Fig. 25.1
Frequency distribution histogram of level of RBC TPMT enzyme activity in blood samples from 298 randomly selected Caucasian blood donors

Fig. 25.2
Common TPMT mutant alleles. Wild type (TPMT1) is the most common and associated with normal TPMT enzyme activity
Pharmacology of Thiopurines
6-MP and AZA are often used interchangeably in the treatment of IBD. Once absorbed, AZA is rapidly converted via a nonenzymatic process to 6-MP and S-methyl-4-nitro-5-thioimidazole. AZA is 55% of 6-MP by molecular weight and 88% of AZA is converted to 6-MP such that a conversion factor of 2.08 must be taken into account when calculating equivalent doses of 6-MP and AZA. 6-MP (or AZA converted to 6-MP) undergoes a complex biotransformation to its inactive and active metabolites via competing catabolic and anabolic metabolic pathways (Fig. 25.3). 6-MP undergoes extensive “first pass” catabolism by xanthine oxidase (XO), which is present in both the intestinal mucosa and liver limiting the systemic bioavailability of thiopurines. 6-MP also serves as a substrate for the TPMT enzyme, which methylates 6-MP to the inactive methylated-mercaptopurine metabolite (6-MeMP). Like XO, TPMT may be present in the intestinal mucosa and may also contribute to the pre-systemic catabolism of 6-MP. The hypoxanthine phosphoribosyl transferase (HPRT) enzyme is paramount to the initiation of the anabolic transformation of 6-MP to its active metabolites. The intracellular activation of 6-MP yields the first active intermediate metabolite, thioinosine monophosphate (TiMP), which is then rapidly converted to the thioguanine nucleotides (6-TGN). The cytotoxic and immunosuppressive effects of thiopurines, were, until recently, presumed to be primarily mediated via the incorporation of 6-TGN into cellular nucleic acids ultimately resulting in inhibition of lymphocyte proliferation. Recent advances in the understanding of the mechanism of action of thiopurines, however, suggest that the 6-thioguanine triphosphate (6-thio-GTP) nucleotide may play an important role in the process of signaling lymphocyte apoptosis by inhibiting Rac1 activation in T cells [21]. The triphosphate metabolite appears to correlate most with clinical efficacy of thiopurines [22]. Further research is needed to examine the role of 6-thio-GTP in patients with IBD.

Fig. 25.3
Thiopurine metabolism. Oral azathioprine (AZA) is rapidly converted to 6-MP by a nonenzymatic process [19]. Initial 6-MP transformations occur along competing catabolic (XO xanthine oxidase; TPMT thiopurine methyltransferase) and anabolic (HPRT hypoxanthine phosphoribosyltransferase) enzymatic pathways. Once formed, 6-thiosine 5′-monophosphate (6-TImP) may be transformed into 6-thioguanine nucleotides (6-TGN) by the rate limiting inosine monophosphate dehydrogenase (IMPDH) or methylated into 6-methyl-mercaptopurine nucleotides (6-MMP)
The major competing enzymatic process that competes with the intracellular activation pathway is the thiol methylation of TiMP by TPMT. Although these methylated metabolites (Me-TiMP or 6-MMPR) have been shown in vitro to inhibit denovo purine synthesis, in vivo evidence is lacking as to their role in the mechanism of action of thiopurines [6]. TPMT methylation competes with the activation pathway and influences the relative proportion of intracellular active 6-TGN produced by a given individual. This inverse relationship between TPMT and 6-TGN has important implications in both the efficacy and toxicity of thiopurines. Patients with intermediate or absent TPMT activity can produce significantly higher concentrations of 6-TGN. The negative correlation between TPMT and 6-TGN was first reported in the treatment of childhood leukemia [4] and has since been confirmed among pediatric IBD patients [23].
Physicians should also pay particular attention to potential drug interactions such that the inhibition of recombinant human TPMT has been demonstrated in vitro and has been reported in vivo with both 6-MP and olsalazine [24, 25]. Thus caution should be taken when co-administering 5-ASAs and thiopurines as TPMT inhibition may increase the risk of developing myelosuppression. A recent study demonstrated that after 5-ASA withdrawal the mean 6-TGN levels significantly decreased without significant changes in TPMT activity or blood counts suggesting an alternative mechanism to TPMT inhibition [26]. This effect may be additive in patients already genetically determined to produce lower levels of the TPMT enzyme such that diligent blood count monitoring is necessary.
