pain scores to quantify pain expression, assuming that this also reflects differences in nociception. In the preverbal setting, the gold standard of pain assessment (i.e., verbal rapport of the individual patient) cannot be applied. The neonate is unable to say and can only show (express) his/her distress or pain. Consequently, it is up to the caregiver to recognize (read) these signs or to look for the absence of signs of comfort. To read these signs in a structured way, several sedation or pain scales have been developed and validated. In general, all currently clinical available tools focus on aspects of pain behavior or expression (e.g., motor activity, facial expression, motor tone, vital signs), not necessary reflecting pain perception or nociception [21–30]. This methodology-related conflict between different methods to assess pain in neonates has recently been illustrated in the paper of Slater et al. on sucrose during heel lancing in neonates [31, 32].
In a randomized controlled setting (sucrose versus water), the authors confirmed the significant decrease in PIPP scores when sucrose was applied. However, when more sophisticated assessment tools (spinal nociceptive reflex withdrawal activity or cortical evoked response, i.e., specific brain activity evoked by one time-locked heel lance with electroencephalography as identified by principal component analysis) were applied, no differences between both groups could be unveiled. We are aware that this study has been criticized on its sample size (insufficiently powered) and methods (electroencephalogram evaluated limited to 0.5 s before up to 1 s after the heel lance), but at least it re-illustrates that pain expression (as assessed by pain scores) is not equal to nociception [31, 32]. At least, the behavioral effect of sucrose can likely be explained by a pain modulation effect, and hereby provides evidence for the presence of pain modulating systems in neonates. In essence, caregivers responsible for neonates and infants are made aware of the fact that early pain experience is one of the covariates of interindividual variability in neurodevelopmental outcome (e.g., pain thresholds, pain- or stress-related behavior, and physiological responses in later life), while Slater et al. illustrated that sucrose or glucose are indeed not perfect as analgesics, and that they are likely in part effective through distraction and in part through endogenous opioid release [31, 32].
Swaddling and Containment Procedures
Van Sleuwen et al. performed a meta-analysis on the available knowledge on the impact of swaddling in excessively crying infants [58]. These authors concluded that swaddled infants arouse less and sleep longer. Preterm infants have shown improved neuromuscular development, less physiological distress, better motor organization, and more self-regulatory ability when they are swaddled. When compared with massage, excessively crying infants cried less if swaddled, and swaddling can soothe pain in infants. It is supportive in cases of neonatal abstinence syndrome and infants with neonatal cerebral lesions. It can be helpful in regulating temperature but can also cause hyperthermia when misapplied. Another possible adverse effect is an increased risk of the development of hip dysplasia, which is related to swaddling with the legs in extension and adduction. In the neonatal intensive care setting, data are somewhat more contradictory. In a meta-analysis, it seems that swaddling has a pain-relieving effect, but it was maintained longer in term compared to preterm neonates [59].
In Table 15.2, we provide an illustrative overview of studies to illustrate the effectiveness of facilitated tucking in (pre)term neonates, either not combined with or compared to other complementary interventions, like oral sucrose, or nonnutritive sucking [56, 60–69]. Methodologically, the majority of these studies were not blinded and applied a crossover type of design, yet order effects are only rarely reported. However, the available evidence points to a modest reduction in pain scores and physiological fluctuations and a faster return to baseline [56, 60–69]. To test the comparative effectiveness of different non-pharmacological pain-relieving interventions, either applied alone or in combination to document potential synergism, effectiveness of oral sucrose, facilitated tucking, or both, a prospective study in 71 preterm (24–32 gestational age) neonates was performed in three NICUs in Switzerland [65]. Facilitated tucking alone was significantly less effective in relieving repeated procedural pain than sucrose 24 % (0.2 mL/kg). However, facilitated tucking in combination with sucrose had an added value in the recovery phase with lower pain scores compared to both single interventions.
Table 15.2
Overview of studies to illustrate the effectiveness of facilitated tucking in preterm neonates, either or not combined with or compared to other complementary interventions (oral sucrose, nonnutritive sucking)
Reference | Study design and results |
---|---|
Liaw et al. [60] | Randomized, controlled crossover trial in 34 preterm (29–37 weeks) neonates to compare nonnutritive sucking to facilitated tucking with routine care on pain response (Premature Infant Pain Profile, PIPP score) after heel lancing. Both facilitated tucking and nonnutritive sucking resulted in a reduced pain response, but nonnutritive sucking was more effective as single intervention |
Liaw et al. [61] | Randomized, controlled trial to assess the impact of nonnutritive sucking, sucrose, and facilitated tucking either alone or combined on infant’s sleep–wake states before, during, and after heel-stick procedures in 110 infants (26.4–37 weeks gestational age). The combination of nonnutritive sucking, sucrose, and facilitated tucking resulted in the best preservation of the infant’s sleep–wake states |
Sundaram et al. [62] | Randomized controlled crossover pilot study in 20 preterm (28–36 weeks) neonates to compare the impact of facilitated tucking to no intervention on the PIPP score 30, 60, 90, and 120 s after the heel stick. Facilitated tucking resulted in significantly lower PIPP scores throughout time (8.8, 7.5, 7.2, 6.6, and 11.2, 10.7, 10.6, and 10.5) |
Hill et al. [63] | Randomized, crossover study in 12 preterm (25–34 weeks) neonates to compare the impact of facilitated tucking to routine care on the stress response (PIPP) during routine nursing assessments. 9/12 infants received a lower PIPP score with facilitated tucking, reflecting the fact that the stress during routine nursing assessment can be reduced by facilitated tucking |
