© Springer International Publishing Switzerland 2015
Timothy P. Cripe and Nicholas D. Yeager (eds.)Malignant Pediatric Bone Tumors – Treatment & ManagementPediatric Oncology10.1007/978-3-319-18099-1_1414. Recurrent Bone Tumors
(1)
Division of Pediatric Hematology/Oncology, Shands Hospital for Children, University of Florida, 1515 SW Archer Road, Gainesville, FL 32608, USA
(2)
Division of Hematology/Oncology/BMT, Nationwide Children’s Hospital, 700 Children’s Drive, Columbus, OH 43205, USA
14.1.1 Incidence of Recurrence
14.1.2 Risk Factors for Relapse
14.1.4 Outcome After Relapse
14.1.5 Therapeutic Approach
14.1.7 The Future for Osteosarcoma
14.2.1 Incidence of Recurrence
14.2.2 Outcomes at Recurrence
14.2.3 Risk Factors for Recurrence
14.2.5 Therapeutic Approach
14.2.6 Emerging Targets
14.2.7 Future of Ewing Sarcoma
Abstract
Multimodal approach to treatment has led to the dramatically improved outcome of patients with osteosarcoma and Ewing sarcoma. However, despite intensive therapies and incorporation of novel agents in trials, the therapeutic plateau in recurrent osteosarcoma and Ewing sarcoma has not yet been overcome. Cure for these patients remain a daunting challenge with dismal survival rate after relapse despite best efforts. Certain clinical factors help prognosticate quality of relapse of these tumors. Because patient characteristics are different upon disease recurrence, therapeutic approach to these patients vary widely.
Further improvements in outcome for patients with recurrent disease will depend on refinement of therapy using agents with clinical activity based on understanding of tumor biology, and within a trial design that optimally detects drug activity. There is a growing list of targets for osteosarcoma and Ewings sarcoma. A significant biology effort has been established by cooperative groups, as well as preclinical drug evaluation systems created towards more rapid identification and validation of compounds active in osteosarcoma and Ewing sarcoma. High throughput screens of novel agents should accelerate clinical trial development. Finally, there are now various national and international cooperative groups to efficiently test new agents in these rare tumors.
14.1 Recurrent Osteosarcoma
In the last three decades, introduction of chemotherapy has greatly improved the prognosis of patients with osteosarcoma (Fuchs et al. 1998; Giuliano et al. 1984; Goorin et al. 1991; Jaffe et al. 1983; Longhi et al. 2006; Saeter et al. 1995). Currently however, despite multimodal intensive treatment, a plateau in the survival and cure rates of osteosarcoma has been reached (Chou and Gorlick 2006). About three fourths of patients with metastases at diagnosis will relapse (Marina et al. 1993; Meyers et al. 1993; Ward et al. 1994). For patients with localized disease at initial presentation, 30–40 % will develop recurrence (Fuchs et al. 1998; Goorin et al. 1991; Jaffe et al. 1983; Kempf-Bielack et al. 2005; Longhi et al. 2006; Saeter et al. 1995). Survival rates continue to be unsatisfactory for patients with metastatic and recurrent disease, with a 10-year overall survival (OS) of 23–29 % (Carrle and Bielack 2009; Kager et al. 2003) and less than 20 % (Kempf-Bielack et al. 2005), respectively. Subjects enrolled on INT-0133 who experienced recurrence had an overall survival of 20 % at 10 years, similar to outcomes reported by other groups. Bielack et al. (2009) reported 5 year OS and event-free survival (EFS) estimates with second and subsequent osteosarcoma recurrences: 16 % and 9 % for the second, 14 % and 0 % for the third, 13 % and 6 % for the fourth, and 18 % and 0 % for the fifth recurrences, respectively. In this study, the median interval from the first to second recurrence was about 9 months, and the median interval between subsequent recurrences remained at approximately 6 months.
14.1.1 Incidence of Recurrence
14.1.1.1 Timing of Recurrence
Most patients developed disease recurrence within 2 years of initial diagnosis (Bacci et al. 2001, 2008; Chou et al. 2005; Duffaud et al. 2003; Ferrari et al. 2003; Hawkins and Arndt 2003; Kempf-Bielack et al. 2005). In the Cooperative Osteosarcoma Study Group (COSS) report of 576 patients who had achieved first complete remission (CR) then developed recurrent disease, the median time from diagnosis to first relapse was 1.6 years (Kempf-Bielack et al. 2005). This was identical to what was described in Chi et al. (2004), Duffaud et al. (2003), and Hawkins and Arndt (2003), but approximately half a year shorter than the median time of 23 months from the first complete remission reported by the Rizzolli Institute (n = 162) (Ferrari et al. 2003).
14.1.1.2 Sites of Recurrence
The lungs were involved in more than 80 % of all patients with recurrent disease and as the only site in nearly two thirds of all patients (Briccoli et al. 2010; Chi et al. 2004; Chou et al. 2005; Duffaud et al. 2003; Ferrari et al. 2003; Goorin et al. 1984, 1991; Hawkins and Arndt 2003; Huth and Eilber 1989; Kempf-Bielack et al. 2005; Meyers and Gorlick 1997; Saeter et al. 1995). Local recurrence occurs in approximately 10 % of patients treated with limb-sparing surgery (Meyers and Gorlick 1997; Saeter et al. 1995; Weeden et al. 2001). In the COSS study, about 10 % relapsed in the bone other than the primary site and 10 % with multifocal recurrence (Kempf-Bielack et al. 2005). As also observed in other reports (Saeter et al. 1995), solitary relapses were more likely to occur in patients without primary metastases (p = 0.01) and more likely to be late relapses (p = 0.001).
14.1.2 Risk Factors for Relapse
Several independent risk factors are associated with the probability to develop osteosarcoma recurrence. Surgically inaccessible primary sites such as the axial skeleton as well as the pelvis, the large size of a primary tumor, and the presence of metastases at diagnosis make it very challenging to secure surgical local control, hence having higher propensity for relapse (Bielack et al. 2002).
Of the factors associated with initial disease presentation and treatment, response to first-line chemotherapy has been shown to be a predictive factor for relapse and correlates significantly with survival (Bielack et al. 2002; Martini et al. 1971; Spanos et al. 1976). Contrary to this, however, there is evidence that response to first-line chemotherapy is not an independent prognostic value for outcome after recurrence (Hawkins and Arndt 2003; Kempf-Bielack et al. 2005), as it seems like the benefit for relapsing good responders may have been mediated by longer times to recurrence. In addition, dose intensification of conventional cytotoxic agents, although leading to greater necrosis after preoperative chemotherapy, does not seem alter the poor outcome of patients with metastatic osteosarcoma (Gorlick and Meyers 2003; Meyers et al. 1998).
14.1.3 Prognostic Factors After Relapse
Outcome after osteosarcoma relapse is largely dictated by onset and location of recurrence, as well as ability to secure surgical local control. However, the reported overall survival rate in patients with relapsed osteosarcoma had a wide range of 13–58 % (Ferrari et al. 1997, 2003; Goorin et al. 1984, 1991; Martini et al. 1971; Rosenburg et al. 1979; Saeter et al. 1995; Schaller et al. 1982; Spanos et al. 1976; Ward et al. 1994). The discrepancies in outcome published by different studies could be from differences in the selection criteria for the type of relapse (i.e., lung relapse only versus all relapses). In addition, variability among prognostic factors in several series could be from studies with small study populations and differences in the treatment approach to recurrence.
14.1.3.1 Timing of Recurrence
While some studies failed to detect association with time to relapse and post-relapse survival (Carter et al. 1991; Goorin et al. 1984, 1991; McCarville et al. 2001; Meyer et al. 1987; Saeter et al. 1995; Tabone et al. 1994), there are numerous reports that confirm that patients with late relapses fared better. Improved survival has been associated by prolonged recurrence-free interval (RFI) (Ferrari et al. 2003; Hawkins and Arndt 2003; Korholz et al. 1998; Putnam et al. 1983; Thompson Jr. et al. 2002), variably defined as longer than 6 months (Putnam et al. 1983), 8 months (Ward et al. 1994), and 24 months (Briccoli et al. 2010; Chou et al. 2005; Ferrari et al. 1997, 2003; Hawkins and Arndt 2003). In a study by Chou et al. (2005), patients who developed disease recurrence before 24 months after primary diagnosis did significantly worse than those who recurred after 24 months. In a separate study, significantly higher disease-free survival (DFS) and OS were associated with RFI of >24 months (Hawkins and Arndt 2003). Bricolli et al. (2010) demonstrated that the EFS was significantly correlated with relapse interval, greatest in patients with >24 months RFI, followed by RFI 12–24 months, and the least in patients with RFI <12 months. This is in accordance to outcome data reported by Duffaud et al. (2003) (n = 24) and Harting et al. (2006) (n = 137). Unfortunately, even in patients who develop late relapse, the survival curve does not reach a plateau, and the ultimate course of disease tends to be relentless in most cases (Kempf-Bielack et al. 2005). Surgery and second CR were more likely to have been reported in patients with late or solitary relapses than in others (p < 0.001) (Chou et al. 2005).
14.1.3.2 Location of Recurrence
Lung
Solitary Versus Multiple Recurrence
In the COSS series of 576 patients (Kempf-Bielack et al. 2005), 39 % had solitary versus 36 % bilateral lung recurrence. Several earlier studies indicate that the number of pulmonary nodules detected at first relapse did not correlate with survival (Carter et al. 1991; Chou et al. 2005; Goorin et al. 1984, 1991; Tabone et al. 1994). However, there is significant evidence that suggests patients with multiple lesions fared worse than those with solitary relapses (Chou et al. 2005; Duffaud et al. 2003; Ferrari et al. 2003; Hawkins and Arndt 2003; Meyer et al. 1987; Putnam et al. 1983; Saeter et al. 1995; Thompson et al. 2002; Ward et al. 1994). Solitary pulmonary recurrence was associated with improved DFS and survival rates compared to multiple pulmonary nodules, likely because isolated pulmonary recurrence achieved a second complete remission (CR2) rate of 83 % (Hawkins and Arndt 2003), similar to other reported rates (Carter et al. 1991; Ferrari et al. 2003; Goorin et al. 1984; Pastorino et al. 1991; Putnam et al. 1983; Rosenburg et al. 1979; Saeter et al. 1995; Tabone et al. 1994).