The Clinical Application of TPMT Pharmacogenetics
As explained above, TPMT methylates thiopurine metabolites at the expense of the formation of 6-TGN. However, patients with less than normal TPMT expression and activity can produce significantly higher concentrations of 6-TGN. This inverse relationship between TPMT and 6-TGN has important implications in both the efficacy and toxicity of thiopurines. The association between TPMT and myelosuppression in IBD patients was published by Colombel et al. who reported that 27% of IBD patients experienced leukopenia and/or thrombocytopenia attributable to abnormal TPMT activity [27]. Moreover, TPMT deficiency (homozygote mutant) accounted for 10% of the cases and the median time to onset of bone marrow suppression was 1 month, whilst 17% of the cases were TPMT heterozygotes. In this study only 32% of the cases of myelosupression were secondary to lower TPMT activity. Thus patients should be regularly monitored with complete blood counts and secondary causes such as concomitant medications or concurrent viral illness should be considered. The a priori knowledge of an individual’s TPMT activity can identify those patients prone to early leukopenic events when treated with standard doses of thiopurines as a result of intermediate TPMT levels. Moreover, the 1 in 300 individuals at risk for potentially life-threatening complications can be rapidly identified and alternate immunomodulators can be administered. Intermediate TPMT activity is not a contraindication for thiopurine use and typically these patients can safely receive thiopurines at lower doses (30–50% of standard dose) and dose escalation can be successful while patients are monitored closely with regular blood counts and 6-TGN concentrations [28, 29]. For the majority of thiopurine recipients, clinicians can safely administer what is considered an optimal starting dose of 6-MP (1–1.5 mg/kg/day) and AZA (2.0–2.5 mg/kg/day) as a result of normal to high TPMT activity. Whether it be empiric or TPMT based dosing, patients require regular blood count and liver chemistry monitoring throughout the duration of thiopurine therapy.
It remains unknown as to the safety implications of having high TPMT activity. Elevated levels of 6-MMP have been shown to be associated with an increased frequency of hepatotoxicity as defined by elevations in transaminases >2× normal [23, 30]. Given that TPMT results in the formation of 6-MMP, it could be hypothesized that patients with high TPMT produce potentially hepatotoxic levels of 6-MMP and the first pass catabolism of the drug in the liver by higher than normal TPMT activity may lead to the accumulation of higher levels of 6-MMP. Liver enzyme elevations typically normalize in a timely manner in response to dose reduction as a result of a decrease in 6-MMP levels. Not all patients experience an elevation in transaminases in association with high 6-MMP levels and few merit a significant dose reduction; however, these patients should be closely monitored.
Pharmacokinetics: The Role of Metabolite Monitoring
The importance of metabolite monitoring and TPMT activity was first brought to our attention through the research initiated in childhood acute lymphoblastoid leukemia (ALL) [4]. Initial studies in IBD were conducted in the pediatric age group and reported that 6-TGN was shown to be significantly and independently associated with therapeutic response [23, 31]. This study demonstrated that significantly higher median 6-TGN levels were observed at points of clinical response (312) vs. nonresponse (199). Further analysis revealed that the best probability of treatment response was not significantly increased until 6-TGN levels were greater than 235 and the odds ratio (OR) of a therapeutic response for a 6-TG level higher than a cutoff of 235 was 5.0 as compared to when the levels were below this cut point. A certain percentage of patients did achieve a therapeutic response with levels <235; however, this response rate was almost less than half the proportion of patients (78%) achieving therapeutic response when levels were >235. Subtherapeutic dosing, or based on these studies, perhaps more appropriately subtherapeutic 6-TGN levels, is the most common reason why patients are not responding to thiopurines. Cuffari et al. demonstrated that most patients, initially not responding to low-dose thiopurine therapy that underwent dose escalation responded well once they achieved 6-TGN levels >250 [32]. A meta-analysis reported that patients with 6-TGN levels above the threshold value were more likely to be in remission (62%) than those below the threshold value (36%) (pooled odds ratio, 3.3; 95% confidence interval, 1.7–6.3; P < 0.001) [33].
Metabolite monitoring is most informative in helping clinicians classify therapeutic failures. Non-compliance and under dosing remain the most common reasons for therapeutic failure and can be identified by measuring 6-MP metabolite levels. When both 6-TGN and 6-MMP levels are undetectable, there is a high likelihood that the patient is not adhering to therapy. However, a subgroup of patients continues to fail therapy despite dose escalation and receiving standard or even higher than standard doses of thiopurines. These patients can be divided into two distinct subgroups and are defined biochemically by their metabolite profiles. The most common subgroup is composed of patients resistant to thiopurines and biochemically characterized by the persistence of subtherapeutic 6-TGN (<235) and the preferential shunting towards excessive potentially hepatotoxic 6-MMP levels upon 6-MP/AZA dose escalation [30]. Individuals unmask this metabolic profile when they are challenged with higher doses of the drug. There is a small group of refractory patients who, despite therapeutic (>235) and often potentially toxic 6-TGN (>450) levels, are unable to benefit from the immunosuppressive properties of these therapies. The identification of these two subgroups early on the course of therapy can significantly improve patient outcomes by moving patients quickly to alternative immunomodulator therapy.
