Fig. 10.1
Molecular structure of D2 and D3
Table 10.1
Food sources of vitamin D and calcium
Dietary sources of calcium and vitamin D |
Calcium content |
Vitamin D content |
---|---|---|
Breast milk |
250 mg/L |
16 IU/L |
Standard infant formula (8 oz) |
130 mg |
100 IU |
Fortified milk (8 oz) |
300 mg |
100 IU |
Fortified almond or soy milk (8 oz) |
300 mg |
100 IU |
Cheese (1 oz) |
200–300 mg |
30 IU |
Yogurt (6 oz) |
250 mg |
200 IU |
Ice cream (4 oz) |
100 mg |
– |
Fortified tofu (4 oz) |
435 mg |
50 IU |
Fortified juice (8 oz) |
300 mg |
100 IU |
Fortified cereal (1 serving) |
1,000 mg |
400 IU |
Egg (1) |
43 mg |
20–40 IU |
Almonds (24 nuts) |
70 mg |
– |
Dark leafy vegetables (4 oz cooked) |
50–135 mg |
– |
Beans (4 oz) |
60–80 mg |
– |
Canned fish with bones (salmon, mackerel, sardine, tuna) 3.5 oz |
70–100 mg |
230–600 IU |
Fresh salmon, wild (3.5 oz) |
– |
600–1,000 IU |
Fresh salmon, farmed (3.5 oz) |
– |
100–250 IU |
Both vitamin D2 and vitamin D3 are converted in the liver to 25-hydroxyvitamin D [25(OH)D], also known as calcidiol, which is the primary storage form and primary metabolite of vitamin D that circulates in human blood. Because of its long half-life and direct relationship to vitamin D exposure and supply, 25(OH)D is considered to be the best indicator of total body vitamin D status [22–24]. The metabolite, 25(OH)D, is converted to the activated form of vitamin D, 1,25-dihydroxyvitamin D [1,25(OH)2D or calcitriol], by 1-alpha-hydroxylase (CYP27B1) in the kidney [1]. Calcitriol synthesis comprises a tightly regulated process controlled primarily by PTH and fibroblast growth factor 23 (FGF23). PTH acts to increase calcitriol levels by up-regulating 1-alpha-hydroxylase, whereas FGF23 down-regulates this enzyme’s activity and subsequently decreases circulating calcitriol levels. In an important feedback loop, calcitriol can then suppress PTH secretion via action on VDRs in parathyroid cells [25]. In addition, calcitriol functions to regulate itself by ensuring a net positive calcium balance through increased calcium availability, which subsequently decreases the stimulus for PTH secretion. The metabolism of vitamin D is illustrated in Fig. 10.2.
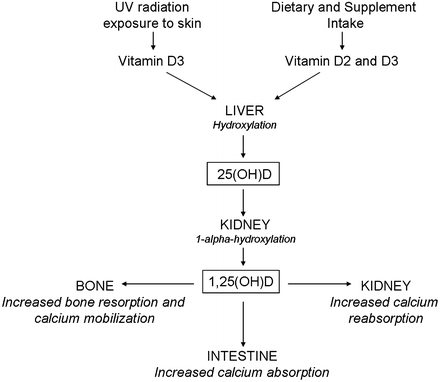
Fig. 10.2
The metabolism of vitamin D and its major effects on calcium regulation
The primary role of calcitriol is to maintain calcium and phosphate homeostasis. Through interaction with the VDR in the nuclei of target cells, calcitriol acts to increase the availability of calcium in the blood by three primary mechanisms: (1) stimulation of intestinal calcium absorption through active transport processes (2), mobilization of calcium from bone (with PTH), and (3) increased renal distal tubule calcium reabsorption. Calcitriol also affects phosphate metabolism, though this mechanism of action is less clear. Calcitriol stimulates increased intestinal phosphate absorption along with calcium and also induces FGF23 synthesis by osteocytes, thus exerting both positive and negative effects on phosphate levels.
As detailed above, active calcium absorption in the intestine is mediated by calcitriol action on VDRs among intestinal cells. In adults, studies have suggested that intestinal calcium absorption varies by serum 25(OH)D level, ranging from 10 to 15 % without vitamin D present, to 30–40 % in vitamin D sufficient states [26, 27]. During puberty, an increase in calcitriol production allows for increased intestinal calcium absorption to ensure adequate calcium availability during peak bone accrual that occurs during this period of rapid growth [28, 29].
Calcitriol also acts in concert with PTH to mobilize calcium from bone by stimulating bone resorption via inducing the expression of the receptor activator of nuclear factor kappa-B (RANK) ligand, which induces osteoclast differentiation and activation [30]. There may also be a possible direct anti-resorptive effect of calcitriol on bone [31]. At the same time, calcitriol positively affects bone mineralization by ensuring the availability of calcium and phosphate through the above-mentioned intestinal and renal effects on the absorption of these nutrients.