Unilateral versus Bilateral Recurrence
In the COSS study (Kempf-Bielack et al. 2005), about 50 % had unilateral and 50 % had bilateral disease. In some studies (Carter et al. 1991; Chou et al. 2005; Goorin et al. 1984, 1991; Pastorino et al. 1991; Tabone et al. 1994), bilateral lung recurrence did not significantly correlate with survival (22 % versus 54 % p = 0.14). In contrast, presence of metastatic nodules in both lungs significantly correlated with poor survival (Hawkins and Arndt 2003; Kempf-Bielack et al. 2005; Saeter et al. 1995; Ward et al. 1994). Again, this is likely due to amenability to surgical local control in unilateral disease versus bilateral recurrence.
Pleural Involvement
Pleural metastases in patients with osteosarcoma may occur via two mechanisms, direct contact of pleura with metastatic lesions in the lungs and/or hematogenous spread of osteosarcoma. In general, pleural involvement of underlying cancer confers worse prognosis. This may be due to the relative difficulty of addressing surgical local control of pleural metastases and possible decreased distribution of chemotherapeutic agents to the pleura. Of note, early relapses to the lungs tend to disrupt the pleura (Kempf-Bielack et al. 2005; Saeter et al. 1995). In a study by Kempf-Bielack et al. (2005), the incidence of pleural disruption was noted at 11 %, and patients with pleural disruption by lung metastases fared worse than those without (p < 0.0001).
Other Prognostic Factors After Lung Relapse
In a large study of patients with extremity osteosarcoma with pulmonary involvement, either with recurrent disease or at initial presentation (Briccoli et al. 2010), it was found that outcome significantly correlated with tumor stage at presentation. The 5 year EFS for patients with localized disease who later relapsed in the lungs was 36 % versus those with resectable lung metastases at presentation 9 % (p < 0.0001). Outcome also significantly correlated with the presence of local recurrence in addition to lung metastases (p = 0.019). The number of thoracotomies performed in a single patient also correlated with outcome, with 5 year EFS of 38 % for patients who had only one thoracotomy versus 8.5 % in patients who had two or more thoracotomies (p < 0.0001). Of note there was no difference between two or three thoracotomies versus four or five (p = 0.29). Interestingly in this study, second-line salvage chemotherapy after relapse did not have an impact on outcome.
Other Metastatic Sites
Extrapulmonary recurrence is often believed to carry a grave prognosis, particularly distant bone recurrence of osteosarcoma (Tabone et al. 1994; Ward et al. 1994). Ferrari et al. (1997) reported no surviving patients among four patients with bone recurrence. In a subsequent study by Ferrari (Ferrari et al. 2003), the 5-year post-recurrence survival was 11 % in 37 patients with extrapulmonary recurrence, most of whom had distant bone recurrence.
In contrast, the COSS series (Kempf-Bielack et al. 2005) results imply that it is not the extrapulmonary site, but rather the high likelihood that extrapulmonary metastases are part of a disseminated disease process that leads to this impression. Recent reports have demonstrated that metachronous osteosarcoma limited to distant bone is not associated with a bleak prognosis (Aung et al. 2003; Jaffe et al. 2003; San-Julian et al. 2003). Multimodal therapy may have contributed to the survival of patients with extrapulmonary recurrences, as was noted by Hawkins et al. (Hawkins and Arndt 2003) where 20 % of patients with distant bone recurrence, either initial or subsequent recurrence, survived without active disease. The location and number of lesions cannot be viewed independently from resectability. There are long-term survivors exclusively among patients who achieved a second surgical remission (Carter et al. 1991; Duffaud et al. 2003; Ferrari et al. 2003; Goorin et al. 1984, 1991; Hawkins and Arndt 2003; Huth and Eilber 1989; Putnam et al. 1983; Saeter et al. 1995; Tabone et al. 1994; Ward et al. 1994).
14.1.3.3 Ability to Achieve Surgical Remission
Ability to achieve complete surgical control of disease is required for cure. The most important factor associated with prolonged survival was the achievement of second remission (CR2) via complete surgical excision. In the COSS series (Kempf-Bielack et al. 2005), failure to do surgery was the strongest negative prognostic factor for the entire cohort (any surgery p < 0.0001, macroscopically complete surgery p < 0.0001). The 4-year survival rate was 33 % for patients who achieved CR2 with a median survival period of 31 months versus 0 % survival rate and median survival period of 7.4 months in patients without CR2 (p < 0.001) (Hawkins and Arndt 2003). In a separate study, complete resection of all disease sites is correlated with survival (26 % versus 17 %, p = 0.05) (Chou et al. 2005). Inability to achieve surgical CR2 was associated with exceptionally poor outcome in other studies, with OS rates from 0 % to 8 % (Carter et al. 1991; Ferrari et al. 2003; Pastorino et al. 1991; Putnam et al. 1983; Saeter et al. 1995; Strauss et al. 2004).
14.1.4 Outcome After Relapse
Overall survival after osteosarcoma recurrence is <30 % (Bacci et al. 2001; Chi et al. 2004; Ferrari et al. 1997; Meyers et al. 1998; Strauss et al. 2004). Chou et al. (2005) reported in a cohort of 43 patients, the OS at 36 months was 35 %, relapse-free survival (RFS) 14 %, and average survival for patients treated with both chemotherapy and surgery was 29.6 months. In the COSS series (Kempf-Bielack et al. 2005), post-relapse actuarial OS at 5 and 10 years was 0.23 and 0.18, respectively. The median time to second relapse in 249 of 339 patients that were surgically disease-free was 0.8 year, shorter than the first relapse-free interval. The median survival was 1.2 years for all patients and 4.2 years for 148 survivors, whereby 82 patients were in CR2 with EFS 0.13 and 0.11 at 5 years and 10 years, respectively. In this cohort of patients, eventually only 37 were alive at the conclusion of the study, with 24 in CR3, 7 in CR4, 4 in CR5 4, 1 in CR7, and 1 in CR8.
Hawkins and Arndt (2003) reported, in a study with 59 patients, that the overall 4y OS was 23 % and 4-year disease-free survival (DFS) was 6 %. The discrepancy between DFS and survival rates for the entire study (6 % versus 23 %) and for patients with isolated pulmonary recurrence who achieved CR2 (7 % versus 28 %) showed the high probability of second disease recurrence. Despite the ability to achieve CR2, most patients with recurrent OS developed other disease recurrence. DFS analysis also demonstrated that the time to second disease recurrence was rapid at a median of 8.8 months after achieving CR2 in patients with isolated pulmonary recurrence.
In the Bricolli et al. study (Briccoli et al. 2010) of 323 extremity osteosarcoma patients with either resectable lung metastases at diagnosis or localized disease who relapsed with resectable lung metastases, 29 % were alive and disease-free, 4 % were alive with controlled disease, and 67 % died from progressive disease. The 5 year OS was 37 %. Other studies confirm poor overall prognosis of recurrent osteosarcoma, with an OS rate after recurrence ranging from 13 to 57 % (Bacci et al. 2001; Carter et al. 1991; Ferrari et al. 1997; Goorin et al. 1984, 1991; Kempf-Bielack et al. 2005; Martini et al. 1971; Pastorino et al. 1991; Putnam et al. 1983; Rosenburg et al. 1979; Saeter et al. 1995; Spanos et al. 1976; Tabone et al. 1994; Ward et al. 1994).
The most common cause of death in patients with relapsed osteosarcoma is progressive disease (Briccoli et al. 2010; Chou et al. 2005; Hawkins and Arndt 2003; Kempf-Bielack et al. 2005). In minority of cases, other causes implicated were sepsis/multiorgan failure, ARDS, perioperative complications, thromboembolic events, cardiomyopathy, pulmonary fibrosis, and treatment-associated AML/MDS (Chou et al. 2005; Kempf-Bielack et al. 2005).
14.1.5 Therapeutic Approach
14.1.5.1 General Approach
More than 90 % of patients with osteosarcoma had already been exposed to at least three of the agents considered most active against the disease: high-dose methotrexate, doxorubicin, cisplatin and ifosfamide. Majority of patients receive multi-agent chemotherapy in combination with aggressive surgery for treatment at relapse. The treatment for isolated pulmonary recurrence varied, most frequently involving surgery alone, or in combination with chemotherapy. The treatment of second disease recurrence was highly variable: surgery alone, surgery in combination with chemotherapy or a novel agent via enrollment in a trial, surgery plus radiation therapy or palliative therapy (Briccoli et al. 2010; Chou et al. 2005; Hawkins and Arndt 2003; Kempf-Bielack et al. 2005). For repeated relapses, use of second-line salvage chemotherapy with drugs not used in previous treatments or with the same drugs used at higher doses (methotrexate, ifosfamide), particularly when metastatic pattern suggests a particularly aggressive tumor behavior (i.e., patients with metastases at diagnosis, less than 2 years disease-free interval from the beginning of any treatment and first relapse, multiple lung metastases, or when complete surgical removal of all tumor was not feasible) (Briccoli et al. 2010).
14.1.5.2 Surgical Approach
Importance of Surgical Local Control
Ability to achieve complete surgical control of disease is required for cure (Briccoli et al. 2010; Chou et al. 2005; Ferrari et al. 2003; Goorin et al. 1991; Hawkins and Arndt 2003; Kempf-Bielack et al. 2005; Saeter et al. 1995). The general approach for late recurrence and presence of a solitary metastatic lung nodule is to do surgery alone (Chou et al. 2005). Hawkins and Arndt (2003) showed that for patients with solitary pulmonary recurrence, surgical resection alone provided a better 4-year survival compared with chemotherapy in addition to surgery (47 % versus 13 %, p = 0.005), and DFS rate was similar for patients treated with either surgery alone or surgery with chemotherapy. A subgroup analysis of patients with completely excised isolated pulmonary recurrence showed a superior 5-year survival with surgery alone, compared with surgery and second-line chemotherapy. For patients with short RFI, 5-year survival rate was 30 % with surgery alone versus 6 % with surgery and second-line chemotherapy (p = 0.0001). For patients with one or two pulmonary nodules, 5-year survival rate of 65 % was noted with surgery alone versus 30 % with surgery and second-line chemotherapy (p = 0.02). This may be due to selection of patients with recurrence amenable to surgical local control only salvage strategies versus patients who have disseminated disease at relapse who were deemed to require systemic chemotherapy for disease control.
