(1)
Bordeaux, France
The principal objective of mono- or paucifollicular ovarian stimulation is to improve or restore ovulation for a patient in which the process is occurring improperly or not at all, or to rescue additional follicles from atresia. On the other hand, a multifollicular stimulation is by its nature disruptive to normal ovarian function because it promotes maturation and development of abnormally numerous follicles. For this reason, it would appear useful to review briefly a few essential concepts of physiology as a way to orient thoughts on the logic, consistency and protocols of different approaches to ovulation stimulation.
At the heart of this process lies the relationship between the ovary and the anterior pituitary gland. The pituitary itself is controlled from the hypothalamus through its gonadotropin releasing hormone (GnRH, gonadorelin) that is in turn subject to influences from multiple areas of the central nervous system that express varied forms of external stimulation upon the pituitary-ovarian axis. Ovarian follicles secrete steroid hormones that act on their respective peripheral hormonal receptors, and they additionally exert feedback actions on the hypothalamic-pituitary complex.
2.1 The Anterior Pituitary-Ovarian Axis
Throughout her reproductive life, a woman’s reserve of primordial follicles declines with advancing age, from some 200,000 at puberty to less than 1,000 at menopause. Each primordial follicle contains an oocyte resting in meiotic prophase I. The fate of a follicle destined for ovulation is actually decided 3 months earlier, when it is “awakened” from this primordial pool. Together with several others, it commences a slow maturation process under the influence of paracrine factors until arriving at the pre-antral stage, a crossing point controlled by anti-Müllerian hormone (AMH). At this point the targeted follicles acquire FSH receptors for the first time. Whereas previous growth was FSH-independent, the structures now become hormonally dependent. By the start of a menstrual cycle, several pre-antral follicles, each 2–5 mm in diameter, have survived the sequence of events during this “awakening” period (Fig. 2.1).

Fig. 2.1
Development of the ovarian follicle from the primordial stage through ovulation
As the menstrual cycle terminates, corpus luteal decay results in a fall of estrogen and progestin secretion that triggers a chain of endometrial changes leading to menstruation. This decline of steroids and inhibin A removes their inhibitory effects on the hypothalamic-pituitary axis and permits a rise of FSH that is perceptible in plasma even before the first day of the menstruation.
Enhanced secretion of FSH, at the end of the previous cycle and during the subsequent early follicular phase, stimulates pre-antral follicles inconsistently because the sensitivity of each follicle is correlated with its respective endowment of FSH receptors. As the growing wave of FSH surpasses the threshold of the most hormone-sensitive follicles, a period of rapid growth ensues for those recruited early, and an “FSH window” opens. With concomitant stimulation by pituitary LH, cells of the theca interna deliver an androgen substrate to the aromatase complex of adjacent granulosa cells that will synthetize increasing amounts of estradiol and also the protein hormone inhibin B. Estradiol in turn furthers the synthesis of additional FSH receptors, with assistance from FSH itself. This “snowballing” effect amplifies follicular sensitivity to both gonadotropins and enhances growth of follicles to the antral stage that is evidenced by accumulating follicular fluid. At the same time, estradiol and FSH induce the synthesis of LH receptors on the granulosa cells, thereby promoting still more estrogen secretion.
The few follicles recruited at the beginning of the cycle are asynchronous with the others as a result of their unique sensitivity to FSH. Although initially indistinguishable morphologically from other follicles, the one whose FSH threshold is lowest begins to grow before the others. Its aromatase complex is the first to be aroused by FSH, and it begins to secrete estrogens before the others. This follicle is then enabled to enter the “snowball” phase ahead of the remaining cohort and is becoming the “selected one” to yield a gamete. As the other follicles join to secrete increasing amounts of estradiol and inhibin B, a negative feedback begins to impact pulsatile FSH release at the hypothalamic-pituitary level. On about the seventh day of the menstrual cycle, FSH secretion falls and the “FSH window” closes. Only the selected follicle continues growing, thanks to its rich population of FSH receptors that permits development even in the face of declining FSH. Whereas growth of all the remaining less sensitive follicles diminishes or stops altogether during the late follicular phase, the lead follicle, now 1 cm in diameter, expands due to additional stimulation from an FSH-like effect of LH. The aromatase complex also becomes functionally coupled to LH receptors, permitting additional LH participation in the selected follicle’s primacy and its late follicular phase maturation. By this time all of the other irregularly stimulated follicles that were initially recruited at the beginning of the cycle have undergone atresia and vanish within the ovarian stroma.
