Fig. 4.1
Fluorescence in situ hybridization of the nucleus from a single blastomere for chromosomes 13 (green), 16 (aqua), 18 (blue), 21 (red) and 22 (yellow), displaying trisomy 13 and trisomy 21 (Image courtesy of Professor Darren K Griffin, PhD, DSc, FIBiol, FRCPath, FRSA, School of Biosciences, University of Kent, UK)
In 1992, the molecular techniques that first allowed the gender determination of embryos were extended to look at specific disease causing genetic mutations. While the majority of groups continued to focus on blastomere biopsy, some undertook a combination of polar body and blastomere analysis – a position that was as much to do with legal implications as science. Cystic fibrosis was the first monogenic condition for which PGD was undertaken on human embryos to detect a specific disease causing mutation [18]. Much research had taken place on mouse embryos prior to this. The deltaF508 mutation is the highest frequency CFTR mutation and is a 3 base pair deletion. Thus a nested PCR of the region and gel electrophoresis allowed the detection of DNA homo- and heteroduplexes and the identification of affected, unaffected and carrier embryos. However, this technique was vulnerable to failed amplification of a specific allele, as the first gender selection cases had been. The introduction of multiplexing protocols allowed multiple linked markers to be used to follow disease inheritance. Subsequently, robust and accurate methods of whole genome amplification negated the need for multiplexing and greatly increased the number of linked markers that could be run and the reliability of testing [19, 20]. This also allowed more than one genetic condition to be tested for, or more commonly, to test for both disease and HLA type in embryos. These technologies were employed with little change to the diagnosis of monogenic disorders for many years. However, the advent of single nucleotide polymorphism (SNP) arrays promises to be the biggest paradigm shift in the field since its introduction and will ultimately allow parallel PGD and PGS in tandem via parallel test [21].
PGD for “De Novo” Mutations
PGD for “de novo” genetic mutations is a challenge, as the person affected and liable to transfer the genetic changes to the child, maybe the only person in the family affected by the mutation. This makes it difficult to test for the abnormality, as the genetic test will normally need other affected or carrier individuals in the family to establish inheritance and increase the robustness of the test used. Hence, PGD for ‘de novo’ mutations is available only in some IVF centres, and several different strategies such as, polymorphic marker evaluation, whole and single sperm testing to establish the normal and mutant haplotypes and PGD by polar body and/or embryo analysis, have been used by PGD providers [22]. Yet another successful strategy is to use the embryos created in an IVF cycle as another generation, allowing the genetic test to follow the mutation [23]. However, this may imply that more embryos have to be tested over more than one IVF cycle, before the diagnosis can be made.
PGD for Sex Selection
According to ESHRE consortium data collection from 2010, PGD for social sex selection was reported in 48 out of 5780 cycles [24]. Some parents undertake PGD to “balance” their family or to satisfy their gender preference [25], a practice that is deemed illegal in Europe, China and Australia. In the UK sex selection may only be performed where one gender is at risk of a genetic condition.
PGD for Chromosomal Rearrangement
Parents carrying a balanced translocation, whether it is reciprocal, Robertsonian or inversion, generally have no clinical symptoms, but carry a higher risk of recurrent miscarriages, recurrent implantation failure with IVF having children born with disability and sometimes infertility [26]. See Fig. 4.2.


Fig. 4.2
Diagrammatic representation of the possible inheritance patterns of a chromosomal rearrangement from a parent with a balanced translocation (Image courtesy of Illumina, Inc.)
Preimplantation genetic diagnosis for chromosomal rearrangements was first applied in the mid 1990s, using FISH probes specific to the chromosomal breakpoints that enabled the detection of unbalanced segregation patterns [3, 8]. However, FISH was unable to identify other aneuploidies leading to poor PGD outcome. This compromised the faith in using FISH for PGD in chromosomal rearrangements. Whilst there was a reasonable argument for natural conception being more successful in the same time frame as multiple PGD cycles [27], it failed to consider the impact of recurrent miscarriages.
The advent of array comparative genomic hybridisation (CGH) or single nucleotide polymorphism array (SNP) not only allowed the identification of unbalanced segregants but also allowed the identification of aneuploidy [4]. This has significantly contributed to higher pregnancy rates with lowered miscarriage rates. Whilst array based testing is expensive and relatively prohibits its wider usage, the latest technology called Next Generation Sequencing (NGS) is proving cost effective and accurate [28].