Effects of Calcium and Vitamin D on the Skeleton
Recently, calcium and vitamin D have received significant attention regarding their role in pediatric and adult bone health. The amount of vitamin D and/or calcium supplementation required for optimal bone accrual and maintenance, as well as for the prevention of bone loss across the age spectrum, is controversial. In addition, the optimal 25(OH)D concentration to ensure skeletal health, including at what level bone turnover markers, PTH, and gut calcium absorption are optimized, is under debate.
Despite these uncertainties, it is well known that calcium and vitamin D are important to maintain skeletal integrity. This point is perhaps best illustrated by the physiological changes noted within the setting of calcium and/or vitamin D deficiency. When a negative calcium balance occurs, PTH secretion is stimulated, leading to increased calcitriol production, which in turn stimulates intestinal and renal calcium absorption. Increased bone resorption from enhanced osteoclastogenesis may also ensue. This secondary hyperparathyroidism maintains normal blood calcium concentrations, but does so at the expense of calcium loss from bone. Thus, vitamin D and/or calcium deficiency can cause secondary osteoporosis, and in more severe cases, deficiency can result in inadequate mineralization of osteoid, leading to rickets in children and osteomalacia in adults.
Rickets and Osteomalacia
Calcipenic rickets occurs in the setting of insufficient calcium substrate to accommodate the needs of the growing pediatric skeleton. This condition is marked by defective mineralization of cartilage in the epiphyseal growth plates and failure of normal mineralization of newly formed osteoid. Classic features of rickets include widening of the long bone epiphyses, progressive bowing of the limbs (genu varus or valgus), craniotabes, delayed closure of fontanelles, parietal and frontal bossing, rachitic rosary from enlargement of the costochondral junctions of the ribs, hypoplasia of dental enamel, muscle weakness, and bone pain. In severe cases, hypocalcemia can result in seizures, particularly in infants less than a year of age [32].
Nutritional rickets can be due to inadequate calcium and/or phosphorus, insufficient vitamin D (from diet and/or sun exposure), or a combination of both. Low calcium intake of less than 200–300 mg/day has been associated with an increased risk of rickets, although the exact amount of calcium intake required to prevent rickets is unclear [33]. Similarly, the degree of vitamin D deficiency associated with the development of nutritional rickets is not well defined. However, in a recent review, a serum 25(OH)D concentration below 11 ng/mL (27.5 nmol/L) was found to be consistently associated with rickets, although the level above which rickets did not occur could not be determined [34]. Concurrent low calcium intake may also increase the level of vitamin D required for rickets prevention.
Nutritional rickets is a worldwide problem, most common in underdeveloped nations, but also occurring in industrialized countries. Although rickets may be less prevalent in the United States, one review noted at least 166 case reports of nutritional rickets identified between 1986 and 2003 [35], and the incidence appears to have risen over the past decade [36]. Patients at risk for rickets include infants who are solely breast-fed (due to low amounts of vitamin D present within breast milk), children with dark skin pigmentation, premature infants, and malnourished children. In addition, children with chronic medical disorders may be at high risk for rickets, particularly those with gastrointestinal malabsorption, pancreatic insufficiency, renal insufficiency, liver disorders, significant burn injury, and receiving medications that alter vitamin D metabolism (i.e., anticonvulsant and antiretroviral medications) [32]. In children who have sustained burn injuries, cutaneous vitamin D synthesis is not normal even a year after the burn. Interestingly, unburned adjacent skin has similarly decreased conversion of 7-dehydrocholesterol to 1,25-dihydroxyvitamin D3 [37]. Moreover, the vitamin D deficiency after the burn injuries is progressive, with low 25(OH)D levels noted 2 years postburn, while 1,25(OH)2D levels are normal [38].
Underlying genetic abnormalities can also result in the development of rickets, either by affecting the production of calcitriol or by inducing resistance to the actions of vitamin D. For example, vitamin D-dependent rickets type 1 is an autosomal recessive disorder that causes abnormally low activity of 1-alpha-hydroxylase leading to low calcitriol levels. This results in the classic skeletal manifestations of rickets and can cause significant hypocalcemia, typically presenting in the first year of life. Treatment includes calcitriol administration along with adequate calcium intake [39, 40]. Hereditary vitamin D-resistant rickets, formerly known as vitamin D-dependent rickets type 2, is an autosomal recessive condition that causes calcitriol resistance due to a mutation in the VDR gene. Patients typically present in the first several years of life with skeletal findings, including rachitic changes and often alopecia [41, 42]. In these patients, increased calcium supplementation or intravenous calcium infusions may be effective in treating rickets [43–46]. In the setting of end-organ resistance to vitamin D, the fact that calcium alone can treat this condition illustrates the important point that one of the principal roles of vitamin D is related to its effects on calcium availability, and that calcium (along with phosphorus) may be more critical than vitamin D in regard to effects on bone.
After closure of the growth plates, severe calcium and/or vitamin D deficiency can result in defective mineralization referred to as osteomalacia. Histologically, the resulting bone is marked by an abundance of undermineralized osteoid. This is not an uncommon finding in the general adult population and contributes to increased bone fragility [47–50]. Like rickets, the specific serum 25(OH)D concentration that leads to the development of osteomalacia is unknown, although a recent study of postmortem bone biopsies and blood 25(OH)D levels among 675 adults, aged 20–100 years, suggested that osteomalacia is most likely to occur at a 25(OH)D level below 20 ng/mL and does not occur at levels above 30 ng/mL [51].