When plasma estradiol levels have been maintained in excess of 100 pg/ml for more than 48 h, and when the selected follicular diameter begins to exceed 16 mm, a large release of hypothalamic GnRH elicits a surge of pituitary FSH and LH. This final gonadotropin wave initiates a stream of follicular events leading to an initial erosion of the follicular wall accompanied by an increase of follicular fluid osmotic pressure that provokes a triad of events: a follicular rupture at the apex, a differentiation of the cumulus, and a final maturation of the oocyte itself. The oocyte’s meiotic process is awakened from prophase I through metaphase II, where maturation is again halted to await arrival of a haploid spermatozoon.
Following expulsion of the ovum, the follicle’s remaining granulosa cells become heavily vascularized and join with remaining theca interna to be transformed into luteinized cells. The corpus luteum actively secretes estradiol accompanied by a large output of progesterone, all being directed by a pulsatile signal from pituitary LH. Luteal lifespan is already programmed for 12–14 days unless its function can be rescued and maintained by exponentially rising chorionic gonadotropin (hCG) levels from trophoblast of an implanting embryo. While the menstrual cycle progressively develops under the influence of two pituitary gonadotropins, FSH and LH, the third gonadotropin, hCG, of placental origin, appears once the embryo implants. This signals the transformation of a menstrual cycle into a beginning pregnancy.
2.2 Follicle-Stimulating Hormone (FSH)
Throughout the menstrual cycle, follicle-stimulating hormone is secreted in a pulsatile manner by gonadotrophs in the adenohypophysis, a process that is itself controlled through a pulsatile release of hypothalamic gonadorelin (GnRH). FSH is delivered through blood to specific receptors on the surface of the ovarian granulosa cells and act to promote follicular growth and development. Despite a modest degradation by the liver, and elimination from the body by the kidneys, urinary FSH retains its biological activity (i.e., it is still able to bind to, and activate, ovarian receptors).
The evolution of ovarian follicles is directed by substantial changes of plasma FSH levels throughout the menstrual cycle. Levels are highest during the end of the luteal phase and early follicular phase (the recruitment period). Then FSH begins to decrease in mid-follicular phase (the selection-leadership period) due to the negative feedback effect of rising levels of estradiol and inhibin B (Fig. 2.2). There is a pre-ovulatory surge of FSH, synchronous with the LH surge, and then levels enter a declining period, only to rise again at the end of the luteal phase.

Fig. 2.2
Patterns of plasma FSH and LH throughout the menstrual cycle
The physiologic role of FSH is directed solely toward proliferation and development of ovarian granulosa cells, thereby to control follicular growth. FSH also induces secretion of inhibin B and, together with estradiol, the appearance and proliferation of LH receptors in the follicle. Finally the pre-ovulatory FSH peak participates importantly in the maturation of the cumulus-oocyte complex.
2.2.1 FSH: A Complex Molecule
Follicle-stimulating hormone is a heterodimer composed of alpha and beta subunits linked noncovalently. The alpha subunit is a chain of 92 amino acids folded by five disulfide bonds, and is identical to the alpha subunits of other glycoprotein hormones: thyrotropin (TSH), luteotropin (LH), and chorionic gonadotropin (hCG).
The beta subunit of FSH is composed of 111 amino acids folded by six disulfide bonds. However, this structure, as with each of the glycoprotein hormones, is unique to its respective hormone. Neither subunit of FSH has biological activity; only the conjoined heterodimer is capable of expressing a hormonal response.
