, Taco W. Kuijpers3, 4, Sergio D. Rosenzweig5, Steven M. Holland6, Mario Abinun7, Harry L. Malech8 and Nima Rezaei9, 10
(1)
Department of Pediatrics, Hospital St. Josef, Braunau, Austria
(2)
Network of Immunity in Infection, Malignancy and Autoimmunity (NIIMA), Universal Scientific Education and Research Network (USERN), Tehran, Iran
(3)
Pediatric Hematology, Immunology and Infectious Diseases, Emma Children’s Hospital Academic Medical Center and University of Amsterdam, Amsterdam, The Netherlands
(4)
Department of Blood Cell Research Sanquin Research and Landsteiner Laboratory, University of Amsterdam, Amsterdam, The Netherlands
(5)
Immunology Service, Department of Laboratory Medicine, Clinical Center, National Institutes of Health, Bethesda, MD, USA
(6)
Laboratory of Clinical Infectious Diseases, National Institute of Allergy and Infectious Diseases, National Institutes of Health, Bethesda, MD, USA
(7)
Primary Immunodeficiency Group, Institute of Cellular Medicine (ICM), Newcastle upon Tyne Hospitals NHS FT, Newcastle University, Newcastle upon Tyne, UK
(8)
Laboratory of Host Defenses, Genetic Immunotherapy Section, National Institute of Allergy and Infectious Diseases, National Institutes of Health, Bethesda, MD, USA
(9)
Research Center for Immunodeficiencies, Children’s Medical Center, Tehran University of Medical Sciences, Tehran, Iran
(10)
Department of Immunology and Biology, School of Medicine, Tehran University of Medical Sciences, Tehran, Iran
Keywords
Phagocyte defectsSevere congenital neutropeniasChronic granulomatous diseaseLeukocyte adhesion defect4.1 Introduction
Our understanding of primary immunodeficiency diseases (PID) in general is changing, shifting from the simple towards the more complex, often including more than exclusively the immune system [41]. As with other PIDs, the recent progress in molecular biology over the last decade has facilitated better understanding of the nature of phagocytes defects (Table 4.1). (See Table 1.3 and Fig. 1.10 for updated classification of phagocytes defects)
Diseases | Genetic defects | Inheritance | Associated features |
---|---|---|---|
Chronic granulomatous disease | CYBB | XL | Infections, McLeod syndrome (in patients with deletions extending into the contiguous Kell locus); Discoid lupus and oral ulcers (in female carriers) |
CYBA | AR | Infections, autoinflammatory phenotype | |
NCF1 | AR | Infections, autoinflammatory phenotype | |
NCF2 | AR | Infections, autoinflammatory phenotype | |
NCF4 | AR | Infections, autoinflammatory phenotype | |
Leukocyte adhesion deficiency | ITGB2 | AR | Delayed umbilical cord separation, periodontitis, omphalitis, skin ulcers, leukocytosis |
FUCT1 | AR | Skin ulcers, periodontitis, mental and growth retardation; hh blood group | |
FERMT3 | AR | Skin ulcers, periodontitis, bleeding tendency | |
RAC-2 deficiency | RAC2 | AD | Poor wound healing, leukocytosis |
β-Actin deficiency | ACTB | AD | Mental retardation, short stature |
Localized juvenile periodontitis | FRP1 | AR | Aggressive periodontitis |
Papillon-Lefèvre syndrome | CTSC | AR | Periodontitis, palmoplantar hyperkeratosis |
Specific granule deficiency | CEBPE | AR | Bilobed nuclei of the neutrophils |
Shwachman-Diamond syndrome | SBDS | AR | Exocrine pancreatic insufficiency; chondrodysplasia |
Severe congenital neutropenias | ELANE | AD | Susceptibility to myelodysplasia/leukemia |
GFI1 | AD | B/T lymphopenia | |
HAX1 | AR | Susceptibility to myelodysplasia/leukemia, neurological problems | |
G6PC3 | AR | Structural heart defects, urogenital abnormalities, deafness, venous angiectasias | |
VPS45A | AR | Extrameduallary hematopoiesis, bone marrow fibrosis, nephromegaly | |
WASP | XL | Monocytopenia, myelodysplasia | |
LAMTOR2 | AR | Hypogammaglobulinemia, partial oculocutaneous hypopigmentation, growth failure | |
JAGN1 | AR | Bone phenotype | |
CSF3R | AR | Poor response to GCSF | |
Cyclic neutropenia | ELANE | AD | Oscillations in production of all types of blood cells |
Glycogen storage disease type 1b | G6PT1 | AR | Fasting hypoglycemia, lactic acidosis, hyperlipidemia, hepatomegaly |
3-Methylglutaconic Aciduria | TAZ | XL | Cardiomyopathy, growth retardation |
CLPB | AR | Microcephaly, hypoglycemia, cataracts, neurological problems, hypotonia | |
Cohen syndrome | COH1 | AR | Retinopathy, developmental delay, facial dysmorphisms |
Poikiloderma with neutropenia | C16ORF57 | AR | Poikiloderma, myelodysplasia |
Myeloperoxidase deficiency | MPO | AR | Asymptomatic, candidiasis |
Fifty years after the description by Kostmann, a gene mutation has been identified in patients with the syndrome bearing his name [38, 40]. Long-term follow-up of relatively large patient groups with known gene mutation(s) (thanks to international multi-center studies) [162] will give us more insight into the natural course of these diseases and will influence our treatment approaches in the future. The clinical identification and careful description of individual patients will continue to add to our better understanding of these disease processes. The ESID/PAGID diagnostic criteria for severe congenital neutropenia (SCN) from 2006 are an example: as commonly perceived, SCN is an isolated condition due to several gene mutations, but it is also part of many complex syndromes [75].
Another half-century mark worth mentioning is the ‘coming of age’ of hematopoietic stem cell transplantation (HSCT) [10], still the main curative procedure for most immunodeficiencies. The future looks even better for these patients as the era of gene therapy has arrived, albeit still evolving, with (un)expected complications keeping it from being ‘the perfect treatment’ [207].
4.2 Chronic Granulomatous Disease
(gp91 phox deficiency, p22 phox deficiency, p47 phox deficiency, p67 phox deficiency, p40 phox deficiency)
4.2.1 Definition
Chronic granulomatous disease (CGD) is a genetically heterogeneous disease characterized by recurrent life-threatening infections with bacteria and fungi and dysregulated granuloma formation. CGD is caused by defects in the NADPH oxidase, the enzyme complex responsible for the phagocyte respiratory burst which leads to the generation of superoxide and other reactive oxygen species (ROS). There are five related genetic defects mapping to different chromosomes that result in this phenotype. The disease was first described by Janeway et al. in 1954 [110], but was not well characterized until 1959 by Bridges et al. [33]. It was initially termed fatal granulomatous disease of childhood, but with early diagnosis and better treatment, the prognosis no longer warrants this pessimistic name.
4.2.2 Etiology
The fully assembled NADPH oxidase is a six-protein complex. In the basal state, it exists as two components: a membrane-bound complex embedded in the walls of secondary granules, and proteins in the cytosol [236]. The secondary granule membrane contains the heme and flavin binding cytochrome b 558, composed of a 91-kd glycosylated β chain (gp91 phox ) and a 22-kd nonglycosylated α chain (p22 phox ). The cytosolic components are p47 phox , p67 phox , p40 phox and RAC2.
After cellular activation, such as that initiated by the phagocytosis of microbes, the cytosolic components p47 phox and p67 phox are phosphorylated and bind tightly together. In association with p40 phox and RAC2, these proteins combine with the cytochrome complex (gp91 phox and p22 phox ) to form the intact NADPH oxidase. Following assembly, an electron is taken from NADPH and donated to molecular oxygen, leading to the formation of superoxide (O2 −). In the presence of superoxide dismutase, this is converted to hydrogen peroxide (H2O2), which, in the presence of myeloperoxidase and chlorine in the neutrophil phagosome, is converted to hypohalous acid (OHCl), or bleach [3]. The rapid consumption of oxygen and production of superoxide and its metabolites is referred to as the respiratory burst.
Mutations in five members (gp91 phox , p47 phox , p22 phox , p67 phox , and p40 phox ) of the NADPH oxidase complex account for all known cases of CGD. The majority of the identified mutations in these genes result in complete or nearly complete absence of the NADPH oxidase activity [236]. The gene for gp91 phox , CYBB, (OMIM*300481) maps to Xp21.1 and causes X-linked CGD (OMIM*306400), accounting for about 65–70 % of cases in Western countries or places with low rates of consanguinity. Its partner in the membrane, p22 phox , encoded by CYBA, (OMIM*608508) maps to chromosome 16q24 and causes one of the four forms of autosomal recessive CGD (OMIM*233690), accounting for less than 5 % of cases. The cytosolic factor p47 phox is encoded by NCF1, located at 7q11.23 (OMIM*608512), accounting for about 25 % of cases. The other cytosolic factor, p67 phox , encoded by NCF2, is located at chromosome 1q25 (OMIM*608515), and accounts for less than 5 % of cases [12, 209, 220, 221, 283]. The cytosolic factor p40 phox is encoded by the gene NCF4, located at 22a13.1 (OMIM*601488). To date, defects in NCF4 have been described in a single child suffering from severe inflammatory bowel disease with mildly impaired respiratory burst activity [166].