Treatment approaches for rickets and osteomalacia with calcium and vitamin D will be reviewed later in this chapter.
Calcium and Pediatric Bone Health
Calcium Requirements in the Pediatric Population
Bone accretion occurring in growing infants, children, and adolescents leads to varying calcium requirements across the age spectrum. Infancy in particular is a time of high calcium requirement, typically met with a combination of high intestinal calcium absorption and high calcium content in breast milk and/or formula. Premature infants require even higher amounts of calcium due to the high rate of growth and bone mineralization that occurs in the third trimester, and these infants typically require fortified formula with a dose of calcium of 80–120 mg/kg/day in addition to that received in milk, for a total of 200 mg/kg/day [52]. If this calcium need is not met, these infants are at risk for “osteopenia of prematurity” (previously known as “rickets of prematurity”). In term infants, total body calcium mass at birth is estimated at 30 g, increasing to approximately 80 g by 12 months of age [53, 54]. To account for this gain, calcium accretion rate approximates 100 mg/day during the first year of life [55], which is readily available in breast milk and infant formulas.
Calcium absorption in infants is initially primarily passive [56, 57] and then gradually involves more active transport [58]. Breast-fed infants have the highest calcium absorption rate at approximately 60 %, and this rate is somewhat lower in formula-fed infants at 30–40 %, compensated by the higher calcium content in formula compared to breast milk [19, 55, 59, 60]. According to the recent guidelines of the Institute of Medicine (IOM), the recommended daily allowance (RDA) for infants 0–6 months is 200 mg/day, and increases to 260 mg/day from 6 to 12 months as solid foods are introduced [61].
Bone growth and calcium accrual continue into childhood and reach a peak during adolescence. In children, average calcium accretion rate is estimated at approximately 140 mg/day, increasing to 140–160 mg/day as puberty approaches [55, 62, 63]. The pubertal growth spurt leads to increases in bone mass and size, such that up to 40 % of bone mass is acquired in a 3- to 5-year period [3, 64]. The average calcium accretion during this period varies by gender ranges from 90 to 200 mg/day [65] and can peak at 300–400 mg/day [66]. At the completion of puberty, a small amount of bone accretion may occur after the age of 20 years, although at a much lower rate [67], and peak bone mass is typically reached by age 30 years [68]. To accommodate this increased calcium requirement during adolescence, intestinal calcium absorption rises as puberty progresses, from approximately 28 % during childhood to 34 % during adolescence, and back down to 25 % thereafter [18]. This increased absorption is primarily mediated through rise in circulating PTH concentrations, leading to higher calcitriol levels [29]. In order to meet these calcium needs, the IOM has set the RDA for calcium as 700 mg/day in ages 1–3 years, 1,000 mg/day in 4–8 years, and 1,300 mg/day in 9–18 years [61].
Pediatric Studies Evaluating the Effects of Calcium on Bone Health
Although it is clear that insufficient calcium intake is harmful to skeletal health, whether calcium intake above this amount, particularly via calcium supplementation, is beneficial is less apparent. A majority of studies assessing calcium intake and bone health have been performed in adults, particularly focusing on postmenopausal women and patients with osteoporosis. These studies have confirmed the importance of adequate calcium intake in maintaining bone health in adults and have driven recommendations for daily calcium requirements. Although less well represented than in the adult literature, multiple studies evaluating calcium intake and bone health in pediatrics have been performed, providing important insights into our understanding of this complex issue.
As detailed above, calcium requirements in infants are primarily met in breast milk and formula, allowing for bone accrual to occur. Whether additional calcium during this period improves long-term bone health is unclear. In one study, premature infants receiving formula with 700 mg/L of calcium had greater bone mineral content (BMC) at a corrected gestational age of 3 and 9 months compared to those receiving formula with 350 mg/L [69]. However, other studies suggest that the effects of higher calcium intake on bone mass accrual in infancy may be transient [70, 71], and further studies are required.
More data are available assessing calcium interventions in childhood and adolescence. Multiple randomized controlled trials have been performed in this population, and the majority have suggested neutral or modest improvement in bone outcomes. Several studies have suggested that bone mineral density (BMD) and bone accretion are improved with calcium intake that is increased above a baseline of 900 mg/day [72–78]. However, other studies suggest that this may only be a transient effect [79–81]. For example, in a trial of adolescents in the United States ages 15–18 years, improvement in hip and forearm BMD was noted after calcium supplementation [78]. A 3-year randomized controlled trial evaluated the effect of 1,000 mg of calcium supplementation compared to placebo in twins, revealing increased BMD in prepubertal supplemented subjects, although not in pubertal or postpubertal subjects [72]. A meta-analysis of 19 trials involving 2,859 subjects treated with calcium supplementation at a dose of 300–1,200 mg daily showed a 1.7 % increase in upper limb BMD compared to placebo, without an effect on other sites [82, 83]. Overall, these studies suggest that increased calcium intake and supplementation may increase skeletal size and mineralization, although it is unclear whether the effect is sustained.