Among treatment-related variables, surgery and a second surgical CR were correlated with improved OS (p < 0.0001), and failure to achieve second surgical remission was the strongest negative prognostic factor for the entire cohort (Kempf-Bielack et al. 2005). About 25 % of patients who relapsed with lung-only metastases could become long-term survivors and perhaps also be definitely cured by thoracic surgery alone or in combination with chemotherapy.
Durable remissions have been achieved in patients who experience two or more lung relapses. Bricolli et al. (2010) reported that among 323 patients with relapsed osteosarcoma, complete remission rate was 26 % for patients who had one relapse only and 18 % for patients who had four relapses (p = 0.79). Of the five patients who had five relapses, one is alive and disease-free 5 years from last relapse. In another study, 30 % (10 out of 33) remained in complete remission at a median follow-up of 34 months from surgery of metastatic lung nodules (Duffaud et al. 2003). In addition, Tabone et al. (1994) showed that 31 % (13 out of 42) were in complete remission after repeated surgeries at a median follow-up of 39 months. These studies showed that all patients in complete remission at the last follow-up had a complete surgical resection of all metastatic tumor tissue, but those who were not operated or only had a trial removal of disease did not survive (Briccoli et al. 2010; Duffaud et al. 2003; Tabone et al. 1994). Several series including Hawkins and Arndt (2003) and Bricolli et al. (2010) have shown the curative potential of pulmonary metastasectomy, with or without further adjuvant therapy.
Variable Reported Outcomes After Surgery
There are different possible reasons for variable reported outcomes after surgery in patients with recurrent osteosarcoma. First, the definition of complete resection varies, and often a loose criteria for complete remission (CR) in different studies is used. In a study by Goorin et al. (1984), a much more stringent criteria for CR was used: complete removal of macroscopic disease, negative margins, and no histologic evidence of pleural disruption by tumor. The use of such rigid criteria narrows down considerably the number of patients assumed to have achieved a second CR, possibly allowing a better definition of the subgroup from which survivors will originate (Kempf-Bielack et al. 2005). Second, threshold for resectability varies per institution and surgeon’s experience. In a large study (Briccoli et al. 2010), criteria for resectability of metastatic lung lesions included primary tumor under control, no pleural or pericardial effusions, no metastasis in other organs besides the lungs, and complete resectability leaving adequate residual pulmonary functions. These variables likely contribute to the variability of outcome in relapsed patients following surgery.
14.1.5.3 Chemotherapy
Unclear Role of Chemotherapy in Relapse Setting
Unlike in the treatment for primary disease, the role of chemotherapy in the setting of disease recurrence has been less clear as that of surgery. Although the benefit of multi-agent chemotherapy in addition to complete resection of all areas of tumor involvement has been proven in the initial therapy of OS in a randomized trial (Link et al. 1986), there has been no randomized study evaluating the benefit of chemotherapy after osteosarcoma recurrence.
Many investigators consider patients who relapse after completing modern chemotherapy resistant to further systemic treatment (Goorin et al. 1984; Pratt et al. 1987). Several studies (Ferrari et al. 2003; Pastorino et al. 1991; Tabone et al. 1994), have shown there was no benefit or observed difference in survival after second-line chemotherapy in the setting of relapse. In a large study (Briccoli et al. 2010), second-line salvage chemotherapy did not influence outcome, with EFS of 23 % for those who received chemotherapy (n = 111) versus 27 % for those who were treated with surgery alone (n = 212) (p = 0.42).
There are factors that complicate the analysis of the published literature regarding DFS and survival after osteosarcoma recurrence which make interpretation of impact of chemotherapy not well defined. First, in the past, chemotherapy given to patients who received neoadjuvant or adjuvant treatments often differed significantly from regimens used currently, including relatively inactive or non-intensive agents (Goorin et al. 1984; Gorlick and Meyers 2003; Pastorino et al. 1991; Putnam et al. 1983; Rosenburg et al. 1979; Schaller et al. 1982; Tabone et al. 1994; Ward et al. 1994). Second, most series only reported survival rates, and very few reported DFS or EFS after disease recurrence (Ferrari et al. 2003; Goorin et al. 1991; Tabone et al. 1994) making it difficult to evaluate impact of chemotherapy. Third, in many series, the number of patients treated with second-line chemotherapy was too small to draw conclusions regarding its impact on survival (Ellis et al. 1997; Rosenburg et al. 1979). More importantly, some series (Schaller et al. 1982; Spanos et al. 1976; Temeck et al. 1995) included selected cohorts of patients amenable to surgical excision, thereby excluding patients with unresectable, and therefore unfavorable, recurrent tumors. The superior surgical survival rates observed for patients treated with surgery alone were likely due to biased use of chemotherapy in patients with marginally excised or difficult to resect tumors, early or multiple recurrences, or short relapse-free interval (<1 year) (Chou et al. 2005; Hawkins and Arndt 2003). Because of these limitations, an unambiguous conclusion regarding the role of second-line chemotherapy could not be drawn.
Evidence to support chemotherapy
When primary mode of treatment for osteosarcoma involved surgery alone, most patients recurred in the lungs within 12 months (Friedman and Carter 1972). Introduction of chemotherapy has greatly improved prognosis for these patients (Fuchs et al. 1998; Goorin et al. 1991; Jaffe et al. 1983; Link et al. 1986; Longhi et al. 2006; Saeter et al. 1995). Moreover, the incorporation of chemotherapy to treatment of osteosarcoma has made DFS significantly longer and the number of lung metastases significantly lower compared to patients treated with surgery alone (Fuchs et al. 1998; Longhi et al. 2006; Saeter et al. 1995). This relapse pattern change seems to indicate a partial effect of combined chemotherapy, which may also benefit patients who relapsed (Briccoli et al. 2010). The adjuvant effect of chemotherapy is much less obvious than that of first-line treatment and may be easily missed when analyzing small cohorts. There might be some selection bias, as chemotherapy efficacy may have been underestimated as the patients who received chemotherapy were more likely to have presented with more extensive recurrences.
Several studies have demonstrated the possible benefit of second-line chemotherapy in the relapse setting. In a study by Saeter et al. (1995), survival improved in those patients (n = 60) with recurrent disease who received “adequate” chemotherapy, defined as receiving at least one agent to which the patient had not been previously exposed or a dose escalation of methotrexate, in addition to surgery.
It seems that impact of chemotherapy is a bit more obvious in patients who have not achieved second surgical remission. In the Cooperative Osteosarcoma Study Group (COSS) study (Kempf-Bielack et al. 2005), the use of chemotherapy is correlated with overall survival for patients who did not achieve a second complete remission (p = 0.0001). It was also noted in this study that chemotherapy use also was found to correlate with event-free survival for patients who were able to achieve a CR2 (p = 0.016). When multidrug chemotherapy was compared with no chemotherapy or single-agent chemotherapy, multidrug chemotherapy is correlated with overall survival in the total cohort (p = 0.012). The use of second-line chemotherapy is correlated with good response to first-line chemotherapy (p = 0.022), multiple lesions at relapse (p = 0.001), and bilateral pulmonary involvement (p = 0.001), but not the time to relapse (p = 0.953). Most patients treated without chemotherapy for a first relapse will undoubtedly receive chemotherapy for subsequent recurrences, and analysis of overall survival compare immediate with delayed chemotherapy rather than chemotherapy with observation. This bias does not apply to analyses of event-free survival, which stop at second recurrence. By performing such analysis, in this study Kempf-Bielack et al. (2005) were able to expand previous observations of a positive correlation between second-line chemotherapy and outcome to patients achieving a second surgical remission, suggesting an adjuvant effect, albeit limited, even in these heavily pretreated patients.
Ferrari et al. (2003) showed that in 162 patients with relapsed osteosarcoma, chemotherapy was given when surgical remission was not achievable or when pattern of disease recurrence suggested a particularly aggressive tumor. This study concluded that for the majority of patients in whom surgical remission was achieved, the use of second-line chemotherapy did not affect survival. However, for those patients who were not able to achieve surgical remission, the use of chemotherapy was found to increase survival.
Systemic Agents
Ifosfamide ± Etoposide
Ifosfamide is an oxazaphosphorine nitrogen mustard that has proven activity as a single agent against a variety of neoplasms, including bone (Carli et al. 2003). In adults, it is clinically active in advanced soft tissue sarcoma, with higher response rates achieved using higher doses. Interestingly, high-dose ifosfamide has also been proven effective in patients previously treated with conventional dose ifosfamide (Elias et al. 1990; Le Cesne et al. 1995; Palumbo et al. 1997; Patel et al. 1997). In a phase I and II combination studies with other agents, ifosfamide has significant activity in recurrent or metastatic osteosarcoma (Goorin et al. 2002; Miser et al. 1987), with objective responses reported in 30–50 % (Miser et al. 1987).
Since high-dose ifosfamide was found to have increased response rate in patients with osteosarcoma (Goorin et al. 2002), the 2.8 g/m2/day × 5 days with mesna has become widely used by pediatric oncologists. Often, etoposide is added at a dose of 100 mg/m2/day × 5 days (Chou et al. 2005). The response rate to ifosfamide and etoposide regimen is 38 % (Miser et al. 1987).
Ifosfamide can also be given as a prolonged 14-day infusion which was found to be well tolerated in pediatric patients with favorable toxicity profile, not requiring hospitalization for administration and thereby promoting patient’s QOL as well as decreasing cost. A response rate of 35 % in heavily pretreated patients is a promising finding, even in patients who were already previously treated with ifosfamide (Meazza et al. 2010). This study reported very low incidence of nausea, no encephalopathy or renal toxicity, low incidence of bladder toxicity, and reduction in cytopenias.
MTP-PE
Muramyl tripeptide phosphatidylethanolamine (MTP) is a nonspecific immunomodulator that is a synthetic analogue of a component of bacterial cell walls. Incorporation of MTP into liposomes has allowed targeted delivery of this agent to monocytes and macrophages in areas such as the lungs, activating these cells to be tumoricidal (Chou et al. 2009). Preclinical models in mice and canine osteosarcoma have confirmed antitumor effects of MTP (Anderson 2006; Asano and Kleinerman 1993; Kleinerman et al. 1993; MacEwen et al. 1989; Nardin et al. 2006). Moreover, concurrent administration of chemotherapy does not interfere with antitumor effects of MTP (Kleinerman et al. 1995; Meyers et al. 2005).