Corff et al. [64] | Randomized, crossover study in 30 preterm (25–35 weeks) neonates to compare the impact of facilitated tucking with routine care on vital signs and sleep disruption following heel lancing. A lower heart rate, a shorter crying time and shorter sleep disruption times were documented during facilitated tucking |
Cignacco et al. [65] | Randomized controlled trial in 71 (24–32 weeks) neonates to assess the effectiveness of sucrose, facilitated tucking, or both on the pain response following heel lancing, using the Bernese Pain Scale for Neonates. Facilitated tucking was less effective compared to sucrose, but combination of both interventions resulted in a further improvement in the recovery phase |
Axelin et al. [66] | Prospective, randomized controlled trial in 20 preterm (24–33 weeks) neonates to assess the impact of facilitated tucking by parents on pain expression (Neonatal Infant Pain Scale, NIPS) and vital signs during endotracheal or pharyngeal suctioning. Facilitated tucking by parents resulted in a lower NIPS (median 3–5) score, and the infant calmed down more quickly (median: 5–17 s) |
Ward-Larson et al. [67] | Prospective, randomized crossover trial in 40 (23–32 weeks) preterm neonates to assess the impact of facilitated tucking (second nurse) to routine nursing on procedural pain (PIPP) related to endotracheal suctioning. PIPP scores during facilitated tucking were significantly lower compared to routine nursing care |
Fearon et al. [68] | The responses of preterm neonates to swaddling after a heel lance were quantified in 15 preterm neonates after blood sampling. Preterm infants aged 31 weeks or older showed protracted behavioral disturbances that were reduced by the use of swaddling. In younger infants, there was a return to behavioral patterns irrespective of the treatment conditions |
Gabriel et al. [56] | NIPS scores in 136 healthy newborns. Skin-to-skin contact (SSC), combined with either sucrose (Sucr) of breastfeeding (BF) during heel prick. BF in addition to SSC provides superior analgesia to other kinds of non-pharmacological analgesia |
Johnston et al. [69] | Therapeutic touch given immediately before and after heel lance in extreme preterm (<30 weeks) neonates in a randomized, blinded approach was ineffective (PIPP score) to reduce pain expression during and after heel lance |
Multisensorial Stimulation and Sensorial Saturation
Sensorial saturation is a multisensorial stimulation consisting of simultaneous delicate tactile, gustative, auditory, and visual stimuli. This procedure consists of simultaneously attracting the infant’s attention by massaging the infant’s face; speaking to the infant gently, but firmly; and instilling a sweet solution on the infant’s tongue. Non-painful stimulation, by engaging a number of channels (i.e., auditory, tactile, visual, olfactory, vestibular, gustatory), is thought to compete with the painful sensory input. In a recent systematic review on this topic, ten studies were retrieved that evaluated at least partial sensorial saturation [47]. Based on the evidence collected, the use of an oral solution alone is less effective than sensorial saturation, while sensorial stimulation without oral sweet solution is ineffective. Consequently, it was concluded that sensorial saturation can be used for all newborns undergoing blood samples or other minor painful procedures. It is more effective than oral sugar alone and promotes interaction between the caregiver and infant [47, 56, 61, 65].
From Evidence to Practice: The Implementation Issue
Despite the available knowledge, deficits in the clinical management of pain remains. One reason is the gap between research evidence and translation of this knowledge into the clinical setting [70]. This is particularly true for non-pharmacological pain-relieving methods. Effective performance of some of these methods requires additional staffing and time. Although “facilitated tucking” is described as an efficient method for acute pain relief, the clinical facilitators required to successfully implement such a resource-consuming intervention remain unclear. In essence, the increased economic costs and organizational impact need to be balanced against possible (long-term) health gain benefits. Following the availability of studies on effectiveness of a given intervention, the subsequent implementation in the absence of allocation of appropriate means could provide caregivers with new knowledge and the burden not to be able to perform this good clinical practice. A report on the limited compliance with pain management guidelines for heel blood sampling in European NICUs confirms this gap between the available knowledge, the guidelines, and the bedside practices [71].
Another relevant question is how to integrate parents into these complementary interventions through either kangaroo care or facilitated tucking. Kangaroo care is defined as holding the newborn skin-to-skin against the mother’s body with or without additional covering and in an upright 40–60° angle. Kangaroo care was documented to have some effect on pain expression (PIPP score) during heel lancing [72]. Similar, skin-to-skin contact, containment, and maternal voice resulted in a reduction in duration of crying or grimacing during and following heel lancing. However, the Johnston study had a 40 % refusal rate, indicating that not all parents are comfortable with these procedures and their contributions to the pain relief [72].
In two consecutive studies on parental-facilitated tucking, Axelin et al. first illustrated that facilitated tucking by parents is indeed effective (NIPS score: 3 [2–6] versus 5 [2–7]) and safe in preterm neonates that undergo endotracheal suctioning [66]. This was followed by an evaluation of the parental willingness to actively participate in their preterm infants’ pain care through parental-facilitated tucking. The willingness to participate related to their internalized involvement, i.e., to what extent do the parents consider themselves skilled enough to take care of this responsibility [73].
Take-Home Messages
Sucrose 24 %, glucose 30 %, or mother’s milk, all, respectively, combined with a pacifier, are the most effective analgo-distractive techniques currently available for procedural pain relief in neonates. There is evidence in support of other non-pharmacological pain-relieving methods (e.g., swaddling, containment, multisensorial stimulation) [53–55, 61, 65].
The sweet solution should be administered on the tongue shortly (2 min) before the initiation of the procedure. The illustration that this might not be as effective as anticipated should only enforce us to avoid procedural pain as much as possible [31, 32].