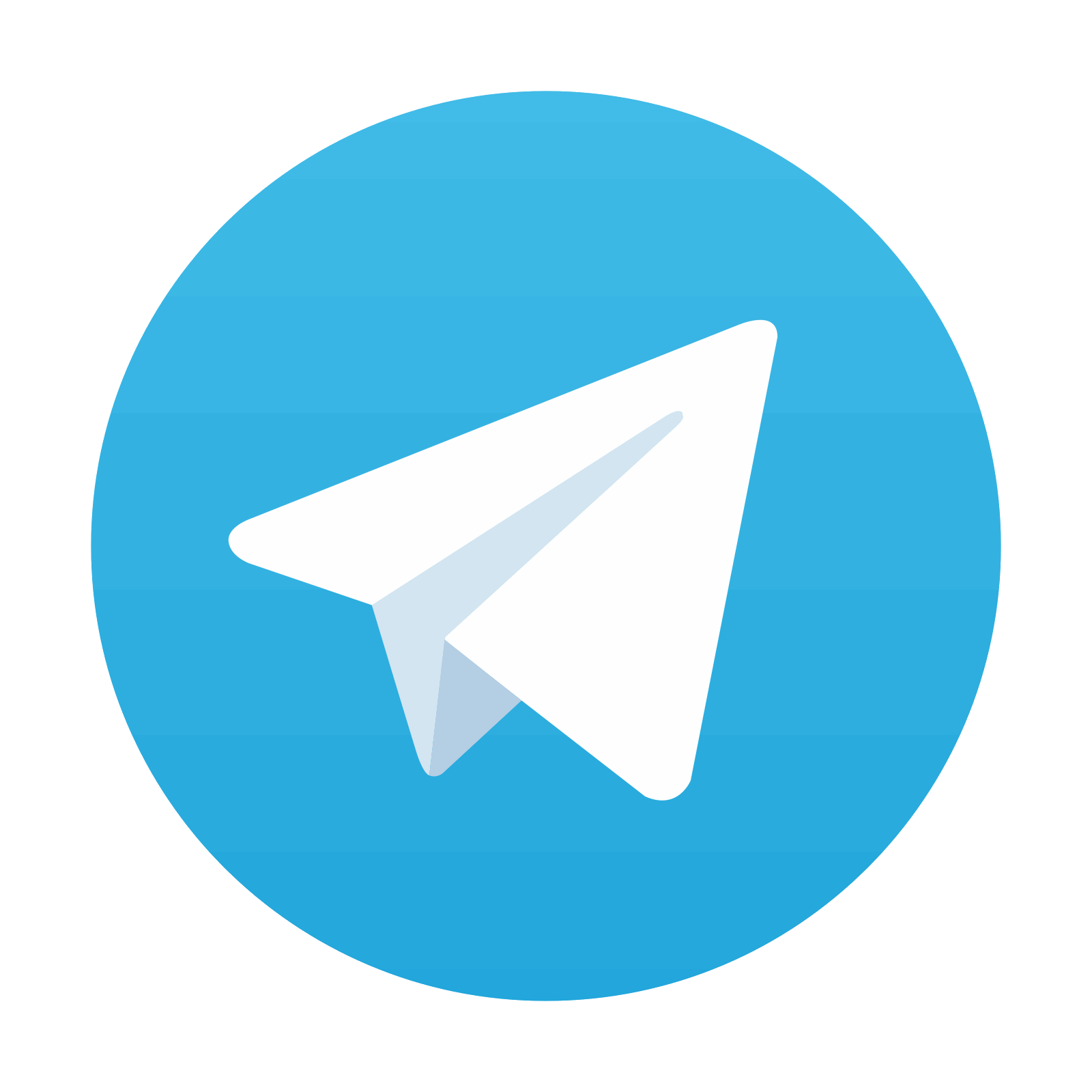
Stay updated, free articles. Join our Telegram channel

Full access? Get Clinical Tree
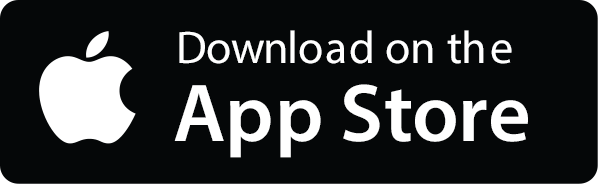
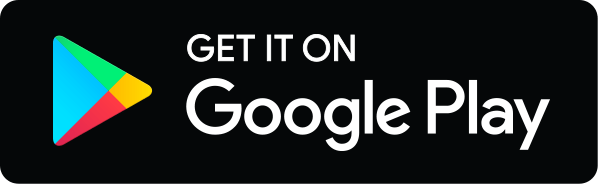