In a study by Meyers et al. (2005), patients with newly diagnosed metastatic osteosarcoma (n = 677) were randomly assigned to receive or not to receive ifosfamide and/or MTP in a 2 × 2 factorial design, in addition to standard chemotherapy (methotrexate, doxorubicin, and cisplatin). The 3y EFS for patients treated with standard arm of therapy, addition of MTP to standard therapy, addition of ifosfamide to standard therapy, and addition of both ifosfamide and MTP were 71 %, 68 %, 61 %, and 78 %, respectively. There was an observed interaction between the addition of ifosfamide and addition of MTP. From this study, it was concluded that the addition of MTP to chemotherapy might improve EFS. A follow-up analysis (Meyers et al. 2008) showed no evidence of interaction of ifosfamide and MTP, and both chemotherapy regimens resulted in similar EFS and OS. The addition of MTP to chemotherapy resulted in a statistically significant improvement in OS (6y OS increased from 70 to 78 %, p = 0.03) and a trend toward better EFS (p = 0.08).
An access study (MTP-OS-403, NCT00631631) (Anderson et al. 2014a) for MTP in patients with relapsed and/or recurrent osteosarcoma was done after initial approval by the European Medical Agency and at the request of the US Food and Drug Administration. This study also helped better define pharmacodynamics, pharmacokinetics, and safety profile of this drug in osteosarcoma. Resection of recurrence or metastases was encouraged but was not required for study entry. Concurrent chemotherapy was also allowed. Greater than or equal to grade 3 toxicity attributed to MTP was far less common than those attributed to chemotherapy. Among 50 patients whose disease was completely resected, >50 % remained alive more than 2 years from study entry. Many of these patients had already received ≥2 lines of therapy. MTP is currently available in Europe, Mexico, South Korea, Switzerland, and Israel for nonmetastatic osteosarcoma.
Gemcitabine/Docetaxel
Gemcitabine is a difluorinated deoxycytidine analogue, which is taken up by cells and depletes deoxynucleotide stores and interferes with DNA elongation and ultimately DNA synthesis. Docetaxel is a semisynthetic analogue of paclitaxel, a taxane, which stabilizes microtubules against depolymerization resulting in cell cycle arrest and apoptosis.
In phase 1 and 2 trials with gemcitabine alone (Reid et al. 2004; Wagner-Bohn et al. 2006), disease stabilization was reported in 4 of 13 patients with osteosarcoma. Modest responses in patients with Ewing sarcoma, rhabdomyosarcoma, and osteosarcoma have been observed with docetaxel as a single agent (Blaney et al. 1997; Seibel et al. 1999; Zwerdling et al. 2006). In a phase 2 trial of docetaxel (125 mg/m2 every 21 days) in 55 patients with recurrent bone and soft tissue sarcomas, there were 6 objective responses, 2 of which have underlying osteosarcoma (Zwerdling et al. 2006).
Combination of gemcitabine and docetaxel was proven to be synergistic in terms of antitumor activity against different cell lines, including osteosarcoma cells (Leu et al. 2004; Ricotti et al. 2003). In a retrospective case review, 22 patients with recurrent or refractory bone or soft tissue sarcoma were given gemcitabine (675 mg/m2 IV on days 1 and 8) and docetaxel (75–100 mg/m2 IV on day 8) (Navid et al. 2008a). Osteosarcoma was the most common diagnosis in this cohort (n = 18). Of the 14 patients evaluable for response, 3 patients with osteosarcoma achieved a partial response and 1 had stable disease. The overall objective response rate (CR+PR) was 29 %. Median duration of response was 4.8 months (1.6–13 months). Toxicity was manageable, primarily thrombocytopenia and neutropenia. The authors concluded that this combination demonstrated antitumor activity, especially against recurrent or refractory osteosarcoma. Response Evaluation Criteria in Solid Tumors (RECIST) showed shrinkage or stabilization of tumor in heavily pretreated patients with osteosarcoma and compared favorably with ifosfamide and cyclophosphamide/etoposide regimens (Miser et al. 1987; Rodriguez-Galindo et al. 2002 b; Van Winkle et al. 2005).
In a phase II trial through Sarcoma Alliance for Research Collaboration (SARC), gemcitabine (675 mg/m2 IV on days 1 and 8) and docetaxel (75–100 mg/m2 IV on day 8) were given every 21 days in children and adults with recurrent ES, osteosarcoma, or unresectable or recurrent chondrosarcoma (Fox et al. 2012). Out of 53 evaluable patients, there were no complete responses observed, and partial responses included 1 (out of 14) osteosarcoma, 2 (out of 14) ES, and 2 (out of 25) chondrosarcoma patients. For this study, gemcitabine and docetaxel combination was associated with the probability of reaching the target of 35 % response rate <5 % in osteosarcoma and 5.6 % in EWS.
14.1.5.4 Other Local Control Measures
Radiation Therapy (RT)
Traditionally, osteosarcoma has been considered a relatively radioresistant neoplasm. Viable tumor cells have been observed in surgical specimen after a dose of 60–70 Gy or more (Cade 1955; de Moor 1975). In contrast, there is evidence of a direct relationship between radiation dose and percent tumor necrosis (DeLaney et al. 2005; Gaitan-Yanguas 1981).
Delaney et al. (2005) reviewed 41 patients who received RT for osteosarcoma that was unresected or resected with positive margins. Local control was achieved in 78.4 % for patients with gross total resection, 77.8 % who had subtotal resection, and 40 % for those who had biopsy only. Control is also correlated with radiation dose, and a dose of >55 Gy achieved 71 % local control versus in <55 Gy only 53.6 % local control rate. This study concluded that RT can help provide local control of osteosarcoma for patients in whom surgical resection with widely negative margins is not possible. RT was specifically noted to be effective in situations in which microscopic or minimal residual disease is being treated.
Machak et al. (2003) determined the effectiveness of radiation therapy for local control of nonmetastatic osteosarcoma of the extremities after induction chemotherapy. Thirty-one patients refusing surgical local control were treated with neoadjuvant chemotherapy followed by standard fractionated external beam radiotherapy with median dose 60 Gy. The 5 year OS was 61 %, local progression-free survival 56 %, and metastases-free survival 62 %. Local treatment failure occurred median time of 18 months. As with DeLaney et al. (2005), the incidence of local relapse was higher when the dose was <50 Gy than those with >50 Gy. No definite correlation was found between the rate of local failure and regimen of fractionation. The outcome is correlated significantly with imaging and biochemical surrogate markers (alkaline phosphatase). Of note, survival probability was largely determined by whether a patient’s tumor responded to the induction of systemic therapy. In the COSS series (Kempf-Bielack et al. 2005), RT was almost exclusively administered to patients failing to achieve a second CR. RT was associated with prolonged survival only in the subgroup without a second CR (p = 0.0001).
Nowadays, there are multiple and advanced ways of delivering RT. Intensity-modulated RT (IMRT) has the potential to deliver higher doses to the tumor bed while sparing adjacent organs. This can be used for primaries in the pelvis, vertebrae, and chest wall. Another mode of delivering RT is with protons. Ciernik et al. (2011) demonstrated that high dose proton therapy allowed locally curative treatment for some patients with unresectable or incompletely resected osteosarcoma. After a median dose of 68.4 Gy, local control after 3 years and 5 years was 82 % and 72 %, respectively, with distant failure rate of 26 % after 5 years. In this study, the 5-year DFS was 65 % and OS 67 %. The extent of surgical resection did not correlate with the outcome. Risk factors for local failure were ≥2 grade disease (p < 0.0001) and total treatment length (p = 0.0008).
The effectiveness of the conventional, fractionated radiotherapeutic approach is probably limited by tumor cell repair of sublethal radiation injury and a relatively high fraction of hypoxic tumor cells (van Putten 1968; Weichselbaum et al. 1977). To overcome these causes, radiation is combined with various radiosensitizers (Kinsella and Glatstein 1987; Martinez et al. 1985). Radiotherapy combined with chemotherapy has been used in animal and human osteosarcoma to enhance local control over that achieved with radiation therapy alone (Caceres et al. 1984; Withrow et al. 1993). Concurrent use of ifosfamide during RT has been the standard of care for patients with Ewing sarcoma. Caceres (Caceres et al. 1984) was able to show complete tumor devitalization on 80 % of patients with osteosarcoma treated with high-dose methotrexate, doxorubicin, and 60 Gy of RT. Chemotherapy agents that combine systemic osteosarcoma control and also increase radiation effectiveness include ifosfamide, cisplatin, high-dose methotrexate, and gemcitabine (Leu et al. 2004). Gemcitabine, an active drug against a variety of sarcomas, is a potent radiosensitizer. Concentrations of 1,000-fold lower than typical plasma levels can be effective (Joschko et al. 1997; Lawrence et al. 1997), when given at least 2 hours prior to radiation with an effect lasting up to 48–60 hours after a dose (Wilson et al. 2006). Severe radiation recall is rare with gemcitabine compared to anthracyclines and taxanes, and might involve proinflammatory cytokine production (Friedlander et al. 2004).
There is evidence that chemotherapy markedly improves the effectiveness of local control using RT (Anderson 2003; Machak et al. 2003). In addition, there is also data that radiation dose required for adequate osteosarcoma control is less when combined with chemotherapy. In canine osteosarcoma, the radiation dose predicted to cause 90 % tumor necrosis when radiation was given with cisplatin was 36 Gy, but needed 50 Gy when radiation was given alone (Withrow et al. 1993). Mahajan et al. (2008) analyzed the exposure of 39 high-risk metastatic and/or recurrent osteosarcoma during consecutive 20 months. The median radiation dose was 30 Gy in 10 fractions. Chemotherapy was used in 80 % of radiotherapy courses. Their results confirmed that external beam radiotherapy with systemic treatment may provide a successful multimodality approach to local control and symptom relief.
In summary, RT can reduce the likelihood of positive margins and can be given after surgery if there are close or positive margins. RT is an option for patients with unresectable or incompletely resected tumors, for patients who refuse surgery, and for palliation of symptomatic metastases (DeLaney et al. 2005; Schwarz et al. 2009).
Thermal Ablation
The term “tumor ablation” refers to the destruction of tumor tissues in situ. The use of thermal extremes to ablate the tumor has been used widely in specific clinical scenarios. Lesions must be detectable using CT or ultrasound (>1 cm) and not so large (<5 cm) that heating and freezing uniformaly within lesion become difficult.