Pharmacological Interventions
Pharmacological interventions focus either on analgesia, sedation, or both. We will first discuss agents commonly administered to attain analgesia with increasing potency (topical and local anesthesia, acetaminophen/paracetamol, morphine and fentanyl, remifentanil), followed by sedatives (benzodiazepines, chloral hydrate, propofol, dexmedetomidine), or both (ketamine, inhalational agents).
Topical and Local Anesthesia
Local anesthetics of the amide group (Ia) have effects on the central nervous system (depression or activation), peripheral nervous system (decreased conduction), and cardiovascular system (shortening action potential). Elimination is either through primary renal elimination or through hepatic metabolic clearance. Hepatic metabolism does result in intermediate metabolites, and these metabolites have also been linked to some of the observed toxic side effects [74]. However, the extent of the metabolic clearance compared to the primary renal elimination in neonates is unknown. Besides analgesia, there is also an increasing experience with lidocaine to treat neonatal seizures. However, this specific indication is beyond the scope of this chapter. In essence, there remains a delicate balance between effects and potential side effects in neonates with use of local anesthesia [74].
Topical local anesthetics are available in various forms such as a lidocaine ointment or gel, and amethocaine/tetracaine cream, but the Eutectic Mixture of Local Anesthetics (EMLA) as a cream, containing both 2.5 % of lidocaine and 2.5 % of prilocaine, is most commonly used and evaluated. We will first discuss efficacy data, followed by some observations on toxicity. In general, it provides good superficial (skin) anesthesia for 1–2 h when applied under an occlusive dressing. Application should be done about 1 h before the skin-breaking procedure. In neonates, this has been evaluated for heel lancing, venipuncture, lumbar puncture, and circumcision, but data for skin-breaking procedures are to a certain extent conflicting.
In a meta-analysis on heel lancing, there was no significant benefit of EMLA for any of the outcome measures used to assess pain (i.e., behavioral pain scores, infant crying, heart rate, blood pressure, respiratory rate, oxygenation) [75]. For venipuncture, infants treated with EMLA had significantly lower heart rates and crying duration compared with infants treated with a placebo. However, oral sucrose 24 % [76] or glucose 30 % [77] in combination with a pacifier seems to be more effective in reducing pain expression during venipuncture than EMLA application. However, the combination of sucrose and EMLA cream revealed a higher analgesic effect than sucrose 24 % alone during venipuncture in preterm infants [78]. Similar effects have been documented for pain relief during percutaneous venous catheter placement (heart rate, respiratory rate) and arterial puncture (behavioral pain score).
For lumbar puncture, we are aware of two studies with conflicting results. Kaur et al. provided evidence that supports the concept that EMLA is effective in reducing pain associated with needle insertion and withdrawal during lumbar puncture in newborn infants [79]. Unfortunately, compared with baseline observations, all newborn infants experienced pain as evidenced by increased heart rate, decreased oxygen saturation level, and total behavioral score [79]. In contrast, EMLA did not reduce physiological changes or behavioral pain scores in another RCT in neonates (>34 weeks GA) undergoing lumbar puncture [80]. Based on the available evidence, topical anesthetics may blunt the physiological markers of pain, but this does not result in a pain-free procedure [81].
Similar trends on limited to moderate effectiveness have been observed to treat pain during circumcision. EMLA cream (1–2 g) can be applied to the distal half of the penis with subsequent occlusive dressing 60–90 min before circumcision is performed. It is important to minimize systemic absorption by removing the cream just before the start of the surgical procedure. The first data on the efficacy and safety of this approach have been described by Taddio et al. [82]. Using an RCT approach, 38 neonates were treated with EMLA. Compared to 30 neonates in the placebo arm, the neonates in the lidocaine–prilocaine group had less facial activity, spent less time crying, and had smaller increases in heart rate than the neonates in the placebo group. Blood methemoglobin concentrations (expressed as a percentage of the hemoglobin concentration) were similar (1.3 %) in both groups. Lidocaine and prilocaine were detected in plasma in 61 and 55 % of the infants treated with lidocaine–prilocaine cream, respectively. However, when compared to other regional analgesic interventions (ring block, dorsal penile block), the ring block was equally effective through all stages of the circumcision, whereas dorsal penile nerve block and EMLA were less effective during foreskin separation and incision; methemoglobin levels were highest in the EMLA group, although not a single newborn required treatment [83].
Pretreatment with EMLA decreases infant pain related to routine vaccinations, but the application of these data is limited to healthy infants [84]. The combined use of EMLA and glucose 30 % was proven to be effective when compared to placebo, while combining sucrose, oral tactile stimulation, and parental holding was also associated with significantly reduced crying in infants receiving multiple immunization injections [85].
Besides EMLA cream, sprays (4 % lidocaine, max 0.1 mL/kg) or gel (2 %, max 0.3 mL/kg) for mucosal topical anesthesia (2) or local injection of lidocaine (up to 3 mg/kg max, equal to 0.3 of the 1 % formulation) are also commonly used. Data in infants documented that nebulized lidocaine is not effective to reduce the pain response to nasogastric tube placement [86]. In contrast, lingual 24 % sucrose is effective in reducing the behavioral and physiological pain response to nasogastric tube insertion in preterm infants [87]. We could not find data on the effects of mucosal spray to facilitate bronchoscopy or gastroscopy in neonates.