The nomenclature for various levels of protein expression of gp91 phox has been confusing [236]: when gp91 phox was absent, such as due to a stop codon or a deletion, it has been referred to as X910; when reduced amounts of a hypofunctional protein are present, such as due to a splicing or promoter defect, X91−; and when normal amounts of a nonfunctional protein are present, such as due to a missense mutation, X91+. Similar nomenclature has been used for recessive forms of CGD [222]. However, more recent work has shown that the critical issues surrounding NADPH oxidase characterization are not protein presence or absence, but function. Specifically, mutations in CYBB fall into functional and nonfunctional categories regardless of protein expression, with important clinical consequences [131]. In fact, function can be largely predicted from the mutation: stop codons or deletions obviously are null and have no function and more severe clinical presentations with higher mortality. A bit more surprising is the finding that essentially all missense changes beyond amino acid 310 in CYBB lead to a complete loss of function, while missense changes up to amino acid 309 may have residual function with better survival than those with absent function. That is, those gp91 phox -deficient patients with residual function have clinical courses similar to those with p47 phox deficiency. Similarly, those patients with recessive disease who have complete loss of function have clinical courses similar to gp91 phox -deficient patients with no residual function. This tight genotype-phenotype correlation in CGD indicates that very small increments in residual superoxide production have major effects on survival and disease severity. However, surprisingly enough, these features have no correlation with the frequency or severity of gastrointestinal manifestations in CGD [131].
In general, X-linked CGD tends to have an earlier onset and be more severe than p47 phox deficiency [283]. A single case of a dominant negative mutation in RAC2 presented with an impaired neutrophil respiratory burst due to rac’s critical role in NADPH oxidase function, as well as impaired chemotaxis and adhesion, due to RAC’s critical role in linking surface adhesion molecules to the cytoskeleton [136]. The frequency of CGD in the general population is close to 1:200,000 live births, and likely higher than that. The rates appear about the same across ethnic and racial groups, with about one third of the X-linked mutations representing de novo mutations [16, 102, 270, 283], but in regions with high rates of consanguinity the relative rates of recessive CGD are much higher [285].
The X-linked carrier state for gp91 phox is not entirely silent. Lyonization of the X chromosome leads to two populations of phagocytes in X-CGD carriers: one displays normal respiratory burst function, whereas the other population, which has inactivated the normal X chromosome and left the defective one active, has impaired respiratory burst activity. Therefore, X-CGD carriers have a characteristic mosaic pattern on respiratory burst testing of peripheral blood. As few as 10 % of cells having normal respiratory burst activity is usually sufficient to prevent severe bacterial and fungal infections. However, other manifestations of heterozygous carriage of X-CGD mutations include discoid lupus erythematosus–like lesions, aphthous ulcers, and photosensitivity and are not clearly related to the degree of skewing of X-chromosome inactivation [31, 129]. The ratio of neutrophil Lyonization in peripheral blood is apparently not fixed and may change over time, allowing carrier women and girls to develop a CGD infection diathesis over time.
4.2.3 Clinical Manifestations
Infectious manifestations
CGD can present any time from infancy to late adulthood, but the majority of patients are diagnosed as toddlers and children. However, a growing number of patients are diagnosed in later childhood or adulthood [283].
The frequent sites of infection are lung, skin, lymph nodes, and liver. Osteomyelitis, perianal abscesses, and gingivitis are also common [236, 283] (Table 4.2). Pulmonary infection is typically pneumonia, but hilar lymphadenopathy, empyema, and lung abscesses also occur (Fig. 4.1). The microbiology of infections in CGD is remarkable for its relative specificity. The overwhelming majority of infections in CGD are due to only a limited number of organisms: Staphylococcus aureus, Burkholderia (Pseudomonas) cepacia complex, Klebsiella pnuemoniae, Salmonella species, Serratia marcescens, Nocardia species, and Aspergillus species. In the pre-prophylaxis era, most lung, skin, and bone infections were staphylococcal. With trimethoprim-sulfamethoxazole prophylaxis, the frequency of bacterial infections in general has diminished. Staphylococcal infections in particular are essentially confined to the liver and lymph nodes [283]. Whereas the typical liver abscess in the immunocompetent patient involves enteric organisms, is liquid and easily drainable, the liver abscesses encountered in CGD are dense, caseous, and staphylococcal and have required excisional surgery [147]. More recent experience is that the simultaneous use of steroids and antibiotics allows cure of these liver abscesses [141]. Bacteremia is uncommon, but when it occurs, it is usually due to B. cepacia, S. marcescens, or Chromobacterium violaceum, one of the gram-negative rods that inhabits soil and warm brackish water. Bacterial and Nocardia infections in CGD tend to be symptomatic and associated with elevated C-reactive protein (CRP), erythrocyte sedimentation rate (ESR) and fever [67]. In contrast, fungal infections are much less symptomatic in terms of leukocytosis or fever, and are often detected at asymptomatic stages. Unlike in neutropenic patients, fungal pneumonias do not generally cavitate in CGD, whereas Nocardia infections do.

Table 4.2
Percentage prevalence of frequent infections by site in CGD patients
Type of Infection | USA (n = 368) [283] | Japan (n = 221) [102] | Iran (n = 41) [177] | Germany (n = 39) [145] |
---|---|---|---|---|
Pneumonia | 79 % | 88 % | 65 % | 67 % |
Abscess | 68 % | 77 % | 53 % | 41 % |
Lymphadenitis | 53 % | 85 % | 75 % | 72 % |
Osteomyelitis | 25 % | 22 % | 21 % | 15 % |
Sepsis | 18 % | 28 % | ND | 23 % |

Fig. 4.1
Pneumonia and localized regional BCGitis in a 9 year-old X-linked CGD patient. Chest X-ray showing a right basal pneumonia and 2 calcified lymph nodes on the left axillae sequel to neonatal BCG vaccination
Fungal infections have been the leading cause of mortality in CGD [283]. However, the advent of itraconazole prophylaxis and the newer agents for treatment of filamentous fungal infections, such as voriconazole and posaconazole, have markedly reduced the frequency and mortality of fungal infections in CGD. Bony involvement by fungi typically occurs by direct extension from the lung (Fig. 4.2). Aspergillus nidulans is an organism virtually exclusive to CGD. It causes a much higher rate of osteomyelitis than other fungi, and has had a much higher rate of mortality than Aspergillus fumigatus or other fungi [235, 254].


Fig. 4.2
Osteomyelitis in a 7 year-old X-linked CGD patient. Total body scintigram showing a posterior arch left rib fungal oeteomyelitis in a CGD patient. His only manifestation was increased erythrocyte sedimentation rate in a routine follow up laboratory control. No fever, pain or discomfort was reported at presentation
Besides A. nidulans and C. violaceum, other microorganisms should also encourage physicians to suspect CGD. Granulibacter bethesdensis is a novel gram-negative rod isolated from necrotizing lymph nodes and meninges in CGD [94]. Penicillium piceum is a relatively non-pathogenic fungus that produced lung nodules and osteomyelitis in a CGD patient [227].
BCG vaccine, given to almost 90 % of newborns around the world, is usually the first important infectious challenge CGD patients will face (typically 3.75 × 104 to 3.2 × 106 live organisms/dose). Different BCG strains are used around the world, some of them defined as “Strong” (e.g., Pasteur 1173, Danish 1221) and others as “Weak” (e.g., Glaxo 1077, Tokyo 172) based on their immunogenicity potential, degree of cutaneous hypersensitivity or granuloma formation, and incidence of side effects [17, 250]. BCG complications range from none to self-limited localized BCGitis to fatal disseminated BCGosis (Fig. 4.2). Although all BCG strains appear to be equally aggressive in severe combined immunodeficiency (SCID) patients [158], the type of BCG vaccine may have a role in CGD, where “Strong” strains have a higher and more severe complications (SDR, personal observation). In some CGD surveys, some pneumonias were reported to be mycobacterial, but BCG and tuberculosis infections are clearly increased [34, 49, 137, 283].
Inflammatory manifestations
Patients with CGD are prone to excessive granulation tissue and granulomata (Table 4.3). These can affect any hollow viscus, but are especially problematic in the gastrointestinal (GI) and the genitourinary tracts (GU). Marciano et al. (2004), analyzed 140 CGD patients and found 43 % of the X-linked and 11 % of the AR-CGD patients had symptomatic, biopsy proven inflammatory bowel disease. Abdominal pain was the most frequent symptom [159]. Walther and colleagues found 38 % of patients had some kind of urologic event, including bladder granulomata, ureteral obstruction, and urinary tract infection. All patients with granulomata of the bladder or stricture of the ureter had defects of the membrane component of the NADPH oxidase: 8 had gp91 phox defects and 1 had a p22 phox defect [26]. Steroid therapy is quite effective and surprisingly well tolerated for resolution of obstructive lesions of both the GI and GU tracts. Several reports and many anecdotes confirm the benefit of steroids given at about 1 mg/kg for a brief initial period and then tapered to a low dose on alternate days [45, 181, 208, 253]. Prolonged low-dose maintenance may be necessary and does not appear to be associated with an increased rate of serious infections. There are anecdotal reports of the successful use of infliximab in severe cases of inflammatory bowel disease in CGD patients, but several of these have been accompanied by severe infections and death from typical CGD pathogens [267]. Therefore, we advise against their use in CGD, but if needed, intensified vigilance and prophylaxis for intercurrent infections seem prudent.
Chorioretinal lesions could be seen in up to one fourth of X-linked CGD patients. They are mostly asymptomatic retinal scars associated with pigment clumping. Interestingly, these same lesions can also be detected in gp91 phox female carriers [92]. Bacterial DNA has been isolated from these lesions, which typically do not progress even during profound immunosuppression, making the role of infection in CGD-associated chrorioretinal lesions unclear [278].
Hepatic abnormalities are frequently described in CGD patients. Liver enzymes were reported to be elevated at least once in 73 % of a CGD cohort followed at the NIH (n = 194), 25 % had persistent elevations of alkaline phosphatase and drug-induced hepatitis was reported in at least 15 % of these patients. One-half had splenomegaly that was usually associated with portal vein venopathy; in cases with abnormal liver enzymes who underwent biopsy liver histology, 75 % had granulomata and 90 % had lobular hepatitis [108].
Autoimmune disorders are more common in CGD. Both discoid and systemic lupus erythematosus have been described in CGD patients, and in X-linked female CGD carriers [36, 156] (Fig. 4.3). Idiopathic thrombocytopenic purpura (ITP) and juvenile idiopathic arthritis (JIA) are also more frequent in CGD than in the general population [283].