The effect of calcium supplementation on fractures in the pediatric population is also unclear. In the above-mentioned meta-analysis, there was uncertain benefit on fracture rate [82, 83]. Epidemiologic studies suggest that there is an inverse association between calcium intake and childhood fracture rate [84–88]. For example, one case–control study in 100 Caucasian girls aged 3–15 years with distal forearm fractures and 100 controls without fractures found that older girls with fractures reported lower calcium intake than controls [85]. Another study suggested a decreased odds ratio for fracture in children, mean age 14 years, with higher calcium intake [88].
The effect of calcium supplementation in childhood and adolescence on the future development of low bone density and osteoporosis in adulthood also remains to be elucidated. Although intuitively it would be reasonable to hypothesize that any intervention that could optimize the achievement of peak bone mass might improve bone health into adulthood, this has not been proven to date in prospective long-term interventional studies. In observational and retrospective studies, there may be a suggestion of support for this hypothesis. For example, BMD measured by pQCT of the dominant radius in postmenopausal women correlated with retrospective recall of dietary history of calcium intake in childhood [89]. Given the limits of this type of data, the question remains as to the long-term efficacy of calcium supplementation on future osteoporosis risk.
Vitamin D and Bone Health in the Pediatric Population
Defining Vitamin D Deficiency and Sufficiency
The level of 25(OH)D required for the optimization and maintenance of bone health is controversial, and recommendations from different academic and professional medical societies vary. Many of the studies used in the determination of definitions for vitamin D deficiency and sufficiency have primarily involved adults, and data in the pediatric population are limited. In assessing optimal vitamin D status, studies have focused on determination of the PTH plateau (i.e., the 25(OH)D level at which PTH levels stabilize), calcium absorption studies, bone turnover markers, BMD measures, and fracture outcomes. Because many of these factors are affected by multiple confounders, such as concurrent calcium intake, age, and sunlight exposure, it has been difficult to establish consensus on this issue.
In adults, several studies have evaluated the association between serum 25(OH)D and PTH concentrations to determine whether there is a minimum 25(OH)D level that elicits a rise in PTH, as well as the maximum level of 25(OH)D at which PTH no longer declines. This is referred to as the PTH plateau. Initial influential studies in adults suggested that a PTH plateau occurs at a 25(OH)D level of 30 ng/mL (75 nmol/L) [90, 91]. However, this has been called into question, and more recent data suggest that there may not be an absolute threshold of 25(OH)D at which the PTH plateau occurs, but rather this may range from a 25(OH)D level as low as 12 ng/mL (30 nmol/L) to over 50 ng/mL (125 nmol/L) depending on multiple other confounding factors [92]. In the pediatric population, fewer studies have evaluated this question, and a clear point of PTH inflection based on 25(OH)D level has not yet been identified in children and adolescents [93].
Calcium absorption studies have also been used as a consideration for determining the ideal serum concentration of 25(OH)D that maximizes intestinal absorption of calcium, as this has important implications on calcium availability for bone health. In adults, a threshold of 32 ng/mL (80 nmol/L) was suggested as the level at which maximal efficacy in intestinal calcium absorption is reached [27]; however, other studies in adults have suggested that calcium absorption is not necessarily optimized at levels above 30 ng/mL [17, 94, 95]. Studies addressing this question in children are fewer. In one dual-labeled calcium absorption study in 251 children and adolescents ages 5–17 years, higher 25(OH)D level was not found to affect total or fractional calcium absorption in school-age children, and there was a modest effect of higher 25(OH)D on calcium absorption in early pubertal subjects [96]. In this study, highest calcium absorption was noted at the range of 11–20 ng/mL. Other pediatric studies have also suggested that calcium absorption may not necessarily be increased with the achievement of a higher 25(OH)D level [97, 98].
Adult studies have also illustrated that bone turnover markers are affected by circulating 25(OH)D level, such that higher 25(OH)D levels were associated with lower bone turnover markers such as serum osteocalcin and C-telopeptides, and urinary N-telopeptides [99]. In children, cross-sectional studies have similarly indicated that an inverse association exists between bone turnover markers and serum 25(OH)D, with lower markers of bone resorption with an improved vitamin D status [100–102]. In clinical trials of vitamin D supplementation in children and adolescents, effects on markers of bone turnover have been varied, with some reporting a decline in bone resorption markers [103], an increase in bone formation markers [104], or no change [105, 106].
Studies assessing associations between serum 25(OH)D concentration and both BMD and fracture risk have primarily focused on adults, with a particular emphasis on postmenopausal women because this population is at highest risk for osteoporosis and fracture. Although out of the scope of this chapter, observational data have supported the association of higher 25(OH)D with improved BMD and reduced fracture risk, although randomized controlled trials have yielded more variable results [34, 107]. Clinical studies assessing these outcomes in children, as well as how this affects future adult BMD and fracture risk, will be reviewed in more detail below.