PGD for HLA Tissue Typing
The short arm of chromosome 6 holds a cluster of genes that encode the major histocompatibility complex (MHC) comprising the HLA family of genes. HLA genes encode cell surface proteins that play a crucial role in the immune response. Pre-implantation genetic diagnosis for HLA tissue typing enables the conception of a child who is HLA compatible with a disease-affected existing child. The baby’s cord blood is then used for stem cell transplantation or bone marrow transplant. The term “saviour sibling” is frequently used for such PGD treatment. HLA typing is often performed in conjunction with testing for a recessive monogenic disorder [29, 30]. Conditions such as β-thalassemia and Fanconi’s anaemia have been treated with HLA tissue typing [6] with a 3 in 16 chance of finding an unaffected and HLA matched embryo for transfer via PGD [30].
Preimplantation Genetic Screening
Chromosome anomalies are the major cause of unsuccessful IVF or pregnancy loss after IVF. The age related decline in natural and IVF birth rates is linked to increased aneuploidy rates, which contribute to, failed implantation, miscarriage and increased rates of Downs, Edwards and Patau syndromes. Whilst the rate of aneuploidy in women under the age of 36 years is around 55 %, this increases to around 90 % in women aged 43 years. Theoretically, then, the identification and negative selection of chromosomally abnormal embryos should mitigate the age effect in IVF and increase implantation and reduce miscarriage rates in patients of advanced maternal age [31]. Thus, PGS in patients with advanced maternal age, recurrent miscarriage and recurrent implantation failure was reported to improve success rates [32].
The introduction of FISH to single cell PGD was expanded to chromosome screening – PGS – in 1993. However, there were a number of issues with this strategy. Firstly, FISH could only test a limited number of chromosomes – generally between 5 and 8. The chromosomes tested were those associated with miscarriage and live birth, meaning many abnormalities, which would result in failed implantation, were undetected. Secondly, there were a number of technical artefacts affecting the reliability of the testing – loss of micronuclei during cell fixing, split signals, and overlapping signals. Thirdly, the incidence and impact of mosaicism was not fully understood. Published RCTs, 10 years after its clinical introduction showed no advantage from PGS, and even reduced success rates [33]. This was controversial at the time and hotly contended by initial pioneers of the treatment who continued to evidence improved success rates [34]. However, the controversy did lead to many changes in practice, most notably the introduction of comprehensive chromosome screening (CCS). Despite more recent RCTs and systematic reviews supporting the use of PGS when CCS is employed [35], the majority of the scientific community seem to remain sceptical, and, professional bodies including American Society of Reproductive Medicine the European Society of Human Reproduction and Embryology, and British Fertility Society have not changed their guidance to reflect this.
For some reason, human reproduction, among mammalian species, is characterised by a high rate of chromosomally abnormal gametes and embryos produced. Around 10–15 % of clinically recognised pregnancies end in first trimester miscarriage, which does not include occult or missed abortions. Transient implantation may occur with minimal disruption to the menstrual cycle and an individual might never realise it has occurred. More than half of these events, 60–80 %, are a result of aneuploidy in the embryo. Additionally, an even higher rate of aneuploidy exists at the embryo stage than detected in pregnancy given many chromosomally abnormal embryos will simply fail to implant. Thus, the rationale behind PGS remains sound and the advent of CCS has led to evidence that PGS is suitable to be employed as a method of embryo selection in good prognosis patients [35].
Potential Risks of PGD and PGS
There are general risks of IVF treatment namely, suboptimal or excessive ovarian response resulting in either low egg numbers or ovarian hyperstimulation syndrome, respectively. If there are fewer eggs, there is a lower chance of finding suitable embryos from a genetic and developmental perspective. Fertilisation and embryo development during IVF may be poorer than expected thereby limiting the number of blastocysts available on day 5 or 6 for biopsy and freezing.
In terms of risks that are specific for PGD, the risk of accidental damage to the embryo is quoted as less than 0.6 %. When an embryo does not yield a result, this is usually due to the embryo having been of poorer quality when biopsied or not having developed correctly. Embryos with a clear genetic result are deemed suitable for transfer. Couples should understand that there may not be a genetically or developmentally suitable embryo for transfer after IVF/PGD.
The misdiagnosis rate of PGD testing for a genetic disease is less than 3 % [36]. Several intrinsic, extrinsic and human factors have been reported to cause misdiagnosis. These include unprotected sex during treatment, mislabelled tube, misidentified embryo/slide/tube, misinterpreted report, transfer of wrong embryo, chromosomal mosaicism, parental/operator contamination, and allele drop-outs. Allele drop-outs may cause erroneous results owing to the failure of all genetic material to be amplified during PCR.