Fig. 4.3
Cutaneous manifestations in a female CGD carrier. Photosensitive discoid lupus-like lesions involving the cheeks of a 36 years-old X-linked CGD female carrier. A scar on the right side of her neck, secondary to lymphadenitis drainage, can also be seen
The gene coding for the Kell blood cell antigen system (XK) maps to Xp21, immediately adjacent to CYBB, the gene for gp91 phox . Patients with deletions in the X-chromosome may delete portions of both genes (contiguous gene disorder) and thereby present with CGD and McLeod syndrome. McLeod syndrome is a form of acanthocytosis that may result in anemia, elevated creatine phosphokinase, and late-onset peripheral and central nervous system manifestations. Special care has to be taken when transfusing X-linked CGD patients to avoid Kell(+) transfusions into Kell(−) patients [84, 165]. All X-linked CGD patients should be tested for Kell antigens. Consideration should be given to blood storage for CGD patients with McLeod syndrome if bone marrow transplantation is even remotely contemplated.
4.2.4 Diagnosis
A history of recurrent and/or unusually severe infections, particularly abscesses or those caused by the pathogens commonly associated with CGD (see above), should prompt testing for this disorder. Although CGD has no pathognomonic clinical findings, the diagnosis should be particularly considered in the patient with a constellation of characteristic pathologies coupled with characteristic microbiology. Consistent clinical findings include splenomegaly, hepatomegaly, growth retardation, diarrhea, and abnormal wound healing with dehiscence, but these are neither necessary nor sufficient for the diagnosis. CGD patients may have minimal clinical signs and symptoms despite significant infectious involvement. Leukocyte counts are not consistently elevated during infection, whereas elevations of the erythrocyte sedimentation rate (ESR) or C-reactive protein (CRP) are sensitive indicators of infection. Similar to other primary immunodeficiencies, diagnosis and treatment of infections in CGD must be aggressive. Invasive procedures oriented towards direct microbiological diagnosis should be considered as first-line diagnostic tests and should not be left until after the failure of empirical therapy. The reduction in mortality and morbidity in recent years is largely attributable to prophylaxis and aggressive recognition and treatment of infections in these patients [1, 85, 161, 246].
Diagnostic tests for CGD rely on various measures of superoxide production. These include direct measurement of superoxide production, ferricytochrome c reduction, chemiluminescence, nitroblue tetrazolium (NBT) reduction, and dihydrorhodamine 123 (DHR) oxidation. Currently, we prefer the flow cytometry-based DHR oxidation assay because of its objectivity, its relative ease of use, its ability to distinguish between X-linked and autosomal forms of CGD, and the ability to detect gp91 phox carriers [274, 275]. However, myeloperoxidase deficiency gives an abnormal DHR, even when not completely deficient, but can be distinguished by a normal NBT test or other measures of superoxide production directly. It can also be confirmed by myeloperoxidase staining of neutrophils.
The other mentioned techniques are highly effective and provide reliable diagnoses of CGD, but suffer either from an inability to distinguish individual populations or the need for significant operator experience and interpretation.
Several other conditions may affect the neutrophil respiratory burst. Glucose 6-phosphate dehydrogenase (G6PD) deficiency and glutathione synthetase (GS) deficiency may mimic certain aspects of neutrophil dysfunction of CGD, such as the decreased respiratory burst and increased susceptibility to bacterial infections [218, 223, 281]. However, G6PD deficiency is most often associated with some degree of nonsferocytic hemolytic anemia, whereas CGD is not; on the other hand, severe GS deficiency is associated with 5-oxoprolinuria, acidosis and mental retardation, in addition to hemolytic anemia. Diverse pathogens, including Legionella pneumophila, Toxoplasma gondii, Chlamydia, Entamoeba histolytica, and Ehrlichia risticii, have been shown to inhibit the respiratory burst in vitro. Human granulocytic ehrlichiosis infection depresses the respiratory burst by downregulating gp91 phox [14].
Techniques such as immunoblotting can be used to confirm the diagnosis of CGD. Failure to detect p47 phox or p67 phox proteins indicates autosomal recessive mutations in the corresponding genes. A limitation of immunoblotting is that it cannot distinguish between the X-linked gp91 phox defect and the p22 phox autosomal recessive defect, since expression of these two proteins is mutually co-dependent. That is, if there is a deficiency of either one of them, the other is also absent in the membrane [236]. Sequencing of the CGD genes to determine the exact molecular defect is recommended but not necessary. Genetic testing is available through specialized commercial laboratories and selected tertiary referral centers.
Genetic testing may help with risk profiling of X-linked CGD. X-CGD mutations are usually either missense or nonsense. Nonsense mutations generally lead to more severe CGD with diminished survival. Missense mutations that affect amino acids 1–309 are associated with slight DHR positivity, residual superoxide formation and better survival. In contrast, any mutations affecting amino acids 310 and beyond usually alter critical protein functional domains leading to complete loss of DHR activity, more severe CGD, and diminished survival [131].
4.2.5 Management
The cornerstones of CGD management are: (a) Early diagnosis, (b) Antimicrobial prophylaxis with trimethoprim-sulfamethoxazole (TMP-SMX), itraconazole, and interferon-γ (IFNγ), and (c) Aggressive management of infectious complications, which usually include invasive diagnostic procedures and parenteral/prolonged anti-infectious medication. In this section, curative options for CGD are also discussed.
Antimicrobial prophylaxis
CGD is the only primary immunodeficiency in which prospective, randomized, placebo-controlled studies of prophylaxis of infection have been performed [1, 85, 161] Antimicrobial prophylaxis in CGD relies on a triad of antibacterial (TMP-SMX or cotrimoxazole), antifungal (itraconazole) and immunomodulator therapies (IFNγ). Altogether this scheme dramatically reduces the morbidity rates for severe infections from 1 per patient-year to almost 1 every 10 patient-years [1, 85, 160, 161].
The first prophylactic agents shown to be effective in CGD patients were nafcillin and TMP-SMX [124, 204]. With time, TMP-SMX became the standard of care for CGD patients. In a retrospective study, TMP-SMX (5 mg/kg/day) lowered the incidence of bacterial infections from 15.8/100 patient-months to 6.9/100 patient-months in X-linked patients; and from 7.1 to 2.4/100 patient-months in autosomal recessive CGD [161]. No increase in fungal infections has been noted due to the use of TMP-SMX prophylaxis.
Prophylactic TMP-SMX is usually prescribed at 5 mg/kg/day divided twice daily, although several centers use single-day doses to enhance treatment adherence. For patients allergic to sulfonamide drugs, alternatives include trimethoprim as a single agent, oral beta-lactamase stable penicillins such as dicloxacillin, and fluoroquinolones.
Itraconazole is highly effective antifungal prophylaxis in CGD [35, 85, 176, 203]. In the only prospective, randomized, double-blind placebo-controlled antifungal trial in CGD, Gallin and co-workers reported 7 serious fungal infections in patients receiving placebo, compared to only 1 serious fungal infection in those receiving itraconazole (100 mg/day in patients aged 5–12 years; 200 mg/day in patients ≥ 13 years or ≥ 50 kg). The 39 patients in this study were randomized to receive placebo or itraconazole for a year and were then crossed-over to the other arm of the protocol; all patients were on antibacterial prophylaxis and most were receiving prophylactic IFNγ [85]. Itraconazole-resistant fungal infections do occur, but most have been responsive to voriconazole or posaconazole [6, 234]. The advent of the azole antifungal drugs has dramatically altered the clinical consequences of fungal infections in CGD. Azole serum levels are strongly influenced by individual metabolic rates and other medications; therefore, azole blood level monitoring is critical when evaluating fungal treatment response [105]. It is also important to be aware of steroid-azole interaction leading to impaired steroid metabolism in some patients, as this can cause iatrogenic hypercortisolism during therapy and iatrogenic adrenal insufficiency on steroid withdrawal.
Immunomodulatory therapy
An international, multi-center, randomized, double-blind, placebo-controlled trial, showed that IFNγ (50 mcg/m2 subcutaneously three times weekly) reduced the number and severity of infections in CGD patients, regardless of their age, CGD genotype, or concomitant use of other prophylactic agents [1]. This study included 128 CGD patients (4–24 years-old) from 13 centers (10 US, 3 European) and found that IFNγ was well tolerated. Marciano et al. confirmed the tolerability and long-term efficacy of IFNγ in a study of 76 CGD patients followed for up to 9 years [160]. Based on 328 patient-years of observation, the incidence of serious infections was 0.30/ patient-year, and the mortality rate was 1.5 %/patient-year.
For patients over 0.5 m2, IFNγ 50 mcg/m2 three times weekly is recommended, while in children less than 0.5 m2, 1.5 mcg/kg subcutaneously three times weekly is the suggested dose. Fever and myalgias are the most common IFNγ adverse events, but can be minimized by administration before bedtime and concomitant use of acetaminophen.
The need for administration by injection, cost, continuing improvement in prognosis based on better antifungals, and lack of general familiarity with cytokine therapies have contributed to the less than universal use of IFNγ in CGD patients around the world [35, 145, 176]. Despite the strong evidence for IFNγ’s prophylactic benefit in CGD, it has not been shown to help in the treatment of acute infections.
Acute infection management
Life-threatening infections may occur at any time in patients with CGD, even in those who have been free of infections for months or years. Serious infections, particularly those caused by fungi, may be asymptomatic or minimally symptomatic at presentation. Significant increases in ESR or CRP should prompt a search for occult infection. Imaging with plain radiographs, ultrasound, CT, or MR are extremely important for the detection of and determination of extent of infections. Because the differential diagnosis for any specific infection includes bacteria, Nocardia, mycobacteria, and fungi, a definitive microbiologic diagnosis is essential for directing therapy. Biopsies to obtain microbiological specimens should be insisted upon before the initiation of therapy and not after empirical therapy has failed (Fig. 4.4).