Based on the above data regarding vitamin D and skeletal outcomes, the Institute of Medicine 2010 Report redefined vitamin D sufficiency in the healthy pediatric and adult population as a 25(OH)D level above 20 ng/mL and vitamin D insufficiency as less than 20 ng/mL [61], a level that is lower than many experts have recommended [22, 108, 109]. The IOM Committee interpreted available data as showing that higher serum 25(OH)D levels were not consistently associated with greater benefit, and possible U-shaped associations were seen with some outcomes suggesting that both high and low levels of 25(OH)D may be associated with greater risk of negative skeletal outcomes [61]. In contrast, The Endocrine Society published a Clinical Practice Guideline aimed at pediatric and adult individuals at risk for vitamin D deficiency or low bone density, defining vitamin D sufficiency in these patients as a 25(OH)D level above 30 ng/mL, vitamin D insufficiency as a level 20–30 ng/mL, and vitamin D deficiency as a level less than 20 ng/mL [110]. Future studies will be required to determine the long-term effects of these recommendations, particularly in children as they progress to adulthood.
Studies Evaluating Vitamin D and Bone Health in the Pediatric Population
In the following section, the important observational studies, randomized control trials, meta-analyses, and reviews involving infants, school-age children, and adolescents will be reviewed.
Infants
During development, fetal 25(OH)D concentrations are dependent on transplacental passage of vitamin D from the mother; therefore, 25(OH)D levels in neonates are directly related to mother’s vitamin D status. Apart from the previously presented data on rickets, the effects of vitamin D on bone health in infants and long-term repercussions are largely unknown. Studies in a relatively small number of infants have shown inconsistent results regarding associations between serum 25(OH)D levels and BMC in this age group [111–116]. Although some case–control studies suggest a direct association between BMC and 25(OH)D [113, 114], clinical trials have not confirmed this finding. In a randomized controlled trial of 18 breast-fed infants testing 400 IU as a supplementation dose, the treated infants had higher 25(OH)D levels and a transient increase in BMC at the radius at 12 weeks, although BMC changes did not persist at 26 weeks [111]. In a similar study in 46 infants, no difference in BMC was noted at 6 months [115]. Recently, a double-blind randomized clinical trial of 132 1-month old breast-fed infants receiving vitamin D supplementation at doses of 400, 800, 1,200, or 1,600 IU found that 97 % of all infants achieved a 25(OH)D level of 20 ng/mL after 3 and 12 months of supplementation regardless of dose [116]. There were no differences in growth or BMC between groups at 12 months. Only the dose of 1,600 IU daily led to 25(OH)D levels above 30 ng/mL at 3 months in 97.5 % of subjects; however, supplementation with this dose resulted in 25(OH)D levels that exceeded the healthy population target of 50 ng/mL, raising the possibility that this dose may lead to vitamin D toxicity in this population [116].
Long-term effects of vitamin D supplementation are also unclear. In one retrospective cohort study of 106 girls, higher BMD was noted at age 7–9 years in subjects who had received vitamin D supplementation during the first year of life [117] Similarly, whether low vitamin D levels are associated with increased fracture risk in infancy or in future childhood or adulthood has yet to be established. For example, a cross-sectional study of 380 healthy infants and toddlers found that 12 % had 25(OH)D <20 ng/mL, and of these 33 % had demineralization on wrist and knee radiographs [118]. In those 40 infants with 25(OH)D levels less than 20 ng/mL, none sustained a fracture after 2 years of follow-up [119]. Further studies evaluating the effects of vitamin D supplementation in infancy on bone health, fracture risk, and future peak bone mass are required.
Children and Adolescents
Observational studies in children and adolescents have suggested a direct association between serum 25(OH)D levels and BMC or BMD [100, 101, 120–122]. One of the largest cross-sectional studies in this population evaluating 576 12-year-olds and 489 15-year-olds showed that girls (although not boys) with higher 25(OH)D levels had significantly greater forearm BMD [101]. Vitamin D intake in adolescence has also been associated with higher adult BMD in males (although not females) [122]. 25(OH)D levels were directly associated with cortical BMD at the radius and tibia in another study of 10- to 12-year-old girls [100]. In the recent AHRQ review published in 2009, experts determined that there was fair evidence from observational studies in older children and adolescents to support a direct association between serum 25(OH)D levels and baseline BMD, as well as a change in BMD or BMC [107].