The error rate after PGS using CCS has been reported as low [37]. Adhering to robust quality control measures can reduce the misdiagnosis arising from the IVF and genetics laboratories. Additionally, it is vital that the couples are advised to use an effective form of contraception during treatment to avoid a natural conception. Couples are also strongly advised to undergo prenatal testing by chorionic villus sampling or amniocentesis on achieving a pregnancy though clinical experience shows few do proceed to invasive testing. Non-invasive prenatal testing is often preferred by couples in these circumstances as providing reassurance that the fetus is not affected by the common trisomies.
Uniparental disomy (UPD) is a condition in which both chromosome pairs in an embryo are derived from the same parent. Such an inheritance may disrupt the imprinting mechanism in the embryo thereby leading to the development of imprinting disorders such as Prader-Willi and Angelman syndrome [38]. The occurrence of UPD in human blastocysts is random and rare [39]. Couples undertaking PGD for chromosomal rearrangements should be counselled that UPD cannot be routinely diagnosed via PGD.
The Future of PGD and PGS: Karyomapping and Next Generation Sequencing
PGS has seen vast and regular changes in the technologies employed – from the advent of PGS via FISH, looking at a single data point on a limited number of chromosomes, to quantitative PCR looking at two to four data points per chromosome, array-based comparative hybridisation looking at hundreds of points per chromosome and NGS, looking at tens of thousands of data points per chromosomes. A large amount of sequence data can be generated using high-throughput NGS tools such as Illumina MiSeq DNA sequencing platform. Diagnosis of chromosomal rearrangements using NGS has been shown to improve the accuracy of detection of chromosomal abnormalities [28]. Additionally, reports have shown that both single gene disorder and chromosomal testing can be performed simultaneously on the same sequencing platform without the need for the pre-test workup of single gene disorders [40]. See Fig. 4.3.


Fig. 4.3
Results of chromosomal aneuploidy screening in an embryo by Next Generation Sequencing (NGS) – bottom image, compared to array Comparative Genomic Hybridization (aCGH) – top image. Values on the Y axis represent chromosome copy number, the image shown displaying a deletion on chromosome 3 and trisomy 5. The changes are more apparent in NGS as it is a quantitative method based on copy number counting, whereas aCGH qualitatively looks at relative fluorescence of sample and reference (Image courtesy of Genesis Genetics Europe)
Karyomapping is another new technology that has revolutionized PGD for monogenic disorders. Karyomapping, which uses SNP genetic haplotyping, works by identifying the differences in genetic markers between the couples. The aim is to differentiate the four parental copies of the gene an embryo may inherit and identify which are associated with the genetic mutation/disease. This is known as genetic linkage. However, the genetic information is “shuffled” as it is inherited. See Fig. 4.4.


Fig. 4.4
Using a reference of known genetic status, Karyomapping assigns maternal and paternal haplotypes – M1(yellow)/M2(green) and P1(blue)/P2(red). The figure represents a family with a father and child affected by a dominant genetic condition on chromosome 16p. Thus P1 can be identified as the affected allele and embryos 1, 4, and 8 diagnosed as affected and embryo 10 diagnosed as unaffected. The detailed haploblock chart show the whole of chromosome 16 for embryo 4 T. Along the top of the chromosome are the informative (key) SNPs and along the bottom are the non informative (non key) SNPS (Image courtesy of Genesis Genetics Europe)
Karyomapping examines the chromosomes at approximately 300,000 different points and finds a DNA fingerprint unique to the chromosome that carries the affected gene. It is then possible to test the fingerprint in the embryos produced by the parents, revealing those that have inherited the affected gene. If the fingerprint characteristic of the chromosome carrying the affected gene is not detected, then it can be inferred that the embryo has inherited normal copies of the gene and is therefore likely to be free of the disorder. The test will tell which copies of a gene, an embryo has inherited from each parent, rather than identifying genetic mistakes or mutations. As a bonus by-product, karyomapping also identifies any anomalies present in other chromosomes. Karyomapping will also identify some chromosome anomalies where, rather than seeing a maternal and paternal SNP at each point on a chromosome, or region of chromosome, only one, or three, SNPs are detected, indicating a monosomy or trisomy respectively.