Fig. 4.4
Pre, intra and post CT scan-guided FNA in a 7 year-old X-linked CGD patient. (a) A thorax CT scan showing a pleural-based nodular lesion in the basal portion of the left lung (white arrowhead; the patient was placed on prone position for the procedure). (b) Pulmonary fine needle aspiration biopsy performed with a 21G needle (white arrowhead). (c) Post- biopsy control CT scan where no complications are detected (e.g., bleeding, pneumothorax) and a small intralesional scar can be seen (white arrowhead)
While definitive management of infections depends on their etiology, initial empiric therapies are necessary and some general approaches can be outlined. For pneumonias, after diagnostic specimens have been obtained, empirical initiation of TMP-SMX, and/or a carbapenem, and/or fluoroquinolone, along with voriconazole is appropriate. Most Burkholderia, Serratia and Nocardia infections are sensitive to TMP-SMX. The use of TMP-SMX as therapy for infections that have occurred on prophylaxis remains highly effective, and may reflect either the effect of high dose exposure, a failure of patients to actually take their prophylaxis, or both.
Staphylococcal pneumonias are extremely rare after the initiation of prophylaxis, although they may still cause lymph node or liver infections. Lymphadenitis is usually staphylococcal and often necrotic. These infections respond faster to excision along with antimicrobials. Chromobacterium violaceum, a Gram-negative rod that lives in warm brackish water and produces a deep purple pigment, can cause bacteremia and sepsis in CGD. It typically responds to TMP-SMX, quinolones or carbapenems. Granulibacter bethesdensis is a newly identified Gram-negative rod that causes necrotizing lymphadenitis and meningitis in CGD. It grows slowly on Legionella or tuberculosis media and responds best to ceftriaxone [94].
Staphylococcal liver abscess in CGD is a special case, as it responds best to a combined therapy with intravenous antibiotics and steroids (1 mg/kg/d for about 2 weeks followed by slow taper) and allows for avoidance of drainage or liver surgery [141]. Further, liver surgery appears to be associated with more long-term morbidity than steroid treatment of liver abscesses.
In general, fungal infections in CGD are more indolent while bacterial infections are more acute in clinical presentation. However, Siddiqui et al. have described an acute fulminant pneumonitis with hypoxia due to inhalation of mulch or compost [245]. This presentation appears pathognomonic to CGD and requires urgent institution of antifungals and steroids to control the severe pulmonary inflammation.
Granulocyte transfusions have been used in CGD, especially in the setting of refractory fungal infections [109, 194, 273, 288]. However, with the remarkable improvement in antifungals over the last few years, the clinical reasons to use them are very few. Further, granulocyte transfusions often lead to alloimmunization, which may significantly impair the likelihood of successful bone marrow transplantation. Therefore, we view granulocyte transfusions as a useful last resort.
Although bone marrow transplantation is usually contraindicated in the setting of active infection, it has been used repeatedly and successfully for refractory chronic infections in CGD. Ozsahin et al. controlled infections and achieved full immune reconstitution in an 8-year-old boy with Aspergillus nidulans infection [194]. Bielorai et al. reported a similar case [21]. The recent series or Gungor et al. showed rates of success >90 % in CGD patients with active inflammatory or infectious complications [97].
Curative treatments
Successful hematopoietic stem cell transplantation (HSCT) is a definitive cure for CGD [115, 237]. While outcomes may be better in younger patients with less CGD sequelae, HSCT is also useful and successful in patients with recurrent serious infections despite prophylaxis and/or severe, difficult to treat, inflammatory disease [97]. In their European series of 56 patients (mean age 12.7 years, range 0–40), Gungor et al. gave reduced intensity conditioning (high dose fludarabine, low dose or targeted busulfan, and serotherapy with antithymocyte globulin, thymoglobulin, or alemtuzumab) prior to HSCT with unmanipulated bone marrow or peripheral blood stem cells from HLA-matched related donors or HLA-matched unrelated donors [97]. Forty-two patients had intractable infections and/or active inflammation. Overall survival was 93 % at a median follow up of 21 months and the 2-year probability of survival was 96 %, including those patients transplanted in the setting of ongoing infection and/or inflammation. All surviving patients had stable myeloid donor chimerism of at least 90 % and had resolution of all infectious and inflammation. All six cases of acute graft versus host disease (GVHD) ≥ grade II and all four cases of chronic GVHD occurred in patients with HLA-matched unrelated donors. Three patients died from GVHD-related complications. One additional patient, who had an HLA-matched related donor, had secondary graft failure at 9 months and died from hemorrhagic shock 10 days after the second HSCT. Two of the surviving patients have fathered children. These data are very encouraging for the value and safety of HSCT for CGD, even in the setting of active disease.
CGD appears well suited for gene therapy since it results from single-gene defects that almost exclusively affect the hematopoietic system. Retroviral and lentiviral vectors that provide normal gp91 phox , p47 phox , or p67 phox genes can reconstitute NADPH oxidase activity in deficient cells, establishing the proof-of-principle for gene therapy in CGD [63, 153, 279]. Peripheral blood stem cells from five adult patients with p47 phox deficient CGD were transduced ex vivo with a recombinant retrovirus containing a normal p47 phox gene and then reinfused without myeloablative conditioning [153]. Functionally corrected granulocytes were detectable in peripheral blood following this procedure at a peak frequency of 0.004–0.05 % of granulocytes, a level well below that needed for protection.
Subsequently, two adults with X-linked CGD were treated with retrovirus-based gene therapy and non-myeloablative bone marrow conditioning [192]. Clinical response was observed after gene transfer, but both patients had insertional activation of ecotropic viral integration site 1 (EVI1) and developed monosomy 7 [193]. One patient died of infection 27 months after gene therapy. In another study, three adults with X-linked CGD underwent gene therapy and achieved early marking (26 %, 5 %, and 4 %, respectively) [114]. However, over time all had marked diminution or loss of their corrected cells. The long-term risks and effectiveness of gene therapy remain to be determined [114, 193, 252]. New gene therapy trials are underway using lentiviral vectors to reduce the risks of insertional myelodysplasia and more aggressive bone marrow preparative regimens to make room for corrected cells [58, 138, 247].
Prognosis.
When the first 92 patients with “fatal granulomatosis of childhood” were reported, 45 had already died, 34 of them before the age of 7 years. Today, survival is dramatically improved [270]. In the United States CGD registry in 2000, more than 25 % of all living CGD patients (and 42 % of those with autosomal recessive CGD) were 20 years or older [283]. In a German cohort of 39 patients observed over a 22-year period, the survival rate was 50 % through the fourth decade of life [145]. In a British cohort, aggressive antibacterial and antifungal prophylaxis greatly diminished the risk of serious infections compared to historic controls [35].
The quantity and quality of the lives of CGD patients have improved dramatically since its initial description. Life-threatening infections continue to occur, but diagnostic and treatment opportunities have improved as well, making CGD a disease that is eminently survivable. Efforts to focus on the other significant complications of CGD, such as inflammatory bowel disease, are sorely needed. Hematopoietic stem cell transplantation offers definitive correction, and gene therapy should eventually improve and become a therapeutic option. In the interim, antimicrobial prophylaxis with TMP-SMX, itraconazole and IFNγ; early diagnosis of infections and aggressive treatment of them; and aggressive management of CGD-associated colitis will keep patients well.
4.3 Leukocyte Adhesion Deficiency
(ITGB2 or CD18 deficiency, SCL35C1 or CDG-IIc deficiency, FERMT3 or Kindlin3 deficiency)
4.3.1 Definition
During inflammation, white blood cells or leukocytes play a key role in maintaining tissue homeostasis by elimination of pathogens and removal of damaged tissue. Leukocytes migrate to the site of inflammation following a gradient of chemokines, which originates from the source of infection. Upon recruitment to a local vessel, the cells slow down due to transient interactions between selectins and their ligands, which are upregulated on leukocytes and endothelial cells during inflammation. Subsequently, stable adhesion by leukocytic integrins to ligands on the endothelium results in leukocyte arrest, after which the cells extravasate and migrate into the affected tissue.
Leukocyte adhesion deficiencies [i.e., LAD-I (OMIM*116920), -II (OMIM*266265) and -III (OMIM*612840, the latter is also known as LAD-1/v) are caused by defects in the adhesion of leukocytes to the blood vessel wall, resulting in severe immunodeficiency [144]. Patients suffer from recurrent bacterial infections and neutrophilia, but fail to make pus; those with severe disease have delayed separation of the umbilical cord. In LAD-I, mutations are found in ITGB2 (OMIM*600065), the gene that encodes the β subunit of the β2 integrins. In the rare LAD-II disease, the fucosylation of selectin ligands is disturbed, caused by mutations in SLC35C1 (OMIM*605881), the gene that encodes a GDP-fucose transporter of the Golgi system. Fucosylation is important in several cell types, demonstrated by mental retardation and short stature of LAD-II patients. LAD-III is characterized by an additional Glanzmann-like bleeding tendency due to a well-characterized platelet dysfunction. The mutations in LAD-III are found in FERMT3 (OMIM*607901), encoding kindlin-3, a protein involved in the regulation of β integrin conformation in blood cells [269].
LAD-I is an autosomal recessive disorder caused by decreased expression or functioning of CD18, the β subunit of the leukocyte β2 integrins. LAD-I was first described in 1980 and since then several hundred patients have been reported. Mutations are found in ITGB2 (integrin β2, CD18), located at 21q22.3, encoding the β2 integrin. So far, more than 80 different mutations have been reported [269]. Usually, this leads to the absence or decreased expression of the β2 integrins on the leukocyte surface, but sometimes a normal expression of nonfunctional β2 integrins is found. Decreased expression of the common β2 subunit leads to a similar decrease in the expression of all four α subunits on the leukocyte surface (CD11a/CD18 or LFA-1; CD11b/CD18, CR3 or Mac-1; CD11c/CD18 or gp150,95; and CD11d/CD18).