FSH molecules are also characterized by glycosylation: each subunit bears two oligosaccharide chains (glycans) linked to asparagine residues (Asn 52 and Asn 78 on the alpha subunit, Asn7 and Asn 24 on the beta-subunit). These saccharide chains are constructed of N-acetyl-glucosamine, monosaccharides (mannose, galactose), and terminal sialic acids that become more numerous through side-chain branching. Varied oligosaccharide branching results in a number of unique FSH isoforms, also known as isohormones or glycoforms [1]. The pattern of branching is responsible for their richness of sugars (glycosylation) and sialic acid (sialylation) (Fig. 2.3). The glycosylated chains are essential for the full expression of hormonal activity, and cleavage leads to a progressive decline of activity in target cells. The alpha subunit saccharide chains appear to be most important for FSH receptor activation and initiation of the intracellular signal transduction process (adenylate cyclase), whereas the beta subunit chains are known to play a major role in protecting the FSH molecule against degradation and elimination.

Fig. 2.3
Linkage of the polysaccharide groups to the two FSH subunits. The groups extend outside the molecular axis formed by the two interlaced subunits, and thereby increase molecular bulk. The number of radicals linked to the beta subunit distinguishes human FSH tetra- (a), and bi- (b) sialylated molecules, as well as tri-sialylated equine FSH (c), and human recombinant FSH (d)
Some 20–30 FSH isoforms have been identified with distinctive oligosaccharide chain complexes that determine their ability to be maintained in circulation, to bind to the FSH receptor, and to induce a biological response [2]. Observations that the mix of isoforms found in pituitary tissue and in plasma are identical suggest that circulating hormone is not simply the degradation product of a single secreted molecule [3]. Physicochemical characteristics of each isoform are determined by three parameters which affect their physiological properties (Fig. 2.4):

Fig. 2.4
Isoforms of human FSH (Redrawn from Ref. [1])
-
Their degree of sialylation in relation to the number of the terminal sialic acid residues, in part a function of the oligosaccharide chain complexity.
-
Their degree of sulfonation (sulfonic acid radicals): there is significant competition between galactosamine transferase and galactotransferase at the terminal N-acetylglucosamine residue of the common mucopolysaccharide core. The former attaches an N-acetylgalactosamine residue, followed by a terminal sulfonate, whereas the latter enzyme attaches a galactose residue followed by a terminal sialic acid. In contrast to pituitary LH, no FSH isoform contains more than two sulfonate radicals.
-
Their degree of glycosaccharidic chain intricacy, in relation to the richness in chain branching and complexity, and particularly the presence of a bisecting N-acetylglucosamine radical between two other common core segments.
As the branch complexity of FSH isoforms increases, the molecules become more acidic, due to the lateral chains acquiring two to four terminal sialate radicals (or “antennas”). Isoforms are distinguishable by their sialic acid content which proportionately lowers the isoelectric point (pI). Natural FSH becomes a mixture of isoforms with isoelectric points between three (most acidic) and six (least acidic). Nevertheless, mere recognition and identification of the varied FSH isoforms based solely on pI is not in itself sufficient; the richness of internal branching and the number of “antennas” also plays an important part in bioactivity. Isoforms with varied oligosaccharide complexity or degree of sulfonation can share the same level of overall sialylation yet express distinct physiological properties.