Besides the overall limited benefit or add-on effect of lidocaine, there is a relevant concern about toxicity in neonates. Different case reports and case series on the association of EMLA application and seizures (lidocaine mediated) and/or methemoglobinemia (prilocaine mediated) have been described. Newborns are at higher risk to develop methemoglobinemia because of reduced NADH-dependent methemoglobin reductase. The same limited effect/potential side effect balance can be constructed for tetracaine. Some of the available reports on limited tetracaine effects in newborns were summarized in Table 15.3 [88–92]. However, contact dermatitis and cardiac arrhythmia has been described in neonates.
Table 15.3
Reported papers on the analgesic effects of tetracaine/amethocaine in neonates (type of procedure highlighted)
Reference | Study design and results |
---|---|
Shah et al. [88] | Randomized, double-blind, placebo-controlled trial, intramuscular injection (vitamin K) in 110 term neonates, topical amethocaine gel 4 %. There were no differences in crying duration, in pain score and only the latency to cry was somewhat longer in the treated group. Topical amethocaine gel 4 % was ineffective in reducing pain intramuscular injection of vitamin K in full-term neonates |
Jain A et al. [89] | Randomized, double-blind, placebo-controlled trial in 40 (pre)term neonates during venipuncture. Topical amethocaine provided effective pain relief (crying, neonatal facial coding system) during venipuncture in the newborn when used as single technique for analgesia |
Lemyre et al. [90] | Randomized, double-blind, placebo-controlled trial in 142 preterm (from 24 weeks onward) infants during venipuncture. Tetracaine did not significantly decrease procedural pain in infants undergoing a venipuncture, when used in combination with routine sucrose administration |
Lemyre et al. [91] | Randomized, double-blind, placebo-controlled trial in 54 preterm neonates on the add-on effect of tetracaine gel in addition to sucrose to treat procedural pain related to peripherally inserted central catheter (PICC) placement. Tetracaine 4 % when applied for 30 min was not beneficial in decreasing procedural pain associated with a PICC in very small infants |
Jain et al. [92] | Randomized, double-blind, placebo-controlled trial in 60 (pre)term neonates during heel prick blood sampling. Topical amethocaine gel does not have a clinically important effect on the pain of heel prick blood sampling. Its use for this purpose cannot therefore be recommended |
Take-Home Messages
The overall evidence suggests a modest to moderate effect on procedural pain in neonates. This means that for most of the procedures, topical anesthesia should be considered as part of a multimodal analgesia [74].
There remains a concern on absorption-related toxicity (seizures, methemoglobinemia). Maximal doses should be adhered to; absorption is more likely in the presence of disrupted skin. When applied for circumcision, EMLA should be removed just before the start of the surgical intervention [74].
Propofol
Propofol (2,6 di-isopropylphenol) is a highly lipophilic compound that exhibits rapid distribution from the blood to the subcutaneous fat and the central nervous system compartments with subsequent redistribution and metabolic clearance. It is considered to be a short-acting anesthetic (not an analgesic) that is both rapid in its onset and short in duration after cessation [93]. Because of these pharmacokinetic and dynamic characteristics, propofol became a frequently administered drug for induction and/or maintenance of anesthesia in children and, more recently, in neonates. However, continuous administration may result in serious, sometimes lethal metabolic complications (propofol infusion syndrome) in children and has more recently also been described in a preterm newborn following inadvertent dosing during surgery [94]. This is of relevance, since it took about 15 years of unlicensed, off-label administration before this serious side effect, and its risk factors in pediatric patients were unveiled.
Because propofol is a water-insoluble phenolic compound, propofol clearance is exclusively through metabolic clearance. In adults, metabolism is mainly through glucuronidation. Since glucuronidation capacity in neonates displays important ontogeny, pharmacokinetics in this specific population are of utmost relevance. Data on propofol pharmacokinetics in neonates are available [95]. Standardized propofol clearance at 38 weeks postmenstrual age (PMA) (CLstd) was 0.029 L/min. A fixed value in neonates with a postnatal age (PNA) of ≥10 days further improved the model and resulted in the equation:
When compared to adults (1.91 L/min−1) following an IV bolus, the difference in clearance is impressive (65-fold) [95]. The complex interplay between size and maturation results in an overall low propofol clearance capacity at birth (estimated to be 0.029 L/min at 38 weeks PMA) with a subsequent PNA- and PMA-related increase. Consequently, both preterm and term neonates in the first week of postnatal life have an increased risk for accumulation following intermittent bolus or continuous administration of propofol due to the reduced clearing capacity. Secondly, there is still extensive unexplained variability in neonates after introducing PMA and PNA as covariates, making prediction in neonates more difficult [95].
![$$ \left[{\mathrm{CL}}_{\mathrm{std}}.\;\left(\mathrm{P}\mathrm{M}\mathrm{A}/38\right)11.5+0.03\right]\;\mathrm{l}/ \min\;\mathrm{f}\mathrm{o}\mathrm{r}\;\mathrm{neonates}\ge 10\;\mathrm{days} $$](/wp-content/uploads/2016/11/A159425_2_En_15_Chapter_Equa.gif)
Pharmacodynamics of propofol have been described, with specific emphasis on the (side) effects of propofol during endotracheal intubation (Table 15.4) [96–100]. Ghanta et al. reported on propofol (2.5 mg/kg) pharmacodynamics in 33 preterm neonates during semi-elective endotracheal intubation. Compared to a morphine/atropine/suxamethonium regimen, time until sleep, muscle relaxation, and time to achieve successful intubation were shorter [98]. These short-acting sedative effects were confirmed by others [96–100]. In contrast, however, a significant impact on blood pressure (decrease 20 %) and oxygenation have been reported in term neonates, in neonates with an associated cardiopathy, and in two cohorts of preterm neonates undergoing chest tube removal (n = 20, 3 mg/kg−1) or during INSURE (n = 13, 1 mg/kg−1). We hereby would like to remind the readers that there is an association between fluctuations in blood pressure and intracranial hemorrhage in the first days of postnatal life in preterm neonates [101].