LAD-II was first reported in 1992 in two unrelated boys. So far, fewer than 10 patients have been reported, most of them from the Middle East [79, 269]. Patients with LAD-II have a defect in the fucosylation of various cell surface glycoproteins, some of which function as selectin ligands, such as sialyl Lewis X carbohydrate groups (sLeX, CD15a). As a result, the initial “rolling” of leukocytes over the endothelial vessel wall in areas of inflammation, which is mediated by reversible contact between L-selectins on the leukocytes and E- or P-selectins on the endothelial cells with their respective sialated fucosyl ligands on the opposite cells, is disturbed [205]. Without rolling, the leukocytes cannot slow down and stably adhere, and in this way LAD-II leads to decreased leukocyte extravasation and recruitment at the site of infection. Fucosylation is important as well for several unrelated functions, and LAD-II patients present as a result with additional symptoms, including mental and growth retardation and the Bombay (Hh) blood type [79, 163].
The molecular defect in LAD-II has been identified as a deficiency in a Golgi GDP-fucose transport protein (GFTP) [146, 150]. This protein is encoded by SLC35C1 (Solute carrier family 35 member C1), or FUCT1 (GDP-fucose transporter 1) at 11p11.2. Only seven different mutations have been reported so far [269]. Since the genetic cause reveals that the defect involves glycosylation, LAD-II has now been categorized as one of the group of the congenital disorders of glycosylation (CDG), being reclassified as CDG-IIc [146, 150].
In 1997, for the first time a syndrome affecting a 5-years old boy was reported who was hospitalized with a history of nonpussing inflammatory lesions, leukocytosis and an overt bleeding tendency [135]. Apart from the platelet aggregation defect, similar leukocyte defects are seen in the classical LAD-I syndrome, hence designated the novel combination of leukocyte and platelet defects Leukocyte Adhesion Deficiency type-1/variant (LAD-1/v), which was later termed LAD-III. In LAD-III, all integrins are normally present but fail to be activated during leukocyte or platelet activation [135].
LAD-III has now been identified in more than 25 families worldwide. In addition to recurrent non-purulent infections, LAD-III patients exhibit a severe Glanzmann Thrombasthenia-like bleeding disorder. Families have often lost newborns within weeks after birth, demonstrating the high mortality rate of LAD-III patients [133]. The bleeding disorder originates from a platelet defect, indicating that the signaling defect also affects the β3 integrin fibrinogen receptor αIIbβ3 on blood platelets [135, 268].
The molecular defect in LAD-III is in FERMT3 (fermitin family homolog 3) at 11q13.1 [134, 155, 258], encoding kindlin-3, a protein involved in inside-out signaling to all blood cell-expressed β integrins (β1, β2 and β3). So far, 9 different mutations in FERMT3 have been reported [269].
The kindlin family consists of fibroblast-specific kindlin-1, ubiquitously expressed kindlin-2 and hematopoietic kindlin-3, with high homology between them [154]. Loss of kindlin-1 leads to the Kindler syndrome, a hereditary genodermatosis characterized by skin blistering and cutaneous atrophy. Absence of kindlin-2 is embryonically lethal in mice, corresponding to its ubiquitous expression. Kindlin-3−/− mice were first described in 2008 [175] and characterized by a severe bleeding tendency, anemia and defective leukocyte function. The phenocopy of some of the major LAD-III symptoms in the kindlin-3−/− mice contributed to the discovery of kindlin-3-deficiency as the cause of LAD-III.
A discussion has taken place in the literature about the importance of a genetic variation in the gene encoding CalDAG-GEF1 (a guanine nucleotide exchange factor for Rap1, involved in integrin activation) in some patients with LAD-III [199]. Since the functional defect in such patients can only be corrected by reconstitution with kindlin-3 and not by reconstitution with CalDAG-GEF1, this variation in CalDAG-GEF1 is of no importance for the functional defect in LAD-III patients [258]. Recently, a pedigree was identified with homozygous mutations in the RASGRP2 gene encoding an inactive CalDAG-GEF1. The defect resulted in a moderate platelet defect in aggregation and spreading but no leukocyte defect [37].
The small guanosine triphosphatases (GTPases) Rho proteins are members of the Ras-like superfamily. Similar to Ras, most Rho GTPases cycle between active GTP-bound, and inactive GDP-bound conformations and act as molecular switches that control multiple cellular functions.
4.3.2 Etiology
Circulating leukocytes normally migrate to the site of infection following a gradient of chemoattractants in a process called chemotaxis. These chemotactic factors or chemoattractants may be derived either from the infected tissue or local complement activation, or directly from the pathogens themselves, and diffuse within the tissue into the local vasculature. These gradients of chemoattractants recruit the leukocytes in interplay with factors expressed locally on the luminal side of blood vessel endothelial cells. Neutrophils are short-living leukocytes that are recruited early in the inflammatory response (Fig. 4.5).


Fig. 4.5
The life cycle of the neutrophil is shown, including the phases of migration of neutrophils to sites of infection or inflamed tissues. Neutrophils develop in the bone marrow (upper left) and are released into the circulation. Neutrophils sense infection or inflammation in the post capillary venule (bottom of figure) where bacterial factors and inflammatory chemoattractants and chemokines act on both the neutrophils and endothelial cells to increase adhesion. The initial phase of increased adhesion engages selectins which mediate short-lived weak binding encounters between neutrophils and endothelium (rolling). This is followed by activation of integrins, triggering strong adhesive forces that mediate spreading of neutrophils onto the endothelium. This is followed by additional conformational changes that weaken integin adhesion, allowing chemotactic migration of the neutrophil between endothelial cells (lower right), though the basement membrane, and into the tissues to the site of infection. At the site of infection neutrophils phagocytose bacteria (upper right) or other pathogens, triggering the process of degranulation, production of reactive oxygen species, and activation of proteases. Over hours to days, neutrophils proceed into an apoptotic phase (upper middle), triggering engulfment by macrophages in a process that minimizes tissue damage and leads to resolution of inflammation
Leukocytes following the chemotactic gradient towards the site of infection have to leave the blood stream, in a process called extravasation. Extravasation is a multi-step process involving adhesion molecules, in which chemoattractants function as activating agents or (pro-) inflammatory mediators. The first step of extravasation consists of initial contact between endothelial cells and leukocytes marginated by the fluid flow of the blood. L-selectin (CD62L) on leukocytes plays a role herein, contacting several cell adhesion molecules on endothelial cells. Within the local environment of an inflammatory tissue reaction, the endothelium begins to express the adhesion molecules P-selectin (CD62P) and later on E-selectin (CD62E). The low-avidity interaction of these selectins with their fucosylated ligands on the opposite cells forces the leukocytes to slow down and start a rolling movement along the vessel wall [290].
In contrast to the low-avidity binding of leukocytes to selectins, the final step of firm adhesion and subsequent migration depends on stable interaction between integrins on the leukocytes and their ligands on the endothelial cells upon leukocyte activation by endothelial factors [132, 242, 255].
Integrins are ubiquitously expressed transmembrane receptors consisting of an α and a β chain. They represent the major class of adhesion receptors on hematopoietic cells. In mammals, 18 α and 8 β subunits form 24 known combinations, each of which can bind to a specific repertoire of cell-surface, extracellular matrix, or soluble ligands. Different hematopoietic cell types and tissues express different integrins. On leukocytes, α4β1 (VLA-4), α5β1 (VLA-5), αLβ2 (LFA-1; CD11a/CD18), αMβ2 (CR3; CD11b/CD18), αXβ2 (gp150,95; CD11c/CD18) and αDβ2 (CD11d/CD18), the latter only being expressed on macrophages, are the most prominent family members, whereas αIIbβ3 and α2β1 are the predominant integrins expressed on platelets [2, 151].
Integrins are type I transmembrane glycoproteins that form heterodimers via non-covalent association of their α and β subunits, with sizes of 120–170 kDa and 90–130 kDa, respectively [151]. The β2 integrin receptor subfamily is selectively expressed on leukocytes and bind to adhesion molecules on endothelial cells (intercellular adhesion molecule [ICAM]-1 and ICAM-2) and tissue cells (ICAM-1), as well as to several extracellular proteins and plasma opsonins, such as complement factors. The main β2 integrin on neutrophils is CR3.
Once leukocytes are slowly rolling along the endothelial cells, these leukocytes are able to recognize concentration differences in a gradient of chemoattractants and to direct their movement towards the source of these agents. Although the details of this process remain unknown, the gradient most likely causes a difference in the number of ligand-bound chemoattractant receptors on either side of the cell, thereby inducing the cytoskeletal rearrangements needed for movement [242]. Since adhesion molecules such as the β2 integrins are essential for the connections with the tissue cells or with the extracellular matrix proteins, these connections must be formed at the front of the moving leukocytes and broken at the rear end [83]. For continued sensing of the chemoattractant gradient, the chemoattractants must dissociate from their respective receptors for repeated usage. This occurs through internalization of the ligand-receptor complex, intracellular disruption of the connection, and transport of the free receptor to the front of the cell, followed by reappearance of the free receptor on the leukocyte surface. Within the infected tissue, the chemoattractant gradient persists and leukocyte migration is maintained.
The ligand specificity of integrins is determined by their large extracellular ligand-binding head domain, which is composed of several domains of both the α and β subunit. The head domain is attached to the membrane via two flexible legs (one from each subunit), which terminate intracellularly as short cytoplasmic tails. This domain architecture of integrins underlies their ability to transduce bidirectional signals across the plasma membrane: “inside-out” and “outside-in” [242]. Leukocyte activation, e.g. as a result of chemokine binding to chemokine receptors, ligand binding to selectins, or antigen binding to the T-cell receptor, and subsequent intracellular signaling induces conformational changes in the extracellular regions of the β2 integrins, leading to an enhanced affinity for their ligands (“inside-out” signaling). In addition, integrins cluster in larger complexes, which increases their ligand avidity. Binding to extracellular ligands leads to further conformational changes of the β2 integrins, resulting in high ligand affinity and subsequent recruitment of cytosolic proteins and the initiation of downstream signaling cascades that regulate cell spreading and alter gene expression, cell proliferation, differentiation and apoptosis (“outside-in” signaling) [104, 242].