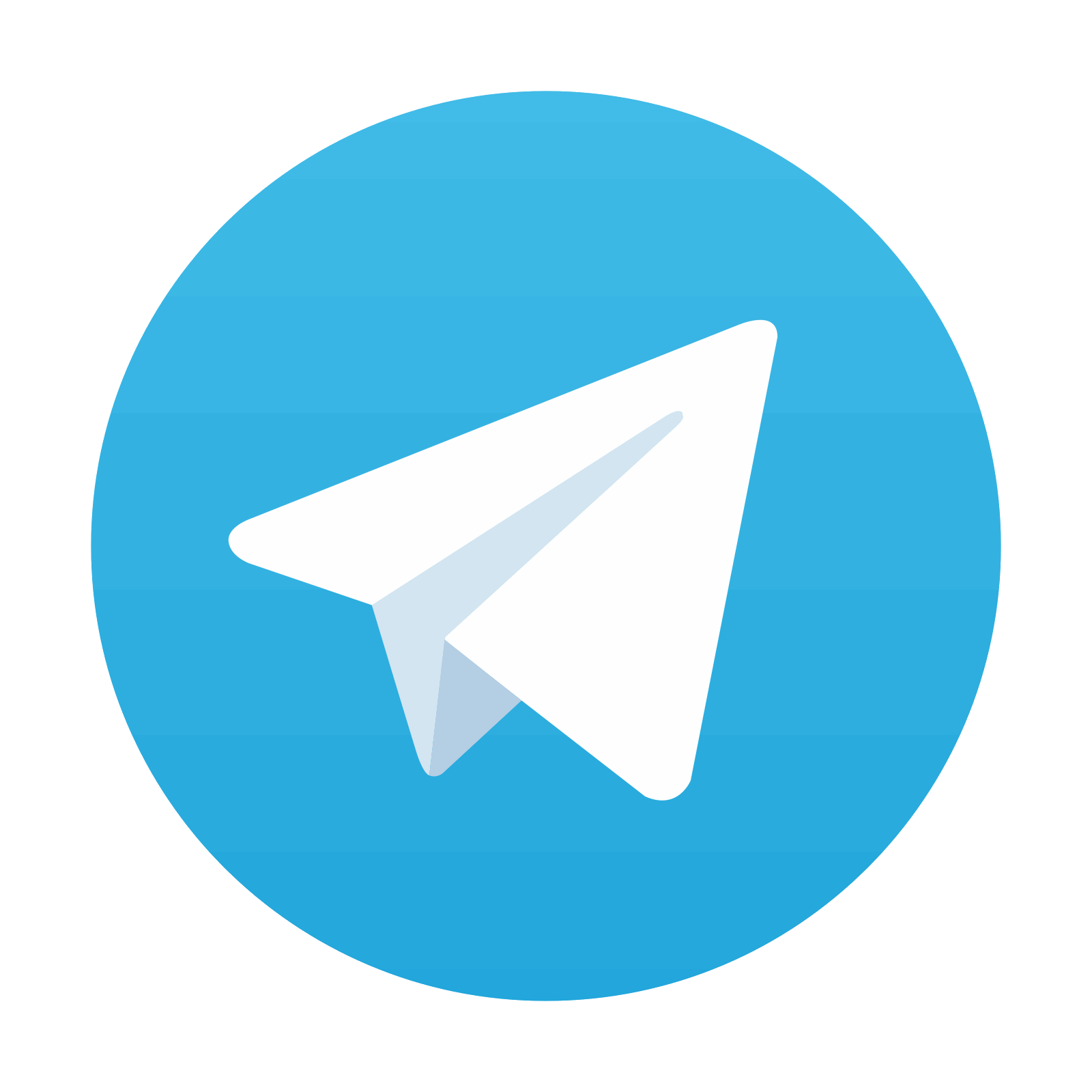
Stay updated, free articles. Join our Telegram channel

Full access? Get Clinical Tree
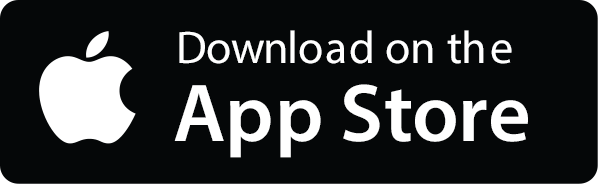
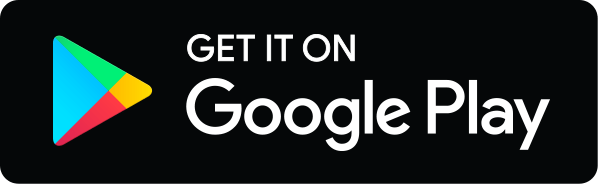