Table 15.4
Summary of the reported studies on the use of propofol to facilitate endotracheal intubation in (pre)term neonates, reflecting the variability in clinical characteristics, outcome criteria, co-medication and doses (1–2.5 mg/kg) evaluated in the different studies
Reference | Study design and results |
---|---|
Welzing et al. [96] | Prospective, observational study on intubating conditions, vital signs, extubation times and outcome in 13 preterm neonates treated with propofol (1 mg/kg) for an INSURE (intubation, surfactant, extubation) procedure. The study was stopped early because of significant cardiovascular side effects expressed as distinct drop in mean blood pressure (mean values = 38 mmHg to 24 mmHg 10 min after propofol exposure). Intubation conditions were reported to be good |
Nauta et al. [97] | Retrospective analysis on trends in arterial blood pressure (invasive) in 21 preterm neonates (28.8, SD 3.5 weeks) exposed to propofol (2 mg/kg), 5/21 co-treated with atropine. The decline in mean arterial blood pressure before and after propofol administration (48–41 mmHg) was not significant, and the proportion of patients with hypotension was similar before and after propofol exposure |
Ghanta et al. [98] | Randomized, open-label controlled trial comparing propofol (2.5 mg/kg) with morphine (100 μ[mu]g/kg)-atropine (10 μ[mu]g/kg)-suxamethonium (2 mg/kg) as induction agents for endotracheal intubation in 63 preterm neonates. There were no differences in vital signs, but trough oxygen saturation was significantly lower in the M-A-S group, and recovery time was shorter in the propofol group [recovery time = return of spontaneous muscle movement] |
Papoff et al. [99] | Pilot study in 21 (pre)term neonates with severe respiratory distress syndrome. Fentanyl (1.5 μ[mu]g/kg) was coadministered with propofol (2 mg/kg over 20 s) and propofol was administered a second time if more than 1 attempt to intubate was needed. A subscore of ≤2 for all items of the Helbo-Hansen score system was qualified to reflect an easy intubation. Intubation was qualified as easy in all cases, intubation at first attempt in 18/21. Oxygen desaturation (all >60 %) was documented in 7/21 cases. These desaturation events were commonly associated with a transient decrease in systemic blood pressure (treated with crystalloids, 10 mL/kg) |
Penido et al. [100] | Double-blinded, randomized controlled trial in 20 preterm (28–34 weeks) neonates, exposed to either propofol (2 mg/kg) or midazolam (0.2 mg/kg). Both (propofol/midazolam) were combined with remifentanil (1 μ[mu]g/kg). No differences in intubation conditions or number of attempts needed were observed |
Because spontaneous respiration can be maintained, propofol (intermittent bolus, 1 mg/kg, combined with topical anesthesia) has been used to facilitate diagnostic or therapeutic bronchoscopies. This approach is similar as in children, but reports in neonates are still limited to case reports. The use of a CPAP mask and maintaining spontaneous breathing significantly reduce the risk of relevant oxygen desaturation during the procedure.
Continuous administration of propofol has been used to facilitate procedural sedation during imaging procedures in neonates. Taking the aforementioned covariates (PNA and PMA) of propofol pharmacokinetics and the prolonged scanning times into account, we suggest to remain cautious with the use of prolonged propofol infusion in neonates. Although limited to one single case, Sammartino reported on the clinical and metabolic symptoms of “propofol infusion syndrome” in a preterm neonate [94].
In the absence of integrated PK-PD models in neonates, we can only speculate on the propofol concentration to aim for in neonates [102]. However, when we take the available pharmacokinetic estimates in early life into account, accumulation may occur even at “routine adult or pediatric” doses in early neonatal life. Although propofol seems to be a promising compound for versatile short-acting analgo-sedation, dose findings and safety studies are urgently needed. In a recent Cochrane review, Shah et al. concluded that no practice recommendation could be made based on the available evidence regarding the use of propofol in neonates [103]. Further research is therefore needed on the pharmacokinetics of propofol in neonates. Once a relatively safe dose range is identified, randomized controlled and comparative trials assessing the safety and efficacy of propofol are needed.
Take-Home Messages
There is extensive variability in propofol clearance within the neonatal population, in part explained by both PNA and PMA [95, 102].
There is conflicting information on the magnitude of hemodynamic (side) effects of propofol in (pre)term neonates [98, 101].
There is experience with IV bolus propofol administration to facilitate endotracheal intubation, but there is important variability in clinical characteristics, outcome criteria, co-medication, and doses evaluated in the different studies [96–100].
We do not recommend the use of propofol for sedation in ventilated neonates.
Ketamine
Ketamine is an anesthetic agent that provides amnesia, sedation, and analgesia. It can be administered by intravenous, intramuscular, nasal, rectal, or oral route with a systemic bioavailability of 93 %, 50 %, 25 %, and 17 %, respectively. It has an established role in pediatric anesthesia and is routinely used for induction and maintenance of anesthesia. This is in part due to the fast onset (30–60 s) and short duration of action, with limited hemodynamic and respiratory effects. The analgo-sedative effects are mediated through different mechanisms and contain both peripheral and central side effects. The contribution of N-methyl-d-aspartate (NMDA) receptor antagonism and interaction with cholinergic, adrenergic, serotonergic, opioid pathways, and local anesthetic effects remains to be fully elucidated. Hypersalivation is commonly observed during ketamine administration, resulting in the clinical practice to coadminister atropine or another antisialagogue. Ketamine is rarely used as a single anesthetic agent, is more commonly used as part of a multimodal anesthesia strategy, but can also be considered for procedural analgo-sedation [104, 105]. The cardiovascular stability observed with ketamine has made it a popular induction agent in infants with a congenital cardiopathy. In contrast, raised intracranial or intraocular pressure may be contraindications for ketamine analgo-sedation.