The common activator of most, if not all, integrins is talin, a large cytoskeletal protein that acts as an allosteric activator of integrins by inducing their ligand-binding affinity [242]. The head domain of talin contains a so-called FERM (4.1 protein, ezrin, radixin, moesin) domain, consisting of three subdomains, F1, F2 and F3. The latter, the F3 subdomain, contains a phosphotyrosine-binding (PTB)-like domain that binds to the NPxY/F motif found in the membrane-proximal cytoplasmic region of several β integrins. The head domain is connected to a long cytoplasmic rod which can interact with the cytoskeleton.
The kindlin proteins have been identified as additional relevant players in the activation of integrins on blood cells. Kindlins comprise a family of integrin-binding proteins [154]. In man, the family consists of three members – kindlin-1, 2 and 3 – that share a high degree of homology. Kindlin-3 is only expressed in hematopoietic cell types, where it plays an important role in a variety of functions depending on integrin-mediated adhesion, such as platelet clot formation and leukocyte extravasation. Biochemical studies have confirmed that all kindlins directly bind synthetically generated cytoplasmic tails of β1, β2 and β3 integrins [100]. Although kindlins possess a FERM domain that is homologous to that of talin, recent studies have demonstrated that the kindlin-binding site of β integrins is distinct from the talin-binding site, i.e. at a membrane-distal NxxY/F motif in the cytoplasmic integrin tail. Biochemical studies with mutants of kindlin-2 have shown that the PTB domain in F3 is, in analogy to talin, essential for integrin binding, in addition to a requirement of the N-terminus of the protein for interaction with β3 [78, 87, 100] (Fig. 4.6).


Fig. 4.6
Leukocyte integrin activation. Upon cell stimulation via e.g. G-protein-coupled receptors (GPCR) for chemoattactants, inside-out signaling results in recruitment of talin-1 and kindlin-3, which act in concert to induce conformational changes in integrins from a low ligand-binding affinity towards an intermediate and subsequent high-affinity state. Talin-1 binds to a membrane-proximal NPxY/F motif whereas kindlin-3 binds to a membrane-distal NxxY/F motif of the β integrin cytoplasmic tails
In sum, leukocyte adhesion deficiencies (i.e., LAD-I, −II and -III, the latter also known as LAD-1/variant) are immunodeficiencies caused by defects in the adhesion of leukocytes (especially neutrophils) to the blood vessel wall. As a result, patients with any LAD subtype suffer from severe bacterial infections and neutrophilia, often preceded by delayed separation of the umbilical cord. LAD-II is characterized by additional developmental problems, whereas in LAD-III, the immune defects are supplemented with a Glanzmann-like bleeding tendency.
The talin and kindlin-3 mediated outside-in affinity regulation of the integrins is essential for the leukocyte and platelet adhesion to their respective substrates. Whereas kindlin-3 defects have been demonstrated to cause LAD-III (or LAD-1variant), any inherited defect in talin-1 has not yet been reported – if compatible with life at all. The regulation of adhesion depends on a signaling cascade that may result in similar adhesion defects.
4.3.3 Clinical Manifestations
LAD-I manifests as recurrent, life-threatening bacterial infections, primarily localized to skin and mucosal surfaces. Infections are usually apparent from birth onward, together with severe septicemia in some patients, and a common presenting feature is omphalitis with delayed separation of the umbilical cord in severe cases (Fig. 4.7). Later on patients develop non-purulent, necrotizing infections of the skin and mucus membranes, resulting in a high mortality rate at early age. Absence of pus formation at the sites of infection is a hallmark and the infections have a high tendency for recurrence; secondary bacteremias may also occur. Among patients who survive infancy, severe gingivitis and chronic peridontitis are major features. Fungal infections may present in individual cases [83].


Fig. 4.7
Severe omphalitis in a child with LAD-1
The clinical course of LAD-II with respect to infectious complications is milder than LAD-I, and correlates with lower leukocyte counts. While rolling is defective in LAD-II patients, the adhesion and transmigration via β2 integrin is intact, thereby apparently permitting some neutrophil mobilization to sites of inflammation, and allowing some level of neutrophil defense in tissues. In addition, the mechanisms of β2-integrin activation are still intact [205]. Although recurrent bacterial infections occur in almost all patients, they often are not very severe and do not result in overt wound healing defects or necrotic lesions as in LAD-I. Most infections occur in the first years of life, although periodontitis has been reported at later ages [79, 163].
However, LAD-II patients present with other abnormal features, such as short stature, mental retardation and facial dysmorphisms. Patients are born at term, with no apparent dysmorphism, but severely impaired postnatal weight gain and microcephaly were reported in most patients. In some families intrauterine growth retardation was sufficient to screen for LAD-II prenatally. In addition, convulsions, cerebral atrophy and autistic features were reported for more than half of the patients [89].
It should be mentioned that the early and late features of LAD-II, namely, moderate immunodeficiency accompanied by neutrophilia in the first few years of life and severe mental retardation and short stature in childhood, are also prominent features of other congenital disorders of glycosylation (CDGs).
LAD-III patients suffer from severe recurrent non-purulent infections [135]. In addition, LAD-III patients are affected by a bleeding tendency, similar or more severe than exhibited by Glanzmann thrombasthenia patients [133, 135].
Some patients suffering from LAD-III may also present with an osteopetrosis-like bone defect in addition to the increased bleeding tendency and recurrent infections. A prominent osteopetrosis phenotype was also observed in the kindlin-3 knockout mice. The cause of this osteopetrosis might lie within the osteoclasts, which represent macrophage-like hematopoietic cells critical for bone resorption. Bone resorption requires the formation of a so called ‘sealing zone’ that depends on αvβ3 integrin-mediated adhesion to the bone, thereby explaining the skeletal defect [175, 233]. However, the prevalence and manifestations of osteopetrosis in the patients differ, as unaffected bone formation is also found in LAD-III. The reason for this heterogeneity remains unclear.
4.3.4 Diagnosis
LAD-I patients exhibit mild to moderate leukocytosis, especially granulocytosis, with neutrophil counts reaching levels above 100,000/μL during acute infection [83]. Due to the lack of adhesive capacity only few, if any, leukocytes are present at the sites of infection, which are most often caused by Staphylococcus aureus, streptococci or Gram-negative enteric organisms.
Definitive diagnosis of LAD-I is based on genetic analysis, revealing mutations in ITGB2. Flow cytometry with antibodies to detect CD18 allows discrimination of two forms of LAD-I, i.e. a severe form with less than 2 % CD18 expression and a moderate form with 2–30 %. However, using CD18 alone for diagnosis is problematic: protein positive mutations that are hypofunctional can be misleading. Therefore, assessment of both CD18 and CD11a is suggested, which increases sensitivity of diagnosis of LAD-I [143]. The severity of clinical presentation and complications in LAD-I correlates with the percentage of leukocytes demonstrating normal CR3 cell surface expression and/or the degree of CD18 molecule deficiency. Patients with severe LAD-I exhibit earlier, more frequent, and more serious episodes of infection, often leading to death in infancy, whereas patients with a moderate to mild phenotype manifest with fewer serious infectious episodes and survive into adulthood.
Extensive in vitro studies on neutrophil functions have demonstrated a marked defect in random migration as well as chemotaxis to various chemoattractants. Adhesion to and transmigration across endothelial cell layers were found to be severely impaired. Neutrophils fail to mobilize to skin sites in the in vivo Rebuck skin-window test [83].
The biochemical hallmark of LAD-II is a lack of expression of fucosylated glycoconjugates, such as the Lewis antigens Lewis X (LeX) and sialyl Lewis X (sLeX) on leukocyte proteins, α1,6-core fucosylated N-glycans on fibroblast proteins and blood group antigen H on erythrocytes, the latter known as the rare Bombay blood group phenotype. Expression of L-selectin (CD62L) and CR3 (CD11b/CD18) on LAD-II neutrophils is normal [79, 163].
Neutrophil values range from 5000 to >50,000/μL in absence of infection up to 150,000/μL during infectious episodes. With intravital microscopy, it was observed that LAD-II neutrophils roll poorly, i.e. 15 % of the rolling fraction of control and LAD-I neutrophils [205]. The neutrophil counts remain high during childhood and then drop at adolescence; this finding might be explained by an improvement in adaptive immunity with age, providing better defense against infections and reducing the stimuli for neutrophilia.
Final proof of LAD-II arises from genetic analysis of the SLC35C1 gene. The mutation seems to determine the severity of LAD-II: whereas GFTP is improperly located in the ER in some patients, it is directed to the Golgi but still dysfunctional in others, the latter correlated with a milder immunological phenotype.
LAD-III should also be confirmed by genetic analysis, which should identify mutations in FERMT3. Expression of integrins on neutrophils and platelets (i.e. αIIbβ3, α2β1) is normal or slightly increased, and integrin activation can be induced by artificial stimulation with mAbs or cations. Based on the persistent leukocytosis, many of the patients were suspected to suffer from juvenile myelomonocytic leukemia (JMML) [133]. However, the increased sensitivity of bone marrow (BM) or blood cells to GM-CSF as the hallmark for JMML, is negative in LAD-III.
Many tests have been performed on LAD-III neutrophils. One example of an assay to discriminate between LAD-I and LAD-III neutrophils is the NADPH oxidase screening test with unopsonized zymosan [133]. Zymosan is used to induce uptake and NADPH oxidase activity in purified neutrophils based on the requirement for kindlin-3-dependent CR3 activation before uptake of the zymosan. The response is absent in both types of LAD, but activation and subsequent zymosan uptake can be induced by high Mg2+ concentrations only in case of LAD-III. Similarly, neutrophil adhesion to CR3 ligands is absent in response to several chemoattractants, but can be induced with Mn2+ upon artificial integrin activation.