Radiofrequency ablation (RFA) is used to coagulate and destroy tumor tissue by direct application of radiofrequency (RF)-generated heat. An RF probe is placed into the tumor, and an RF generator is used to deliver high-frequency (375–480 Hz) alternating current to generate heat within the tumor to temperatures above 60 °C. After access is obtained, percutaneous delivery of thermal energy is used to destroy the tumor. The necrotic tissue is then resorbed and eliminated by the body. RFA requires anesthesia, but with short recovery time of 1–2 days (Rybak 2009).
Traditionally, symptomatic bone metastases have been addressed with chemotherapy and/or radiation with surgery reserved in cases of impending pathologic fracture. Not all lesions however are amenable to these first-line therapies. Lack of tumor sensitivity or an unacceptable risk of damage to adjacent organs may obviate the use of these treatment methods. For many patients, escalating use of opioids to treat pain may be too debilitating. In these cases, RFA may offer a minimally invasive alternative for local control of disease and pain palliation. The main goal of RF treatment of metastases for palliation is the complete ablation of the tumor interface with nearby tumor bone, as this is correlated directly with level of pain relief (Goetz et al. 2004; Simon and Dupuy 2006). In one study (Petsas et al. 2007), 95 % experienced decreased pain that was considered clinically significant.
RFA has been demonstrated to be an effective method, especially in lesions <3 cm in several adult malignancies. In a pilot study of 16 patients with recurrent pediatric solid tumors including 8 refractory osteosarcomas with lung metastases, Hoffer et al. (2009) was able to demonstrate feasibility and safety of RFA. In a later study (Saumet et al. 2014), 10 patients enrolled with osteosarcoma in French Society of Childhood Cancer centers had 22 treatment to lung metastases <3 cm and >1 cm from the large bronchi, trachea, and esophagus. Seven had complete remission at last follow-up (median 24 months after RFA), and none had recurrence at RFA sites. Eight sessions were done for bone lesions, and of three that were curative intent, all were in remission for >3 years. There are various other studies that showed RFA to be beneficial in alleviating pain from symptomatic metastases (Callstrom et al. 2006; Goetz et al. 2004; Rybak 2009; Santiago et al. 2009).
Side effects associated with RFA include immediate post-procedural hemoptysis and pneumothorax, as well as a paradoxic increase in pain up to the first week after RFA. Patients may have post-ablation syndrome, characterized by general malaise and fatigue believed to result from systemic release of cytokines caused by tumor cell death. Patients with lesions in the spine or in weight-bearing areas such as the acetabulum may be at risk for fracture.
On the other extreme, cryoablation involves freezing the tumor to below −20 °C. Cell death is induced by direct cellular injury involving formation of extracellular ice resulting in a relative imbalance of solutes between the intra- and extracellular environment, resulting in cellular dehydration and damage to cell membrane and enzymatic machinery (Baust and Gage 2005; Robinson et al. 2001). Primary use of cryoablation is in palliation of bone metastases (Corby et al. 2008) and primary bone tumors. Cryotherapy has also been used where definitive surgery would result in significant morbidity (i.e., hemipelvectomy for a large pelvic lesion) or may be used as a preliminary debulking measure thus allowing more limited surgical resection.
14.1.6 Emerging Targets and Therapies
14.1.6.1 Radionuclides
Samarium
Samarium-153 ethylenediamine tetramethylene phosphonic acid (153Sm-EDTMP) is a bone-seeking radiopharmaceutical designed to selectively deliver radiation to osteoblastic skeletal metastases (Anderson and Nunez 2007; Anderson et al. 2002). The radioisotope 153Sm emits an electron (beta particle) which induces targeted cytotoxicity. Its photon emissions help with scintigraphic imaging, thereby allowing both confirmation of agent localization and quantification of the absorbed dose delivered to target lesions.
153Sm-EDTMP has been used in the treatment of patients with high-risk recurrent or unresectable osteosarcoma. A phase I study found that up to 30 mCi/kg could be administered with autologous stem cell support; the only significant toxicity was myelosuppression (Anderson et al. 2002, 2005). In an effort to improve efficacy, Anderson et al. (2005) combined samarium with gemcitabine, a radiosensitizer. Although some patients achieved partial responses, none of these were durable. In a dose-finding study (Loeb et al. 2009) to see how much 153Sm-EDTMP can be given that would allow hematopoietic recovery within 6 weeks without use of stem cell support in patients with osteosarcoma bone metastases, the maximally tolerated dose of 153Sm-EDTMP was 1.21 mCi/kg with dose-limiting toxicity (DLT) confined to hematologic toxicity, mainly platelet recovery.
A phase 2 study reported safety and response of high-risk osteosarcoma to tandem doses of 153Sm-EDTMP (37–51.8 MBq/kg followed by 222 MBq/kg) and autologous stem cell rescue in a cohort of heavily pretreated patients (Loeb et al. 2010). Nine patients were enrolled and six had disease stabilization; however, this was not considered a response, so the study was terminated early. There was no correlation between positron emission tomography tumor uptake possibly from tumor inflammation and absorbed dose or time to progression. The median time to progression for the entire group was 79 days. Interestingly, one patient with disseminated lung and lymph node disease had a prolonged period of progression-free survival, and pulmonary nodules had >95 % necrosis of his pulmonary nodules. Another patient with pulmonary parenchymal disease also had disease stabilization. These cases support the idea that 153Sm-EDTMP is cytotoxic to extraosseous disease.
Aside from nonselective toxicity to the bone marrow, another limitation of 153Sm-EDTMP is the significant variability of tumor absorbed dose (Anderson et al. 2002, 2005; Loeb et al. 2009). To circumvent this problem, it would be ideal to administer an individually targeted tumoricidal absorbed dose of the pharmaceutical. Another option is to combine 153Sm-EDTMP with a vascular disrupting agent or a radiosensitizer. In addition, this may have limited efficacy for large tumors since a beta particle such as 153Sm-EDTMP has short path length, meaning that large areas of the tumor are inadequately treated. To augment the effect may need delivery of external beam radiation. Further investigation of this novel form for biophysically targeted therapy is warranted.
Radium
Another investigational bone-seeking radiopharmaceutical is radium (223Ra). Compared to 226Ra which was initially evaluated for internal radiotherapy, 223Ra has favorable decay characteristics, and radon daughter decay is rapid (4 s) providing much less of a chance of “off target” radon diffusion (Anderson et al. 2014b). Experience with 223Ra in phase I (Nilsson et al. 2005) and phase II trials in patients with metastatic prostate cancer confirmed excellent activity against bone metastases and a low toxicity profile (Nilsson et al. 2007). In a phase III prostate cancer trial with 809 patients, 223Ra significantly improved overall survival (p = 0.002) (Parker et al. 2013). It was also associated with low myelosuppression rates and fewer adverse events.
Radium should be suitable for use in combination with chemotherapy because of its higher therapeutic index (low bone marrow toxicity, higher effect on malignant forming cells) than 153Sm-EDTMP. There is currently an ongoing phase I dose escalation of monthly intravenous 233Ra dichloride in osteosarcoma (NCT01833520, clinicaltrials.gov).
14.1.6.2 Novel Delivery Systems
Inhaled Cisplatin (SLIT™ Cisplatin)
Inhalation therapy has potential advantages in the treatment of patients with pulmonary metastatic disease. Inhaled liposomal cisplatin has pharmacokinetic properties that maximize lung tissue delivery of cisplatin with minimal systemic exposure. Cisplatin is one of the most active agents in osteosarcoma and when encapsulated in liposomes limits its undesired side effects like nephrotoxicity, myelosuppression, ototoxicity, and nausea/vomiting (Wittgen et al. 2007). A study using inhaled lipid cisplatin (ILC) in 19 patients, administered via nebulizer every 2 weeks, and whenever possible metastasectomy was undertaken after 2 treatments (Chou et al. 2013). Eleven patients had bulky disease, and all progressed prior to cycle 7, while three of eight patients who had less bulky disease (<2 cm) had sustained benefit. This was well tolerated with only one patient experiencing ≥grade 3 respiratory toxicity.
Liposomal Doxorubicin
Liposomal delivery of doxorubicin allows for selective delivery of therapeutic agent to target tissues such as the lungs. The pegylated formulation of liposomal doxorubicin (Doxil) is a unique form of liposomal doxorubicin in which the liposomes are coated with ethylene glycol, resulting in a diminished uptake by the reticuloendothelial system, leading to a longer half-life in the blood and a different toxicity profile than nonpegylated liposomes (Ta et al. 2009). In a study of methylene diphosphonate (MDP)-conjugated adriamycin liposomes (MDP-LADMs), tumor growth and animal survival rates were evaluated after UMR106 osteosarcomas were established in Sprague–Dawley rats, and SOSP-M pulmonary metastatic osteosarcoma models were established in nude mice, respectively (Wu and Wan 2012). The toxicity assay revealed a significantly higher median lethal dose for MDP-LADMs than for free Adriamycin and LADMs (p < 0.05), and animal survival in the MDP-LADMs group was significantly higher (p < 0.05). These findings indicate that MDP-LADMs have higher therapeutic efficacy against osteosarcomas, demonstrate lower toxicity, and targeted osteosarcomas more clearly than the stand-alone systems.
In a phase II study of Doxil in previously heavily treated sarcoma patients (n = 47, 6 with osteosarcoma) considered unresponsive to chemotherapy (Skubitz 2003), a dose of 55 mg/m2 every 4 weeks was used which was well tolerated without documented cardiac toxicity. Three of the 47 patients received a CR or PR, although 15 of the 47 patients were felt to have derived clinical benefit from the treatment. These data suggest that pegylated liposomal doxorubicin has activity in this population of poor prognosis sarcoma and that this treatment is associated with modest toxicity. In a dose-finding study of temsirolimus 20 mg/m2 weekly and liposomal doxorubicin (30 mg/m2 monthly) for patients with recurrent and refractory bone and soft tissue sarcoma, this combination was shown to be safe for heavily pretreated sarcoma patients (Thornton et al. 2013). The phase II expansion portion of this study is ongoing.