Pharmacokinetics
Ketamine is a highly lipid-soluble drug with rapid distribution from the systemic circulation to the brain. Due to these characteristics, systemic absorption of caudally or epidural-injected ketamine is also more likely [106]. It is a racemic (50/50) mixture of two enantiomers, and the S(+) enantiomer is four times more potent compared to the R(−) enantiomer. Ketamine undergoes N-demethylation to norketamine. This metabolite has limited analgo-sedative effects (30 % of the parent compound). Plasma protein binding is limited (47 %), and the metabolic clearance strongly relates to the hepatic blood flow with a high extraction ratio. Ketamine displays extensive first-pass drug metabolism, explaining the much higher doses suggested for oral as compared to intravenous administration, while rectal administration results in less predictable exposure. Consequently—when corrected for allometric differences—clearance in children and infants is similar to adults, but reduced (80–26 L/h/70 kg) in neonates [107]. In a randomized, crossover, trial in 16 preterm neonates plasma ketamine concentrations 15 min after intravenous administration (0.5, 1, or 2 mg/kg compared to placebo) were 103 (range 73–134), 189 (144–235), and 379 (320–437) ng/mL, respectively. Unfortunately, norketamine data were not collected, and sampling was limited to the 15 min time point [105]. The earlier discussed PK ketamine data explains that the dosing suggestions for analgo-sedation in neonates (0.5–1 mg/kg) are lower when compared to older children and much higher for oral as compared to intravenous administration (2–5 mg/kg oral).
Pharmacodynamics
The number of observations on effectiveness and safety of ketamine in neonates is limited. In the earlier-mentioned study of Saarenmaa et al., these authors evaluated the ketamine-related pain relief in an endotracheal suctioning model in 16 preterm (31, SD 3 weeks) neonates. The increase in heart rate, arterial blood pressure, and plasma catecholamines in response to endotracheal suctioning was not blunted when different (0.5, 1, and 2 mg/kg) doses of ketamine were compared to the response after placebo [105].
Another dataset relates to the use of ketamine sedation during the treatment of retinopathy of prematurity. In a NICU ward setting, ketamine sedation allowed laser therapy for retinopathy of prematurity in 11 preterm neonates (14 procedures). An empirical initial intravenous dose of 0.5 mg/kg was given, followed by further increments every 2 min if the child became distressed at insertion of the speculum. The median total dose was 2.4 mg/kg, the median duration of the intervention 1.6 h. Atropine was coadministered to minimize the salivation effect and to blunt reflex bradycardia [108]. We would also like to emphasize a single case report of a newborn with epidermolysis bullosa. Oral ketamine was used in this patient to facilitate dressing changes. Over 4 days, the dose was titrated from 0.125 to 0.75 mg/kg and resulted in sufficient sedation within 15 min after administration and dressing changes without crying or resisting for 45 min [108]. We hereby would like to mention that this oral dose is lower compared to the oral dosing suggested. In our opinion, differences in intestinal permeability support the need for dosing individualization [109].
Finally, there is growing concern about ketamine causing dose- and duration-related neuronal apoptosis in animal (mice, rat, rhesus monkey) experimental studies soon after birth. At present, it is unclear to what extent this also applies to human neonates and infants. Moreover, similar animal experimental observations have been reported for other analgo-sedatives (e.g., opioids, benzodiazepines, propofol, inhalational agents) [6–10].
Take-Home Messages
Ketamine is rarely used as a single anesthetic agent, but is more commonly used as part of a multimodal anesthesia strategy.
The clinical experience with ketamine in neonates is limited.
Remifentanil
Besides morphine and fentanyl, there are also observations on shorter-acting opioids in neonates. Alfentanil, sufentanil, or more recently remifentanil have been used mainly for short procedures such as endotracheal intubation, retinal laser surgery, or percutaneous intravenous central catheter placement, while there is anecdotal experience during major surgery and to maintain analgo-sedation during mechanical ventilation [93]. Remifentanil hydrochloride is a short-acting, μ(mu)-receptor opioid agonist. It achieves its peak analgesic effect within a minute of administration, 3–4 times faster when compared to fentanyl and much faster when compared to morphine.
Table 15.5 provides a summary of the available studies on endotracheal intubation with remifentanil in neonates [110–114]. These studies do reflect the difference between the reported studies on remifentanil to facilitate endotracheal intubation in (pre)term neonates. There is variability in clinical characteristics (preterm or term, INSURE, or continuation of ventilator), outcome criteria (intubation score, duration of the procedure, physiological variables), co-medication, and doses (1–4 μ[mu]g/kg IV slow bolus) evaluated. The total number of neonates exposed to remifentanil in these studies suggests that further investigations on dose seeking and safety are clearly indicated.