However, randomized control trials in this population have shown inconsistent results of vitamin D supplementation on bone density across different skeletal sites, ages, and gender [107]. For example, a trial of 26 healthy girls did not find an effect of supplementation with 400 IU vs. 800 IU daily of vitamin D for 1 year on BMC compared to placebo [123]. However, a larger study of 179 girls ages 10–17 years randomized to weekly oral vitamin D doses of 1,400 or 14,000 IU or placebo found that bone area and total hip BMC increased significantly after 1 year in the high dose group compared with the placebo and lower dose groups [124]. In a meta-analysis of available pediatric randomized clinical trials encompassing a total of 541 subjects receiving vitamin D supplementation and 343 controls [125], vitamin D supplementation did not have a statistically significant effect on total body BMC or hip or forearm BMD, although a slight effect (nonsignificant trend) was noted at the lumbar spine. However, in subjects with a low serum vitamin D (less than 14 ng/mL or 35 nmol/L), total body BMC and lumbar spine BMD had a significantly greater increase from baseline, by 2.6 % and 1.7 %, respectively, in supplemented subjects compared to placebo. Authors concluded that vitamin D supplementation was unlikely to be beneficial in pediatric subjects with normal vitamin D levels, but may result in clinically useful improvements in BMD in those with vitamin D deficiency [125]. Given the variability in these prior findings, further studies are needed. These conflicting results may also be due to varying corrections for bone size, or the lack thereof. Dual X-ray absorptiometry (DXA) measures in children can be confounded by short stature. Therefore, height will be an important variable for which the BMD Z-score should be adjusted in future clinical trials. In addition, studies utilizing quantitative computed tomography to evaluate three-dimensional bone geometry and strength may be helpful to evaluate the effect of vitamin D on growing bones.
The effect of vitamin D levels and supplementation on fracture risk in childhood and later in adulthood is also unclear. In one observational study, there was 50 % reduced risk of stress fracture in preadolescent and adolescent girls with higher vitamin D intake compared to those with lower intake [126]. However, these results have yet to be replicated in prospective trials of vitamin D supplementation.
In conclusion, a direct association between 25(OH)D and BMD or BMC in children and adolescents has been suggested in observational studies, but randomized clinical trials have not yet confirmed that vitamin D supplementation improves bone health in healthy children and adolescents. Further studies are needed to delineate the short- and long-term effects of vitamin D on future BMD and fracture risk in adulthood.
Calcium as a Pediatric Bone Drug
As detailed in Table 10.2, the IOM has developed recommendations for calcium intake in infants, children, and adolescents based on available calcium balance studies, with the tolerable upper limit of calcium set at 3,000 mg/day [61]. Although these recommendations are believed to account for the calcium requirements of the growing skeleton, it has yet to be confirmed that these recommendations will have long-term effects on BMD in children. However, these recommendations appear to be safe [127], and it is known that extremely low intakes (e.g., less than 600 mg/day) may put children at risk for inadequate calcium retention [128], suggesting that adequate intake (AI) at these levels could prevent inadequate mineralization.
Table 10.2
2010 Institute of Medicine report on dietary reference intakes for calcium and vitamin D
IOM recommendations for calcium and vitamin D intake by age [61] | ||||
Vitamin D |
Calcium | |||
RDA |
UL |
RDA |
UL | |
Infants | ||||
0–6 months |
400 IUa |
1,000 IU |
200 mga |
1,000 mg |
6–12 months |
400 IUa |
1,500 IU |
260 mga |
1,500 mg |
Children | ||||
1–3 years |
600 IU |
2,500 IU |
700 mg |
2,500 mg |
4–8 years |
600 IU |
3,000 IU |
1,000 mg |
2,500 mg |
Adolescents | ||||
9–13 years |
600 IU |
4,000 IU |
1,300 mg |
3,000 mg |
14–18 years |
600 IU |
4,000 IU |
1,300 mg |
3,000 mg |
Calcium within food is primarily found in dairy products such as milk, cheese, and yogurt, as well as some vegetables, legumes, fish, and grains. Foods such as orange juice and cereal may also be fortified with calcium (Table 10.1). Recent studies have suggested that the actual amount of dietary calcium intake in children, and particularly in adolescents, is likely significantly lower than the recommended amounts. In adolescents, calcium intake likely ranges between approximately 500–1,000 mg/day [18, 29, 129, 130], rather than the RDA of 1,300 mg/day. Calcium supplements are also an option for ensuring adequate calcium intake and optimizing bone health; however, calcium supplementation also is associated with both benefits and potential side effects.
Oral calcium supplements are primarily available as calcium carbonate and calcium citrate, and less commonly calcium glubionate. Intravenous forms, such as calcium gluconate and calcium chloride, may be used for the treatment of severe hypocalcemia or for stabilization of the myocardium during emergency situations. Calcium availability varies based on the form of calcium administered (Table 10.3), and oral amounts above 500 mg should be administered in divided doses due to limitations on the amount of calcium that can be absorbed at a given time [131]. The most common form of calcium supplement, calcium carbonate, contains approximately 40 % elemental calcium. Because acid is required for absorption, it is best taken with food and is less well absorbed with medications that reduce stomach acid (e.g., proton pump inhibitor or H2 blocker medications). Calcium citrate has a lower elemental calcium content of approximately 21 %, but this form may be better absorbed than calcium carbonate [131] and is less dependent on an acidic gastric environment, making it a better choice in patients on acid blockade [132–134]. Calcium glubionate has low elemental calcium content but is also available in a liquid form. Head-to-head trials comparing different forms of calcium supplements on bone outcomes are lacking, particularly in pediatrics.