14.1.6.3 Inhibition of Signaling Receptors and Transduction
Mammalian Target of Rapamycin (mTOR) Inhibitors
mTOR regulates protein synthesis and cell proliferation, survival, and angiogenesis. Ezrin is a protein with a role in cell–cell interactions and signal transduction and when upregulated drives metastasis (Hunter 2004). It appears to mediate its metastatic actions through the MAPK signaling pathway. Increased ezrin expression in pediatric osteosarcoma patients is associated with reduced disease-free intervals, and downregulation of ezrin expression in a mouse model of human osteosarcoma is associated with inhibition of pulmonary metastasis (Khanna et al. 2004). Rapamycin, an mTOR inhibitor, has been found to inhibit ezrin-mediated pathways leading to reduced lung metastases in a mouse model in osteosarcoma (Wan et al. 2005).
Response activity to rapamycin was high in one osteosarcoma xenograft (CR) and intermediate in five tumors (Houghton et al. 2008). Combination with cyclophosphamide or vincristine resulted in increased activity in osteosarcoma models (Houghton et al. 2010b). A randomized phase II trial of ridaforolimus as single-agent therapy met primary end point of improved PFS in two osteosarcoma patients (Chawla et al. 2012). Use of mTOR inhibitors in the treatment of osteosarcoma patients is under investigation as single-dose therapy (everolimus, NCT01216826; sirolimus, NCT01331135) and multi-agent therapy (sirolimus and cyclophosphamide, NCT00743509).
Multi-tyrosine Kinase Inhibitors (TKIs)
Tyrosine kinases (TKs) regulate cellular proliferation, survival, differentiation, function, and motility. Osteosarcoma has shown overexpression of several TKs (Rettew et al. 2012).
Src Kinase Pathway
Src is found to be overexpressed in osteosarcoma and mediates PI3K/Akt anoikis resistance (Diaz-Montero et al. 2006). Inhibition of Src prevents cell invasion and induces apoptosis in vitro and reduces tumor growth in vivo (Akiyama et al. 2008). Dasatinib was tested against osteosarcoma xenograft panels and was found to have intermediate activity (Kolb et al. 2008). Hingorani et al. (2009) demonstrated dasatinib to be active in primary osteosarcoma tumor, but not against pulmonary metastasis suggesting that this process may be independent of Src activation. There is a phase I/II study (NCT00788125) of dasatinib in combination with ifosfamide, carboplatin, and etoposide in young patients with metastatic or recurrent solid tumors including osteosarcoma. SARC has a randomized trial using Src inhibitor saracatinib in patients with recurrent osteosarcoma localized to the lung (NCT00752206).
Sorafenib
Sorafenib is an oral multi-TKI that targets Raf kinases and other RTKs involved in tumor progression (FLT-3, KIT, fibroblast growth factor receptor, RET) and angiogenesis (VEGFR and PDGFR-β). Several of these molecular targets are involved in the pathogenesis of osteosarcoma: Raf (Ikeda et al. 1989), PDGF (Sulzbacher et al. 2003), VEGF (Yang et al. 2007), and KIT (Smithey et al. 2002). Sorafenib has been shown to inhibit ERK1/2, MCL-1, and phospho-ezrin/radixin/moesin (P-ERM) pathways which are activated in osteosarcoma cell lines (Pignochino et al. 2009).
Insulinlike Growth Factor-1 Receptor (IGF-1R) Inhibition
IGF-1R has been implicated in the development of sarcomas, and inhibition of IGF-1R function has been demonstrated to reduce growth in osteosarcoma, ES, and rhabdomyosarcoma (Scotlandi 2006; Toretsky et al. 1997b). More than 25 antibodies and small molecules that specifically inhibit IGF-1R have undergone preclinical and clinical testing in both adults and children. Two humanized monoclonal antibodies (mAb) have been evaluated by the Pediatric Preclinical Testing Program (PPTP). Preliminary evidence of antitumor activity was reported in a phase II study of SCH717454 in subjects with relapsed osteosarcoma and Ewing sarcoma (NCT00617890). The mAb cixutumumab (IMC-A12) has been shown to induce intermediate responses in three of five osteosarcoma xenografts (Houghton et al. 2010a). In a phase II COG trial, cixutumumab was well tolerated as a single agent in patients with solid tumors (Malempati et al. 2012). In the phase II study which included patients with osteosarcoma, the combination of cixutumumab and temsirolimus showed clinical activity in patients, but did not have an effect on median progression-free survival (Schwartz et al. 2013). Despite evidence of benefits in some pediatric patients, several drug companies have curtailed or stopped anti-IGF-1R programs because observed clinical benefits did not meet primary end points in many adult trials.
14.1.6.4 Altering Tumor Microenvironment
Receptor Activator of Nuclear Factor-Kappa B Ligand (RANKL) Inhibitors
Zoledronic acid (ZA) is a potent third-generation bisphosphonate which targets the microenvironment of the bone, improves bone strength, and reduces tumor-related pain and skeletal-related events in several adult cancers through inhibition of osteoclast activity and bone resorption. It is approved by the US FDA in adults with solid tumors and bone metastases (Lipton et al. 2003; Rosen et al. 2003).
Preclinical studies suggest that ZA has direct antitumor activity in a variety of tumors, including osteosarcoma. ZA has been shown to inhibit primary tumor growth, reduce lung metastases, and prolong survival in animal models of osteosarcoma (Dass and Choong 2007; Labrinidis et al. 2009; Ory et al. 2005). The COG has completed a feasibility and dose discovery analysis of ZA with concurrent chemotherapy in the treatment of newly diagnosed metastatic osteosarcoma (Goldsby et al. 2013).
Denosumab is a fully human monoclonal antibody targeting receptor activator of the nuclear factor-kappa B ligand (RANKL) which interacts with its receptor RANK to regulate bone turnover. It is approved by the FDA for the prevention of fracture in postmenopausal women with osteoporosis, for prevention of skeletal-related events in adults with solid tumor bone metastases, and for giant cell tumor of the bone (Ellis et al. 2008; McClung et al. 2006). RANKL and its receptor RANK have the physiologic function of regulating bone turnover. In response to RANKL–RANK binding, osteoclast precursors differentiate and become activated resulting in bone resorption. RANK is expressed in 57 % of human osteosarcomas, most human osteosarcoma cell lines and 70 % of canine osteosarcomas (Barger et al. 2007; Mori et al. 2007). In osteosarcoma, RANKL activates downstream signaling and modulates gene expression (Akiyama et al. 2010). A phase II single-arm study using denosumab in recurrent osteosarcoma is currently being developed by COG.
Inhibition of Angiogenesis
Angiogenesis is closely related to tumor development and metastasis, as is seen in osteosarcoma. Endostatin is a broad-spectrum angiogenesis inhibitor. Endostar, a human recombinant endostatin, enhances the antineoplastic effects of combretastatin, a vascular disrupting agent in an osteosarcoma xenograft (Fu et al. 2011). In a trial to evaluate the clinical efficacy of Endostar (Xu et al. 2013), a human recombinant endostatin is combined with chemotherapy in the treatment of 116 newly diagnosed osteosarcoma patients. Immunohistochemistry was used to measure VEGF and CD31 expression. Chemotherapy was noted to increase VEGF expression and the presence of microvessels in osteosarcoma tissues compared with pre-chemotherapy. Although no significant difference was observed in the OS rate between the Endostar treatment and nontreatment groups, Endostar treatment significantly inhibited the chemotherapy-induced VEGF expression and the presence of microvessels. The Endostar group had a higher increase in the event-free survival rate and decreased the occurrence of metastases.
14.1.6.5 Other Targets
Anti-mitotic Activity
Eribulin is a fully synthetic analogue of halichondrin B, which is capable of inducing irreversible mitotic blockade and apoptosis by inhibiting microtubule dynamic instability, which differs from the mode of action of both vinca alkaloids and taxanes (Jordan and Wilson 2004). In an initial testing by the Pediatric Preclinical Testing Program (PPTP), eribulin induced significant differences in EFS distribution compared to control in 29 of 35 (83 %) of the solid tumors. Objective responses were observed in 18 of 35 (51 %) solid tumor xenografts, including complete responses (CR) or maintained CR that were observed in osteosarcoma xenografts (Kolb et al. 2013). A single-arm phase II study using eribulin for recurrent osteosarcoma is currently open to accrual (COG AOST1322).
Targeting Disialoganglioside (GD2)
Disialoganglioside GD2 is a sialic acid containing glycosphingolipid expressed in many tumor surfaces. Targeting of disialoganglioside GD2 with immunotherapy has resulted in improved outcomes for patients with neuroblastoma. It is highly expressed by osteosarcoma cells (>90 %) and thought to play an important role in the attachment of tumor cells to extracellular matrix proteins (Shibuya et al. 2012; Yu et al. 1998). Expression of GD2 may confer worse prognosis. In a report by Roth et al. (2014), osteosarcoma tissue obtained at the time of disease recurrence demonstrated a higher intensity of staining compared with samples obtained at initial biopsy and definitive surgery (p = 0.016). In another study, results suggested that expression of GD2/GD3 is responsible for the enhancement of the malignant features of osteosarcomas (Shibuya et al. 2012). These findings support GD2 as a great candidate for molecular-targeted therapy in osteosarcoma. Currently, there are ongoing trials targeting GD2 in patients with osteosarcoma (anti-GD2 CAR NCT02107963, humanized anti-GD2 antibody NCT00743496).
Targeting Glycoprotein Nonmetastatic Melanoma Protein B (GPNMB)
GPNMB is a transmembrane glycoprotein highly expressed in various types of cancer. It promotes the migration, invasion, and metastasis of tumor cells. Glembatumumab is an antibody–drug conjugate targeting GPNMB which has entered clinical evaluation for adult cancers that express GPNMB, including melanoma and breast cancer (Zhou et al. 2012). PPTP found that, among all xenografts, GPNMB was primarily expressed on osteosarcoma xenografts, all of which expressed GPNMB at the RNA level (Kolb et al. 2014). Glembatumumab induced statistically significant differences (p < 0.05) in EFS distribution compared to control in each of the six osteosarcoma models studied. Glembatumumab yielded high-level activity against three of the six osteosarcoma xenografts, two of which showed the highest GPNMB expression at the RNA level, an evidence for response being related to GPNMB expression levels.
14.1.7 The Future for Osteosarcoma
Osteosarcoma demonstrates high genetic instability, tumor heterogeneity, local aggressiveness, and early metastatic potential, all of which contribute to the aggressiveness of this disease. Hence, understanding the biology of osteosarcoma is of paramount importance in the design of therapeutic trials that will hopefully impact the survival of patients, especially the ones with metastatic and recurrent disease. In the past, hypothesis failure of several early phase trials was due to lack of clinical activity of tested novel agents against osteosarcoma. Metastasis is a consistent feature of osteosarcoma biology that is responsible for patient mortality, and shortcomings in our understanding of the biology of osteosarcoma metastatic process preclude any judgments on the value of agents that target metastatic process.