In addition to the recurrent infections, LAD-III patients suffer from a bleeding tendency. Platelets from Glanzmann patients are still capable of forming small aggregates upon collagen stimulation, whereas platelets from LAD-III patients are not [268]. These aggregates require functional GPIa/IIa (integrin α2β1), thus explaining the clinically more severe bleeding manifestations in LAD-III patients, in which all platelet integrins are functionally defective.
Rac2 −/− mice have a phenotype similar to the human diseases of LAD and chronic granulomatous disease (CGD), including increased susceptibility to Aspergillus infection [219]. The mice show a prominent leukocytosis likely due to reduced shear-dependent endothelial capture via L-selectin (CD62L) and defective neutrophil chemotaxis in response to multiple agonists.
Neutrophils have reduced F-actin assembly, reduced phagocytosis and reduced superoxide production by the NADPH oxidase complex in response to the chemoattractant fMLP.
4.3.5 Management
The only curative treatment for LAD-I and LAD-III is HSCT. In case of LAD-II, oral fucose supplementation may moderate the immune defect, but the mental condition is hardly if at all improved by this treatment.
Antibiotics are commonly used to prevent and treat acute or recurrent infections, and patients affected with the moderate form may survive to adulthood with antibiotics only. As a curative treatment, HSCT is the only approach, and is most often the treatment of choice for patients suffering from the severe form of LAD-I.
Both reduced-intensity and myeloablative conditioning regimens are currently being used in HSCT of LAD-I patients. With myeloablative conditioning, more complete depletion of host marrow can be achieved, thereby decreasing the possibility of mixed chimerism and the risk of rejection. However, pre-transplant infections in immunodeficient patients lead to a high rise in mortality rate with this regimen, especially in patients suffering from co-morbid complications. According to studies by the group of Hamidieh et al., use of the less toxic reduced-intensity conditioning (RIC) regimen is found to be a more safe and feasible therapeutic approach in the treatment of LAD-I patients [99]. Recipients of RIC transplant, those with either full or mixed chimerism, had a long-term survival rate with no manifestation of LAD-I symptoms.
Further, granulocyte transfusions have been reported as a successful supplementation to LAD-I treatment. A patient who was suffering for more than a year from an ecthyma gangrenosum lesion, despite treatment with targeted antibiotics and anti-fungal therapy, has been cured by massive granulocyte transfusions [170]. Overall, the role of granulocyte transfusion in acute infectious episodes is debatable owing to its side effects.
In contrast to the severe form of LAD-I, the moderate form of LAD-I can often be controlled with prompt use of antibiotics during acute infectious episodes and, sometimes, prophylactic antibiotics, but frequent use of antibiotics may result in resistance of the bacteria. HSCT on the other hand can be unsuccessful especially in case of an incompletely matched donor. Survival of HSCT treatment is lower than average for immunocompromised patients, presumably owing to the risk of pre-transplant infections.
Infections are commonly treated with antibiotics. In addition, high-dose oral supplementation of fucose had strong beneficial effects in some, but not all patients [103, 149, 164]. During 9 months of treatment with fucose of the first patients, infections and fever disappeared, elevated neutrophil counts returned to normal, and in one of the patients even psychomotor capabilities improved. However, treatment of the original two Israeli Arab patients did not exhibit a similar beneficial response. In one of the patients treatment led to an autoimmune neutropenia upon refucosylation of the surface antigens [103]. Upon discontinuation of the therapy, selectin ligands were lost and neutrophil counts increased again within a week [149].
The metabolic pathways causing the severe psychomotor and growth retardation are still unclear. Oral fucose supplementation may cure immunological symptoms in some cases, but developmental delay hardly improves.
Patients with LAD-III need prophylactic antibiotics as well as repeated blood transfusions, but the only curative therapy is HSCT. While untransplanted, the need for transfusion differs per patient and can rise to more than 20 and 50 transfusions per year for erythrocytes and platelets, respectively [133]. In addition, granulocyte transfusions have been used and are believed to have improved pathogen clearance.
The survival of untransplanted LAD-III patients is low, and the high mortality is further demonstrated by the incidence of deceased siblings who were not diagnosed but suffered from similar symptoms. Less than four patients have so far survived childhood without HSCT, and the oldest reported patient is a young adult now, though the need for platelet transfusions has increased to 1–2 transfusions per week (unpublished data). Upon successful HSCT, patients may continue to live without further symptoms [73].
Whereas the success rate of HSCT has improved over the last years, pre-transplant infections and the bleeding disorder pose major complications in the treatment of LAD-III patients.
4.4 RAC-2 Deficiency
4.4.1 Definition
RAC-2 deficiency or neutrophil immunodeficiency syndrome (OMIM*608203) is also a leukocyte migration disease. As in patients with LADs (Sect. 4.3) and β-actin deficiency (Sect. 4.5), there is lack of pus formation at the site of infection [7]. Ambruso et al. reported an infant with recurrent infections and poor wound healing, suggesting a neutrophil defect, in whom they found a missense mutation in the RAC2 gene [7].
While most Rho GTPases are expressed widely, the expression of Rac2 is restricted to hematopoietic cells. Of the various Rac isoforms, Rac2 predominates in the human neutrophil. Studies using mutant mice have identified several Rac2 GEFs, including DOCK2, GIT2, and P-Rex1, required for neutrophil function. Whereas DOCK2 and GIT2 regulate both Rac1 and Rac2 activities, genetic data suggest that P-Rex1 functions as a predominant Rac2 GEF in mouse neutrophils [66]. P-Rex1-deficient neutrophils demonstrate a selective defect in Rac2 activation following fMLP stimulation, and P-Rex1−/− neutrophils phenocopy many of the functional defects observed in Rac2−/− cells [66, 219].
Interestingly, the phenotype was predicted by a mouse knock-out of Rac2 and resembles leukocyte adhesion deficiency (LAD) in many aspects [195].
4.4.2 Etiology
Ras-related C3 botulinum toxin substrate 2 or RAC2 (OMIM*602049) is a Rho-GTPase important for the expression of L-selectin, F-actin assembly, chemotaxis and superoxide generation and regulation of actin polymerisation. In activated neutrophils the cytosolic RAC2 comigrates with p67 phox (RAC-1 in macrophages) to attach to p47 phox to form the NADPH oxidase complex (Fig. 4.8) [13]. Besides p47 phox inducible Nitric oxid (iNos) has been suggested to play a role in neutrophils of iNOS-knockout mice [113]. The mutant RAC2 does not bind to its physiological ligand GTP, thus activation of superoxide production via phagocyte oxidase is inhibited [188]. Neutrophils from mice deficient in RAC-2 have defects in rolling on endothelium, chemotaxis and phagocytosis [219]. In humans neutrophils show also defects in chemotaxis, decreased release of enzymes of azurophilic granules after activation with fMLP or PMA and a deficient polarization and actin polymerisation in response to fMLP as well as a deficient production of reactive oxygen radicals (ROS) to fMLP. Interestingly, the syndrome combines feature seen in LAD, chronic granulomatous disease (CGD), specific granule deficiency (SGD) and β-actin deficiency. The RAC2 gene is located on chromosome 22q13 and has a size of 18 kb. In a zebra fish model Rac2 signaling is necessary for both neutrophil 3D motility and CXCR4-mediated neutrophil retention in hematopoietic tissue [59]. In a recent study in Rac2−/− mice an impaired response to Citrobacter rhodentium infection with clinical signs of severe colitis suggests that impaired Rac2 function may promote the development of inflammatory bowel disease [81], which may be linked in humans to rare p67 phox variants with a reduced binding to RAC2 [178].


Fig. 4.8
Molecular features of activation mediated assembly of the phagocyte NADPH oxidase from subunit components are shown. The cartoon images of the subunits are highly schematized and not drawn to scale in order to emphasize some of the known structural features each subunit and some of the intra- and intermolecular binding affinities in the resting state (left side of figure) and upon assembly of the fully activated oxidase (right side of figure). Some known or suspected binding interactions between specific domain motifs are indicated by dotted lines. In the resting neutrophil the cytochrome b558 heterodimers consisting of gp91phox and p22phox are predominantly present in small vesicles and specific granules (upper left). “N”, “F”, and “H” labels on the gp91phox subunit indicate, respectively, the NADPH binding pocket and the Flavin binding site in the cytoplasmic C-terminal region, plus the two Heme moieties within the transmembrane region. Three n-glycosylation sites on two of the intravesicular (topologically extracellular) domains of gp91phox are indicated by the small tree-like stick figures. Indicated in the C-terminal tail of the p22phox subunit is a basic proline-arginine rich region (PR motif) capable of binding with a SH3 domain. In the resting neutrophil the p47phox, p67phox and p40phox subunits exist in the cytoplasm predominantly as a heterotrimer, and the rac2 (rac1 in monocytes) exists separately in its unactivated inhibited GDP charged state bound to Rho-Guanine Nucleotide Dissociation Inhibitor (Rho-GDI). Both p47phox and p40phox have PX domains at the N-terminal portion of the molecule that are protected by intramolecular interactions in the resting state, but which engage specific species of membrane lipid inositides in the activated cell. Of importance in the resting state is that a very basic autoinhibitory region (AIR) of p47phox interacts with one of its own SH3 regions while a PR motif in a nearby domain binds to the C-terminal SH3 domain of p67phox. Both p67phox and p40phox contain PB1 motifs that mediate binding between these two subunits, an interaction that also appears to stabilize and protect p67phox from proteolysis. There is also some evidence to suggest that in the resting state, there is an additional intramolecular interaction between the PX domain and PB1 domain of p40phox that inhibits and protects that PX group. Upon activation of the neutrophil, vesicles and specific granules containing membrane cytochrome b558 fuse with the forming phagosome (upper right), with early endosomes and/or at the plasma membrane. Phosphorylation of the AIR region of p47phox disengages and unfolds it from the SH3 domain, leaving that SH3 domain free to interact with the PR motif of p22phox. Other phosphorylation events induce additional conformational changes in p47phox and p40phox that enhance binding of PX domains to newly generated forms of membrane inositides. There is some evidence to suggest a distinct binding predilection of p47phox or p40phox PX domains, for the types of inositides appearing on activation in phagosome membranes and early endosome membranes, respectively (indicated schematically). Neutrophil activation also triggers disengagement of rac2 from the Rho-GDI with exchange of GDP for GTP allowing binding of rac2 to the TPR region of p67phox and interaction of the rac2 myristoylated C-terminus with the membrane. The fully assembled oxidase shown schematically on the right side of the figure allows electrons to flow from NADPH through the flavin and heme moities to molecular oxygen to form superoxide in the phagosome
The G-protein-coupled receptors (GPCR) for chemoattractants that allow increases in integrin avidity and actin-polymerization are disturbed upon cellular activation in the setting of RAC2 deficiency [7].