Table 15.5
Summary of the reported studies on remifentanil to facilitate endotracheal intubation in (pre)term neonates, reflecting the variability in clinical characteristics, outcome criteria, co-medication, and doses (1–4 μ[mu]g/kg, dose highlighted) evaluated
Reference | Study design and results |
---|---|
Norman et al. [110] | Randomized controlled trial in 34 preterm (<37 weeks) neonates for semi-urgent intubation. Atropine/morphine compared to RSI (rapid sequence intubation, based on glycopyrrolate, thiopental, suxamethonium, and remifentanil [1 μ(mu)g/kg]). Primary outcome: intubation score ≤10, secondary outcomes: procedural duration, physiological/biochemical variables, aEEG, and pain scores. Intubation score was superior in the RSI group [5 (IQR 5–6) compared to 12 (IQR 10–13.5)]. Plasma cortisol and pain scores were similar, but fluctuations in physiological variables were more pronounced and prolonged in the morphine group |
Choong et al. [111] | Double blind, randomized controlled trial, 30 (pre)term neonates, semi-elective intubation. Remifentanil (3 μ[mu]g/kg) compared to fentanyl (2 μ[mu]g/kg) and succinylcholine (2 mg/kg). Primary outcome: time to successful intubation. Secondary outcomes: physiological variables, adverse events, survey on intubation conditions, and time until return of spontaneous respiration. There were no differences in time to successful intubation (156/247 s). Premedication with remifentanil attenuated physiological responses during intubation comparable to fentanyl and succinylcholine in neonates. Intubation condition were rated more favorably with fentanyl/succinylcholine. Muscular rigidity was observed in the remifentanil group (n = 2/15) |
Welzing et al. [112] | Prospective, descriptive pilot study in 21 preterm (29–31 weeks) neonates receiving remifentanil (2 μ[mu]g/kg, combined with atropine, 10 μ[mu]g/kg) as induction agent for the INSURE (intubation–surfactant–extubation) procedure. Outcome variables were intubation conditions, time until extubation, and complications. Intubation conditions were qualified as excellent or good. Average extubation time after surfactant administration was 16.9 (1–45 min), followed by a mean of 3.3 (1–8) days of respiratory support (CPAP) |
Pereira e Silva et al. [113] | Double-blind randomized controlled trial in 20 preterm (28–34 weeks) neonates to evaluate intubation conditions following either morphine (150 μ[mu]g/kg) or remifentanil (1 μ[mu]g/kg), both combined with midazolam (0.2 mg/kg). Overall intubation conditions were better in the remifentanil group |
Hume-Smith et al. [114] | Remifentanil dose-seeking study (sequential up-and-down design), including 20 neonates and young infants (0–<4 months, mean weight 5.9 kg). The ED50 was 3.1–3.7 μ(mu)g/kg when remifentanil was coadministered with glycopyrrolate (10 μ[mu]g/kg) and propofol (5 mg/kg) |
Data on a dose–response for remifentanil to facilitate endotracheal intubation have only been published in two studies, with solely a focus on term neonates and young infants. Based on observations in 32 “term neonates,” it was documented that the effective remifentanil dose in 50 % and 98 % (ED50 = 1.7, SD 0.1 μ[mu]g/kg, and ED98 = 2.88, SD 0.5 μ[mu]g/kg) were similar between “neonates” (mean weight 8 kg, SD 2.2) and children [115]. However, this remifentanil dose was part of a multimodal anesthesia in combination with propofol (4 mg/kg), and glycopyrrolate (10 μ[mu]g/kg) and the “neonates” were in fact infants (mean age 7 months, SD 3.3). In another dose–response study with sequential up-and-down design, 20 neonates and young infants (0–<4 months, mean weight 5.9 kg), the ED50 was significantly higher (3.1–3.7 μ[mu]g/kg) when remifentanil was coadministered with propofol (5 mg/kg) and glycopyrrolate (10 μ[mu]g/kg) [114].
To assess the analgesic and procedural efficacy of low-dose remifentanil infusion during percutaneous central catheter placement in preterm infants, 54 preterm neonates were randomly assigned to remifentanil infusion (0.03 μ[mu]g/kg/min) or placebo in addition to 0.3 mL of 12 % sucrose (oral) combined with nonnutritive sucking. Pain (PIPP = Premature Infant Pain Profile) scores were significantly lower in neonates exposed to remifentanil, suggesting better pain and distress control without significant difference in the time to complete the procedure and the number of attempts [116]. Sammartino et al. reported on their experience with remifentanil (0.75–1 μ[mu]g/kg/min at start, 3–5 μ[mu]g/kg/min during procedure) combined with intravenous midazolam (0.2 mg/kg) for retinal laser therapy in 6 preterm neonates [117]. The same group also reported on two cases of babies born at 26 weeks and 27 weeks gestation, weighing 580 g and 400 g, respectively, undergoing laparotomy for necrotizing enterocolitis [118]. Both received a midazolam bolus and continuous remifentanil infusion at high doses. Finally, this group also reported on their experience with remifentanil for analgo-sedation during mechanical ventilation. In their hands, remifentanil provided adequate analgesia, with a significant reduction of NIPS and COMFORT scores since 1 h after starting the infusion of remifentanil [119]. The drug was initially administered at a dose of 0.075 μ(mu)g/kg/min, but in 73 % of newborns, the latter had to be increased up to a dose of 0.094 (SD 0.03) μ(mu)g/kg/min. Using this dose, 97 % of the newborns were classified as having adequate analgesia and sedation. The time elapsed between the discontinuation of remifentanil infusion and extubation was 36 (SD 12) min, reflecting the short-acting character of this compound [119].