It is clear that there is a need for changes in the trial design which should be specifically curtailed to the underlying biology of osteosarcoma. The traditional phase II study design assesses radiographic response in patients with measurable disease. This end point as a means to evaluate efficacy of treatment is difficult in patients with recurrent osteosarcoma as pathologically responding lesions may not change in size radiographically owing to mineralization of tumors with treatment, leading to a conclusion that investigational treatment is ineffective in error. In addition, many patients with recurrent osteosarcoma may not be considered for phase II agents if all sites of disease recurrence are amenable to surgical resection. Hence, these patients frequently have complete resection without a trial of second-line chemotherapy, excluding them from a phase II trial and limiting the number of patients available to investigate novel treatment strategies. Novel biologic therapies may not be effective in patients with gross residual disease, but might improve outcome in patients with minimal residual disease after surgical excision.
Ongoing biology efforts through pediatric consortium studies are underway, and tumor specimens from this bank have been used as part of the NCI Therapeutically Applicable Research to Generate Effective Treatments (TARGET) initiative and NCI-led genome-wide association study. Preclinical drug evaluation systems created toward more rapid identification and validation of compounds active in osteosarcoma have been established such as the Pediatric Preclinical Testing Program in the United States and Innovative Therapies for Children with Cancer in Europe. High-throughput screens of novel agents should accelerate clinical trial development. Finally, the clinical infrastructure is primed, and there are now various national and international cooperative groups to efficiently test new agents.
14.2 Recurrent Ewing Sarcoma
14.2.1 Incidence of Recurrence
Ewing sarcoma (ES) is an aggressive tumor whose prognosis is critically determined by the adequacy of local control of primary lesion through surgery, radiation, or both and by the efficacy of the systemic chemotherapy aimed at the control of micrometastatic disease. Multimodal approach to the treatment has led to the dramatically improved outcome of patients with localized ES. However, recurrences remain a daunting challenge with dismal survival rate after relapse despite best efforts. Approximately 30–40 % of patients with initially localized disease at diagnosis still died of their disease (Burgert et al. 1990; Craft et al. 1998; Nesbit et al. 1990; Nilbert et al. 1998; Oberlin et al. 1985, 2001; Paulussen et al. 2001). About 60–80 % of patients with metastatic disease at initial diagnosis will relapse (Leavey and Collier 2008).
Most patients recur within 2 years from initial diagnosis (Bacci et al. 2006; Barker et al. 2005; Leavey et al. 2008; McTiernan et al. 2006; Shankar et al. 2003; Stahl et al. 2011). Patients diagnosed initially with metastatic disease relapsed significantly earlier compared to those who relapsed with initially localized disease at diagnosis (median time to recurrence 434 days versus 563 days, respectively, p < 0.001). The overall median survival after first relapse was 9–14 months (Bacci et al. 2006; Barker et al. 2005; Leavey et al. 2008; McTiernan et al. 2006; Shankar et al. 2003; Stahl et al. 2011). It was noted that in subsequent relapses, there was a higher rate of patients who relapsed with disseminated disease at multiple sites versus local recurrences (p < 0.0001) (Bacci et al. 2006). In 714 patients with first recurrence treated by Cooperative Ewing Sarcoma Studies (CESS) and European Intergroup CESS (EICESS 92), the patterns of relapse were as follows: 15 % local, 12 % combined systemic and local, and 73 % systemic. Pattern of relapse seems to change according to the stage of disease at presentation. In this series, among patients who were initially diagnosed with localized disease, 20 % relapsed locally, 73 % had systemic relapse, and 12 % had combined local and systemic relapse. When primary disease was disseminated at initial diagnosis, systemic relapse was seen in 82 %, 13 % combined, and 5 % localized relapse (Stahl et al. 2011). Barker et al. (2005) reported that among 71 % metastatic relapse without associated local relapse, the most common sites were the distant bone (35 %) and lung (18 %), with isolated recurrences occurring in 11 % of patients.
14.2.2 Outcomes at Recurrence
Whereas current intensive multimodal treatment enables patients with localized disease to be cured, in those with metastatic spread or recurrent disease, its benefit is more often limited to extending progression-free survival (Esiashvili et al. 2008; Leavey and Collier 2008). The outcome for patients with initially metastatic disease remains poor with 5 year EFS 28 % (Miser et al. 2007). The outlook for patients with recurrent EWS is even worse at less than 20 % in most series (Bacci et al. 2003, 2006; Barker et al. 2005; Cotterill et al. 2000; McTiernan et al. 2006; Robinson et al. 2013; Rodriguez-Galindo et al. 2002a Shankar et al. 2003; Stahl et al. 2011) despite aggressive multimodal treatment. Patients with recurrent disease have a reported 5-year post-relapse survival (PRS) of 7–23 % (Bacci et al. 2003, 2006; Barker et al. 2005; Leavey et al. 2008; Shankar et al. 2003) and 5-year post-relapse EFS (PREFS) of 7.9–13.8 % (Bacci et al. 2003, 2006). In a single-center case series of 114 patients, only 12.3 % remained alive at a median follow-up of 61 months (Whelan et al. 2012). In a retrospective study by Bacci et al. (2003), of 195 patients with relapsed ES, a second complete remission was achieved in only 26 patients (13.3 %). Of the 169 patients who never achieved disease-free status, 164 died of disease. Among patients who entered remission (n = 26), 12 relapsed once again with none of these patients achieving CR3, and all died of the disease. In the end, 14 patients were alive and free of disease. With a mean follow-up of 9.5 years, the 5-year PR EFS and OS were 9.7 % and 13.8 %, respectively. This study’s outcome was worse than reported in St. Jude’s cohort (Rodriguez-Galindo et al. 2002a) which included patients with metastatic disease at initial diagnosis, with a 5 year PRS of 23.7 % (n = 71) and similar to the UKCCSG ET-2 study (n = 64) with localized ES who had relapsed (Craft et al. 1998). Another study by Bacci et al. (2006) with 290 relapsed ES patients, the 5-year PREFS and PRS 5.1 % and 7.9 %, respectively. The median follow-up was 16.8 years from the start of primary treatment and 13.6 years from first recurrence. This is similar to ones reported by Craft et al. (1998), Oberlin et al. (1992), and Sauer et al. (1987). In a separate series with 114 patients with relapsed or progressive ES, the 2- and 5-year PRS were 23.5 % and 15.2 %, respectively (McTiernan et al. 2006). For all patients with recurrent ES, the 5-year PFS rate was estimated to be 20 % and 5 year PRS 23 % (Barker et al. 2005).
14.2.3 Risk Factors for Recurrence
The Children’s Oncology Group (COG) phase III multi-institutional study (INT0091) showed that older age, tumor size, primary site, advanced tumor stage, and serum LDH were all associated with decreased EFS and risk of recurrence (Grier et al. 2003). This was similar to those in previous studies of Ewing sarcoma (Cotterill et al. 2000; Oberlin et al. 1992; Meyer et al. 1992). Specifically, patients with large tumors (maximal diameter of at least 8 cm) had a poorer outcome than those with smaller tumors (5 year EFS 55 % versus 75 % percent, p < 0.001). The site of the tumor was also correlated with the outcome with the 5 year EFS 68 % among patients with tumors of the distal extremity, 61 % among patients with tumors of the proximal extremity, and 50 % among those with primary tumors of the pelvis (p = 0.003).
Grier et al. (2003) reported that younger patients had a better outcome than older patients. The 5 year EFS was 70 % for patients under 10 years, 60 % for those 10 years to 17 years, and 44 % for those 18 years or older (relative risk as compared with those under 10 years was 2.5; p = 0.001). Interestingly in this study, even though ifosfamide and etoposide benefited patients with localized ES, older age remained an adverse prognostic factor despite this addition. Other studies also supported older age (>14 years) being associated with poor outcome (Cotterill et al. 2000; Kolb et al. 2003; Siegel et al. 1988; Picci et al. 1997). The explanation of this is unclear. Some series suggest no differences between age-groups in terms of metastasis at diagnosis, tumor location, or histological response to neoadjuvant chemotherapy (Verrill et al. 1997; Bacci et al. 2007). However, there is a higher incidence of increased tumor size and extraskeletal primaries among patients >40 years (Pieper et al. 2008; Maki 2008). Older patients (>18 years) were also noted to have not benefited from compressed cycles of vincristine, Adriamycin, and cyclophosphamide alternating with ifosfamide and etoposide (VAD/IE) every 2 weeks instead of 3 weeks (Womer et al. 2012). In the study by Bacci et al. (2000), it is interesting to note that some of the factors that influenced initial EFS also impacted the pattern of relapse and the length of survival. For instance, in this series, in patients less than <12 years who relapsed, time to relapse and time to death were significantly longer than patients who are older than 12 years when their disease recurred.
Overt metastatic disease at diagnosis is established as one of the strongest indicators of poor outcome (Cotterill et al. 2000; Grier et al. 2003; Bacci et al. 2000). Initial pretreatment serum LDH correlates with tumor burden and has also been shown to be an adverse prognostic factor for outcome in ES (Bacci et al. 2000, 2003). Pathologic assessment of response to therapy involves evaluation of the treated tumor for degree of necrosis similar to what is done for osteosarcoma. There is general agreement that a complete response (inability to detect viable tumor cells with extensive sampling) is associated with better outcome than residual gross disease in the resection specimen (Picci et al. 1993, 1997; Bacci et al. 2000). Five-year EFS was 77.2 % and was noted for patients with good histologic response versus only 28 % for poor responders (p < 0.001) (Bacci et al. 2000). Moreover, patients who had good histologic response who relapsed and died of their cancer had significantly longer survival time versus poor responders (p = 0.03). However, histologic response to chemotherapy is hard to be properly defined as a prognostic factor because it is not assessable for patients who did not undergo surgery for local treatment. Other studies show conflicting data with regard to impact of tumor necrosis with post-relapse survival (Bacci et al. 2003, 2006).