Table 10.3
Types of calcium supplements and elemental calcium content
Type of calcium |
Route of administration |
Elemental calcium per 1 g calcium salt (mg) |
---|---|---|
Calcium carbonate |
Oral (tablet) |
400 |
Calcium citrate |
Oral (tablet) |
211 |
Calcium glubionate |
Oral (liquid) |
66 |
Calcium gluconate |
IV |
93 |
Calcium chloride |
IV |
273 |
Risks of Calcium Supplementation
Calcium supplementation may also be associated with risks, particularly when total calcium intake is excessive. These risks include hypercalcemia, which can lead to anorexia, weight loss, polyuria and dehydration, constipation, and renal insufficiency. Milk alkali syndrome is a condition marked by excess intake of calcium leading to hypercalcemia, metabolic alkalosis, and renal failure, typically seen at levels of intake greater than 3,000 mg/day [135–137]. Hypercalciuria can also develop with calcium supplementation, considered to be a urinary calcium level of above 250 mg/day in women, above 275–300 mg/day in men, and above 4 mg/kg/day in children over age 2 years. Hypercalciuria can predispose to nephrocalcinosis and nephrolithiasis. Although the development of kidney stones is often multifactorial related to different dietary and non-dietary factors, there may be an increased risk of nephrolithiasis with calcium supplementation in adults. For example, in the Women’s Health Initiative, where 36,000 postmenopausal women were randomized to calcium and vitamin D supplementation (1,000 mg/day and 400 IU/day, respectively) or placebo, the supplemented group had an increased risk of kidney stones (HR 1.17) [138]. Both supplemented and placebo groups in this study were noted to have a high baseline dietary intake of calcium of 1,100 mg/day, which may have contributed to this finding. Studies in children are limited, but two studies suggested that calcium supplementation does not significantly affect urinary calcium excretion at daily intakes (diet plus supplement) of 1,800 mg/day [139] or 1,560–1,740 mg/day [140]. In addition, because IV calcium gluconate formulations contain aluminum, prolonged administration of IV calcium gluconate may lead to aluminum toxicity, including osteomalacia and bone pain [141].
Recent studies in adults have also raised the question as to whether calcium supplementation may predispose to vascular and soft tissue calcification, potentially leading to increase in cardiovascular disease and higher mortality. For example, a recent meta-analysis evaluating 15 randomized controlled trials of calcium supplementation involving 20,000 adult participants suggested an increased risk of myocardial infarction with this intervention [142]. Although these data are not available in the pediatric population, this does raise concern regarding the advisability of routine calcium supplementation, and optimizing dietary calcium may be a safer approach than supplements.
Vitamin D as a Pediatric Bone Drug
As previously reviewed, the level of serum 25(OH)D at which bone health is optimized in the pediatric population remains controversial, and various professional societies have differed regarding the 25(OH)D level that represents sufficiency. Depending on the definition used, low vitamin D concentrations are common among individuals across the United States. Data from the 2001–2006 National Health and Nutrition Examination Survey showed that 18 % of children ages 1–11 years had a 25(OH)D level less than 20 ng/mL, and 1 % had a level less than 10 ng/mL, although this varied by age, gender, and race [143]. Reasons for vitamin D deficiency most commonly include inadequate intake or sun exposure, and other potential causes may be inadequate absorption due to underlying gastrointestinal issues or malabsorption, accelerated metabolism (i.e., from antiepilectic drugs or certain HIV medications), impaired liver hydroxylation due to hepatic disease, impaired 1-alpha-hydroxyaltion due to renal disease, or excess adipose tissue leading to vitamin D sequestration in obese patients.
Vitamin D supplements are administered orally in the form of vitamin D2 and vitamin D3. These supplements can be taken on an empty stomach and are available in a liquid form or in pill or capsule form. In the United States, vitamin D is not currently available in intravenous or intramuscular formulations. In patients dependent on intravenous parental nutrition, these preparations typically contain multivitamins that include 400 IU/L of vitamin D, although this may not be adequate to prevent vitamin D deficiency in these patients [144].
Vitamin D2 vs. Vitamin D3
Although vitamin D2 and vitamin D3 are often considered bioequivalent and interchangeable, recent questions have been raised as to whether differences in efficacy may exist. As previously mentioned, these two forms of vitamin D differ based on side change structure (Fig. 10.1), but available evidence suggests that the steps involved in their metabolism and action are the same [1], including hydroxylation [145, 146], binding to vitamin D binding protein [147], and binding of their activated forms to VDR [148].
In adults, some clinical studies suggest equal potency between vitamin D2 and vitamin D3 [149, 150] whereas others have suggested that vitamin D3 may be more effective [151–153]. A recent meta-analysis of seven trials involving supplementation with vitamin D2 vs. D3 found a significantly greater overall change in 25(OH)D levels with vitamin D3 over vitamin D2; however, the studies included in this meta-analysis utilized a variety of supplementation regimens ranging from a single very high dose of vitamin D to daily supplementation with lower doses [154]. When studies evaluating only daily supplementation regimens were evaluated, no significant difference between D2 and D3 was noted, suggesting that this difference in efficacy may only be present at very high doses of vitamin D.