Glycosylation of the FSH beta subunit is a separate issue. A portion of the subunit molecules have no glycosylation at either Asn 7 or Asn 24 which results in a mixture of tetra- (MW 24,000) and bi- (MW 21,000) sialylated molecules. This mixture is the result of the hormonal milieu in blood: estradiol decreases the rate of N-glycosylation, most likely through inhibition of oligosaccharyl transferase. Bi-glycosylated forms comprise 75–95 % of the molecular population in postmenopausal women, but only 35–40 % of plasma FSH in menstruating women [4]. This shift of proportion exerts important effects upon the 3-dimensional configuration of the FSH molecules, and hence upon plasma clearance. Both of the N-glycans of the alpha subunit are aligned along the molecule’s axis, whereas the N-glycans of the beta subunit extend outward, effectively doubling the molecular diameter and reducing its rate of glomerular filtration. Studies of a mutant di-glycosylated recombinant FSH showed an elimination rate from blood to be twice as fast as that of normal tetra-glycosylated rFSH [5]. This “all or nothing” process of beta subunit glycosylation provides yet another control mechanism over bioactivity, plus it alters the electrical charge of the molecule as the proportion of the 2- and 4-glycan isoforms shifts. A final point: 3-glycosylated isoforms, having a single radical on the beta subunit (Asn 24), may be found in natural equine FSH or in recombinant human FSH (on Asn 7) but this is never found in naturally occurring human FSH.
The degree of sialylation, and of possible sulfonation, exerts a considerable influence on metabolism of the various isoforms. FSH metabolism begins with removal of hormone from plasma by binding to receptors for specific sialic acid or sulfated residues in hepatic epithelial cells, and followed by renal excretion. More numerous sialic residues on a molecule hinder its hepatic capture and slow its glomerular filtration due to the expanded molecular size. Consequently, the more sialylated and acidic isoforms persist in blood for a longer period. An isohormone with a pI of 4.27 has a half-life of 24 h, but the duration falls to 12 h for isoforms at a pI of 5.49 [6]. On the other hand, for the same degree of sialylation, the addition of sulfone radicals enhances the rate of extraction and elimination of the glycoforms, in direct proportion with the number of SO radicals [7]. Thus the pituitary actually secretes a mixture of isoforms, and each interacts uniquely with the ovarian FSH receptor.
In addition, the structural mix of secreted molecules is not static, and it changes throughout one’s life. The mix also changes throughout the menstrual cycle in reproductive-age women, in accordance with GnRH pulsatility that is principally controlled by the plasma levels of estradiol. The more acidic FSH isoforms prevail during lower estrogen environments, such as before puberty and after menopause. Similarly, more acidic isoforms dominate at the beginning and the end of the menstrual cycle, and less acidic isohormones prevail during the pre-ovulatory period to produce a more homogenous distribution (Fig. 2.5) [8]. Individual patients secrete a uniquely tailored isoform profile that is already distinguishable on cycle day 3. The same occurs for LH isoforms [9].

Fig. 2.5
Profile of FSH isoform secretion during the menstrual cycle (Redrawn from Ref. [2])
Bioactivity of the isoforms also varies according to the model studied. Whereas in vivo activity is principally related to the molecular half-life, in vitro activity becomes more a function of its affinity for the cellular receptors. The relative activity of FSH isoforms is affected by their degree of glycosylation, in an apparently conflicting manner [10]:
-
The more acidic glycoforms show a higher bioactivity in vivo, for example, in the Steelman-Pohley bioassay that measures a dose–response variation of immature rat ovarian weight in relation to the injected FSH doses.
Stay updated, free articles. Join our Telegram channel

Full access? Get Clinical Tree
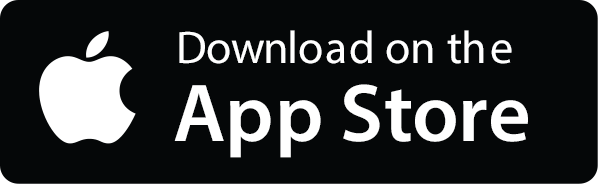
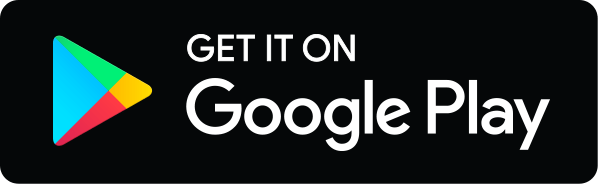