14.2.4 Prognostic Factors at Recurrence
14.2.4.1 Time to Recurrence
Several studies have documented that outcome is significantly correlated with time to relapse, with patients who relapsed early (within 2 years of initial diagnosis) having worse prognosis compared to those who experienced late recurrence (≥2 years after initial diagnosis) (Shankar et al. 2003; Leavey et al. 2008; Bacci et al. 2006; McTiernan et al. 2006; Stahl et al. 2011; Barker et al. 2005; Robinson et al. 2013). There was an observed significantly better outcome in patients who had late versus early relapse, with 5 year PRS 14 % versus 7 % (p < 0.001), respectively, and PREFS was 5 % versus 19 % (p < 0.003) for early versus late relapse, respectively (Bacci et al. 2003). In a separate study, 5-year PREFS is significantly correlated with time to first relapse (p < 0.0009) (Bacci et al. 2006). In a study by Leavey et al. (2008), the disease-free interval (DFI) was shown to be significantly associated to the risk for post-recurrence death, with 5 year EFS of those who recurred >2 years from initial diagnosis 30 % versus 7 % for those whose disease recurred <2 years (p < 0.0001). The DFI for those who relapsed >2 years is 23 months, 1–2 years is 10 months, and <1 year is 5 months. Robinson et al. (2013) reported that time to relapse was a significant predictor of 5 year PRS, with early relapse (<2 year) at 12 % versus late relapse (>2 year) at 50 % (p = 0.003). In this study, those who had late relapses also had a greater PREFS than those who relapsed early (16 % versus 0 %).
Disease-free interval in between relapses is noted to be shorter with every relapse. Bacci et al. (2006) reported that the mean time to relapse was 27 months since the start of primary treatment, 17.6 months for second relapse, 13.7 months to third relapse, and 4 months for those who experienced fourth relapse. Moreover, the time to first relapse was significantly longer for patients who achieved second remission after treatment than for those who were never free of disease (52 months versus 23.5 months, p < 0.0001) (Bacci et al. 2003). This association in terms of time of recurrence is similar to findings in other studies (Nesbit et al. 1990; Oberlin et al. 1985; Paulussen et al. 2001; Rodriguez-Galindo et al. 2002a; Schuck et al. 2003).
14.2.4.2 Location of Recurrence
Local Versus Distant Relapse
Shankar et al. (2003) reported no difference in OS between local and systemic relapse and site relapse. In a study by Robinson et al. (2013), the 5 year PRS (35 %) was similar in all patients with distant relapse (lung-only metastases and other patterns of failure). In this study, however, the 5yearear PRS for those with localized relapse was significantly better than distant relapse (55 % versus 22 %, p = 0.045). Patients with combined relapse (local and distant) fared worse with a 5 year PRS for combined recurrence that was 12.5 % ± 8.3 % versus 21.7 % ± 7.8 % for those with localized relapse (Rodriguez-Galindo et al. 2002a). This is similar to a report by Stahl et al. (2011).
Isolated Lung Recurrence: Unilateral Versus Bilateral
Leavey et al. (2008) reported that 30 % of relapsed patients recurred in the lungs, but only 17 % had isolated pulmonary recurrence. In this cohort of patients, pulmonary recurrence was not predictive of post-recurrence survival (PRS), with isolated pulmonary recurrence not shown to be a statistically significant advantage compared with combined pulmonary recurrence (17 months versus 9 months, p = 0.08). This is in contrast to findings by Bacci et al. (2003) and McTiernan et al. (2006) who reported that for those with lung-only recurrence, those with bilateral disease has a significantly worse outcome compared to those with unilateral lung recurrence.
Recurrence in the Lungs Versus Other Sites
Some studies did not show a difference in the outcome of patients who had lung recurrence compared to other distant sites (Shankar et al. 2003; Robinson et al. 2013), although this can be due to smaller sample size of these studies. On the contrary, several studies have reported improved survival in patients who had lung-only relapses versus those with combination of distant recurrences (Bacci et al. 2003, 2006; McTiernan et al. 2006; Stahl et al. 2011). Specifically, Bacci et al. (2003) reported a significantly better outcome in patients who had lung-only metastases versus the ones with combined lung and outside lung metastases with a 5 year PREFS 14 % versus 0.9 % (p < 0.0001). In McTiernan’s study (McTiernan et al. 2006), patients with local relapse or lung-only relapse fared better compared to those with extrapulmonary recurrence. Moreover, patients with disease confined locally or to the lungs at both diagnosis and relapse had superior survival to those with extrapulmonary disease at any time.
14.2.4.3 Treatment for recurrence
Treatment for relapsed ES varies because of the many different types of recurrences that occur (localized, systemic, or combined), the site of distant relapse (lung, bone, lung and bone, other sites), the number of pulmonary metastases, and the type of first-line local and systemic treatment performed. The treatment is consequently tailored on an individual basis. Depending on what patients received at diagnosis, those who have local recurrence typically have local treatment with surgery or radiation therapy (RT) with our without systemic therapy.
Local Control
Shankar et al. (2003) reported that patients who received multimodal treatment had significantly higher response rate, but outcome was no different to those who only received single modality treatment. In another study, outcome significantly correlated with treatment performed after relapse, with all patients free of disease treated with surgery alone or combined with radiation therapy or a second-line chemotherapy (Bacci et al. 2003). In this study, there was a significantly better outcome in patients who had definitive local control (surgery and/or radiotherapy) alone, or in combination with chemotherapy, versus the ones who had chemotherapy alone (15.4 % versus 0.9 % p < 0.0001). In a separate study, the rate of the first remission was significantly higher for patients treated with surgery alone (93.6 %) or combined with chemotherapy (91.6 %) than those treated with conventional (2.2 %) or high-dose chemotherapy (11 %) or by radiotherapy (22 %) (Bacci et al. 2006). This however needs to be interpreted with caution since patients treated with surgery alone likely had isolated local or lung recurrences, and the ones who were treated with chemotherapy alone were highly selected and restricted to patients with unresectable disease and not amenable for curative intent radiotherapy.
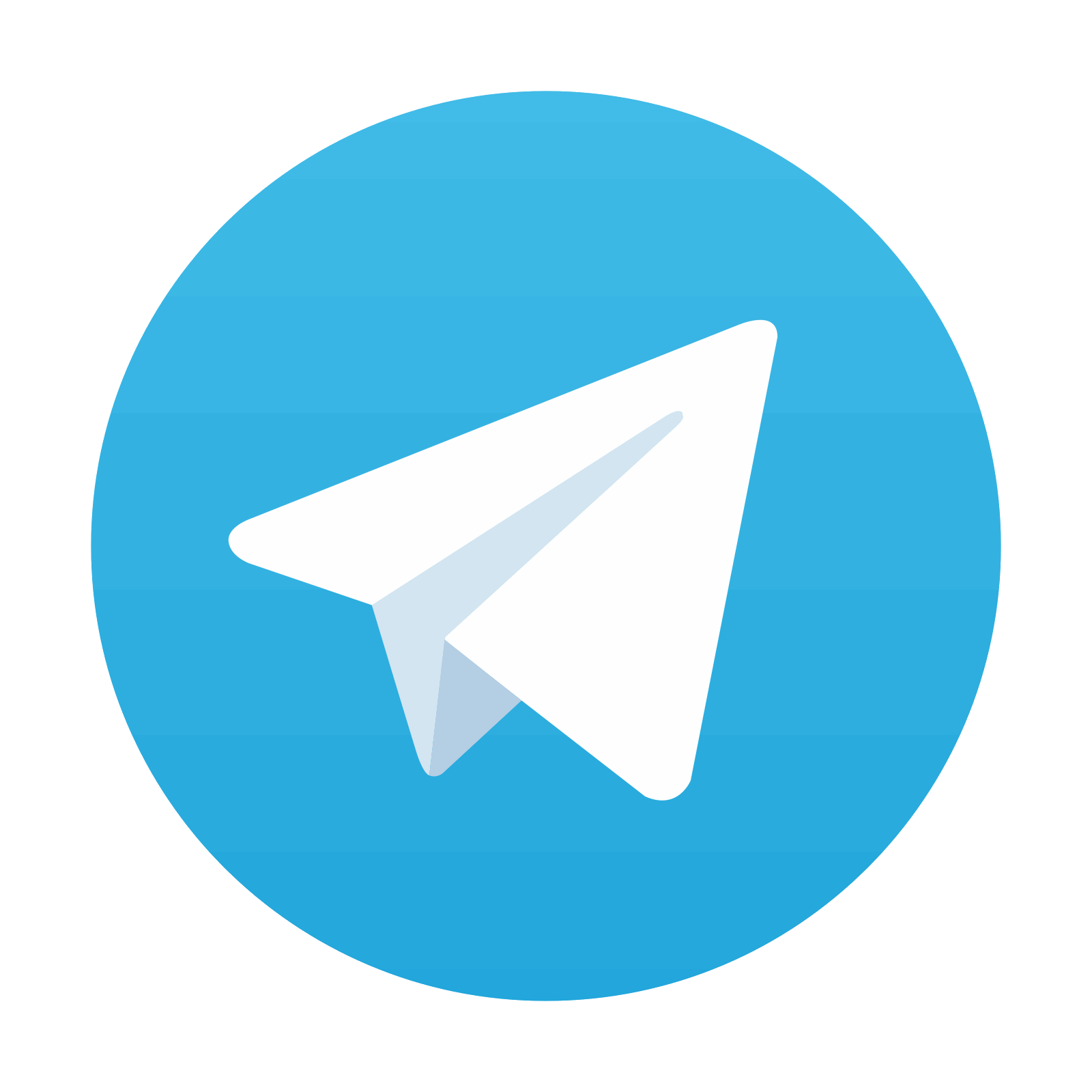
Stay updated, free articles. Join our Telegram channel

Full access? Get Clinical Tree
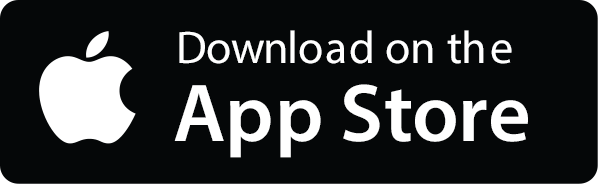
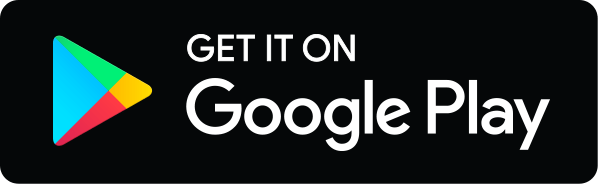