4.4.3 Clinical Manifestations
Mutations in the hematopoietic-specific GTPase, RAC2, have been found to cause a severe phagocytic immunodeficiency in humans, characterized by life-threatening infections and poor wound healing starting at infancy [7, 195].
Patients with RAC2 deficiency suffer from delayed separation of the umbilical cord, poor pus formation, non-healing perirectal/periumbilical abscesses, and peripheral blood leukocytosis similar to LAD-1. Reduced binding of RAC2 to a genetic variant of p67 phox may be associated with inflammatory bowel disease.
Both children were found to have a heterozygous dominant negative c.169G > A, p.Asp57Asn (D57N) mutation. This mutation corresponds to mutations in the GTP binding pocket of other Rho GTPases and Ras superfamily members, such as p21Ras D57A, that result in dominant negative activity. The second case was identified by newborn screening for SCID by current TREC analysis.
Why the TRECs are disproportionately low in this case of a relatively mild lymphopenia remains unclear. Overall the lymphocyte phenotype of the human mutation is less severe than that seen in the Rac2-deficient mouse, which may reflect the differences between a murine null and the dominant-negative human mutants.
There is also a recent interesting report of common variable immunodeficiency in two siblings with homozygous complete RAC2 deficiency in consanguineous Iranian siblings [5].
4.4.4 Diagnosis
Many tests have been performed on RAC2-deficient neutrophils. One example of an assay to discriminate between LAD-I, LAD-III and RAC2-deficient neutrophils is the NADPH oxidase screening test with zymosan and the F-actin polymerization test [7, 135]. Adhesion may be affected to a certain degree, but spreading and chemotaxis are defective in RAC2-deficiency.
Wound biopsies show appropriate number of neutrophils and normal CD18 expression, differentiating this disease from LAD-1. Chemotaxis toward C5a, fMLP, and IL-8 is impaired. Moreover, neutrophil polarization in response to fMLP is also deficient. NADPH oxidase activity is normal after PMA, but decreased after fMLP stimulation [190], which in itself already demonstrates the uniqueness of RAC2-deficient neutrophil reactivity.
4.4.5 Management
Patients with mutations in RAC2 need prophylactic antibiotics as well as repeated blood transfusions, followed by the only curative therapy of HSCT. Survival of untransplanted RAC2 deficient patients is unknown. Only 2 patients with dominant negative RAC2 mutations have so far survived childhood with HSCT (the oldest reported patient has become a teenager, unpublished data). Of the 2 patients with complete deficiency of the protein due to autosomal recessive defects in RAC2, one died at 21 after a renal transplant rejection, while the other was alive at 28 years of age without HSCT [5]. No neutrophil defect was observed in the complete RAC2 deficiency, in contrast to the two de novo autosomal dominant-negative D57N mutated cases reported to date. Upon successful HSCT, dominant-negative RAC2-defective patients may continue to live without further symptoms. It remains to be seen whether the CVID-like complete RAC2-deeficient individuals have a similar life-threatening risk to the more severely affected patients with a heterozygous de-novo dominant-negative mutation.
4.5 β-Actin Deficiency
4.5.1 Definition
β-actin deficiency (OMIM*243310) is a leukocyte migration disease. As in patients with LAD syndromes, there is no pus formation at the site of infection.
4.5.2 Etiology
β-actin deficiency is an autosomal dominant deficiency of the actin polymerisation of neutrophils. A heterozygous negative dominant mutation of non-muscle β-actin (ACTB) (OMIM*102630) impairs the binding of profilin, which is an actin regulatory protein [189].
4.5.3 Clinical Manifestations
The patients suffer from recurrent bacterial and fungal infections without pus formation, mental retardation and photosensitivity. One patient developed recurrent stomatitis, cardiomegaly, hepatomegaly and hypothyroidism [188].
4.5.4 Diagnosis
Wound biopsies show reduced numbers of neutrophils. Chemotaxis and phagocytosis is markedly impaired as well as polymerisation of actin monomeres after activation. LAD-1 (CD18) and LAD-2 (CD15s) should be excluded. Definitive diagnosis can be achieved by mutational analysis of the ACTB (cytoplasmic actin) gene.
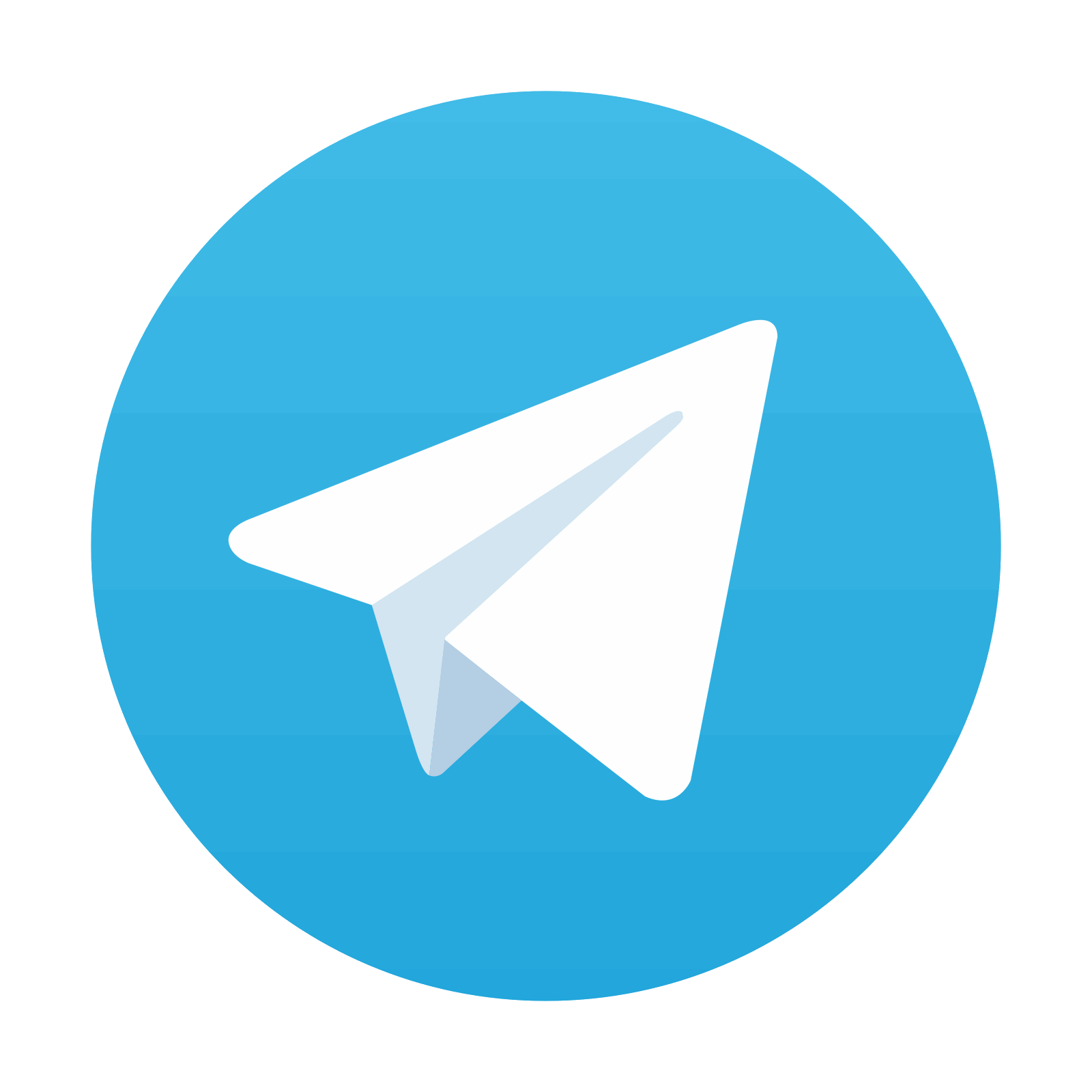
Stay updated, free articles. Join our Telegram channel

Full access? Get Clinical Tree
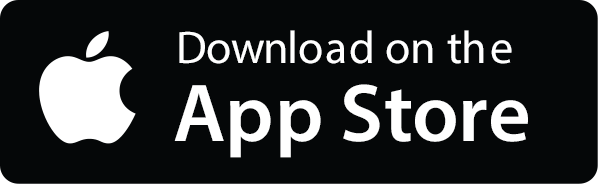
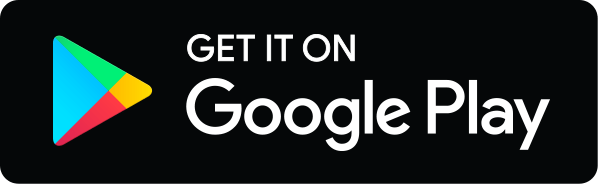