However, in the clinical setting, these short-acting and versatile characteristics need further considerations. A specific advantage of remifentanil is that this compound undergoes metabolic clearance by plasma esterases, resulting in fast and predictable clearance, irrespective of liver or renal function. The analgo-sedative effects disappear very soon after discontinuation of remifentanil since the drug is cleared very rapidly. This is perfect or optimal when used for procedural analgo-sedation without subsequent pain. However, the “short-acting” concept hereby refers to both its onset of action and end of action: remifentanil-related analgo-sedation disappears very soon after discontinuation. This warrants anticipation and its management may be dependent on the indication [120]. When used for major surgery, anticipation and replacement by another (longer) acting opioid or non-opioid analgesic is needed, or the remifentanil infusion should be prolonged. Further continuation will, however, more likely result in potential negative effects such as opioid-induced tolerance or hyperalgesia since these phenomena are much more common when opioids with a short elimination half-life are administered [120].
A recent trial in Europe, the RAPIP trial, examined whether remifentanil induced tolerance, withdrawal, or hyperalgesia in infants. An RCT of intubated infants between 36 and 60 weeks gestational age and PNA, respectively, compared the efficacy and safety of a remifentanil- to fentanyl-based sedation regimen. When administered for less than 96 h, remifentanil did not increase the risk of tolerance, withdrawal, or opioid-induced hyperalgesia [121].
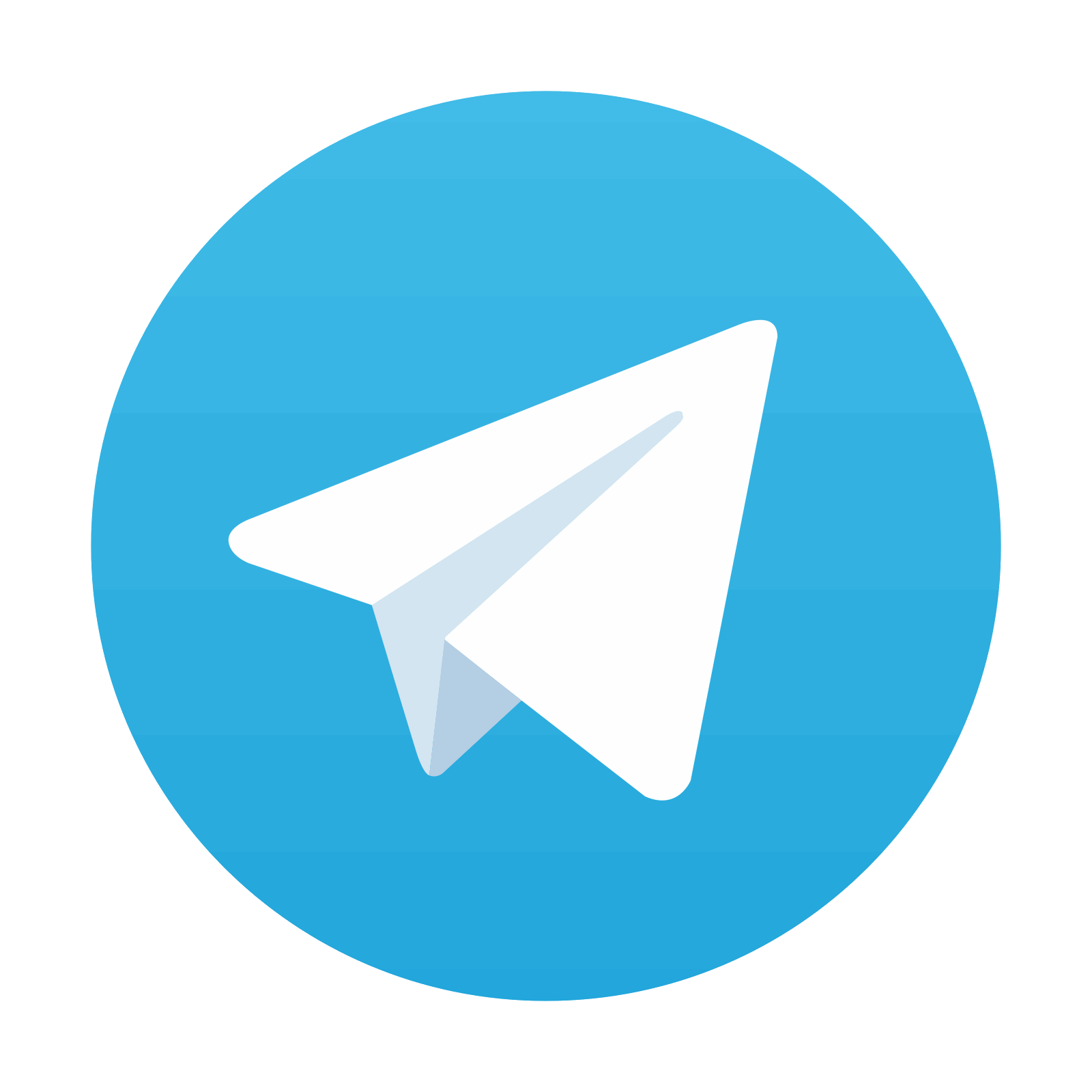
Stay updated, free articles. Join our Telegram channel

Full access? Get Clinical Tree
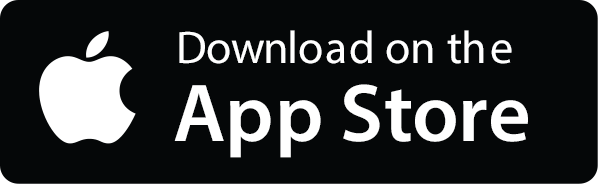
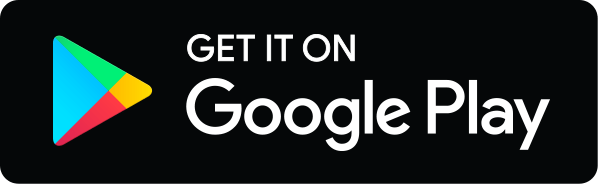