Pediatric studies evaluating vitamin D2 and D3 regimens have been fewer and have also come to varying conclusions regarding the efficacy of these formulations. In 40 otherwise healthy infants and toddlers with serum 25(OH)D levels below 20 ng/mL, three different supplementation regimens (vitamin D2 50,000 IU weekly, vitamin D2 2,000 IU daily, or vitamin D3 2,000 IU daily) resulted in a similar tripling of serum 25(OH)D over a 6-week period, without significant differences between regimens [155]. In contrast, in a study of children and adolescents ages 5–20 years with underlying inflammatory bowel disease, the authors found that a regimen of vitamin D3 2,000 IU daily and vitamin D2 50,000 IU weekly was superior to vitamin D2 2,000 IU daily in raising 25(OH)D [156]. Whether compliance differences were at play, especially among the adolescent subjects, is unclear.
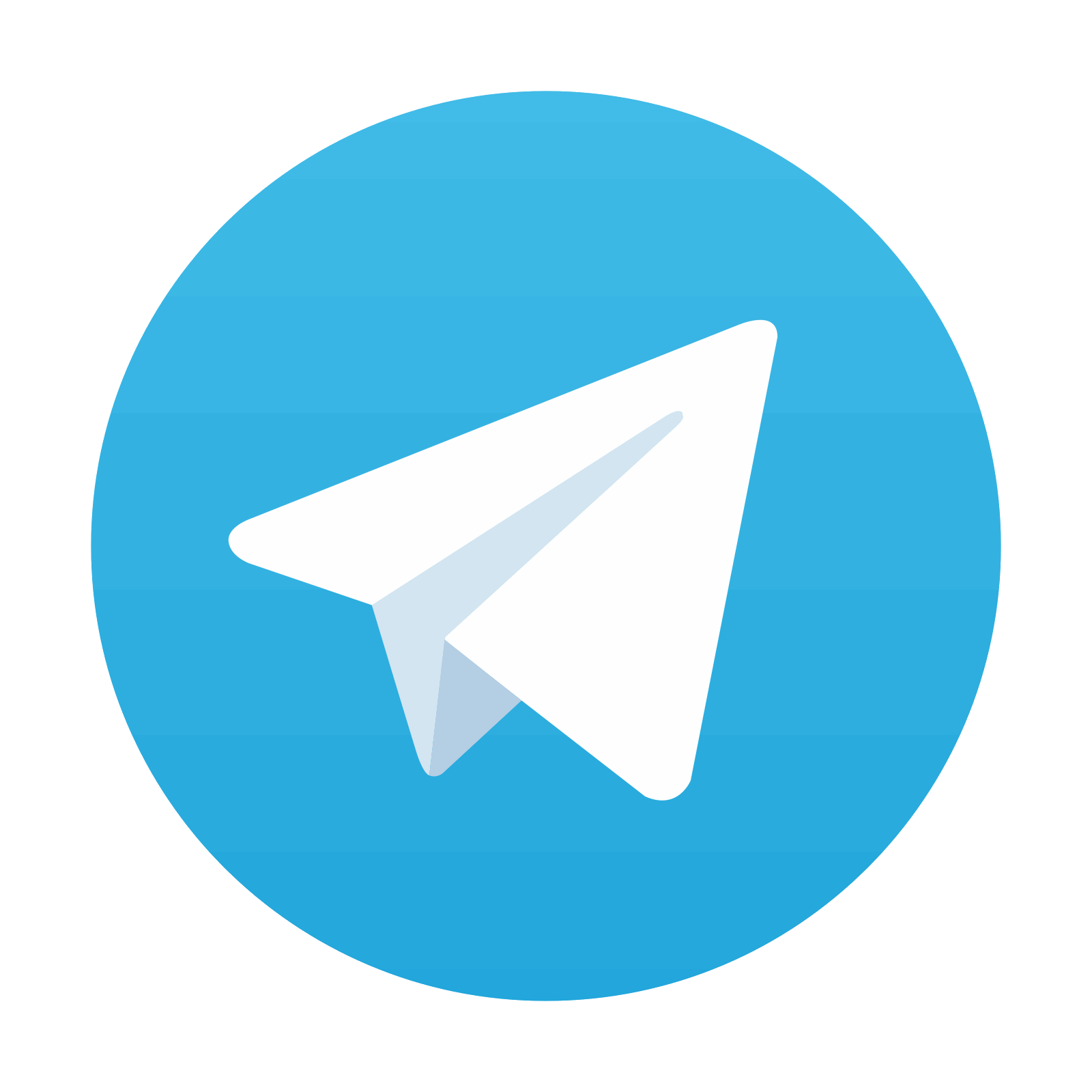
Stay updated, free articles. Join our Telegram channel

Full access? Get Clinical Tree
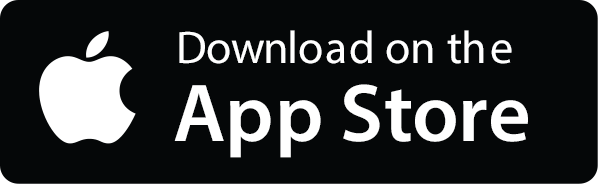
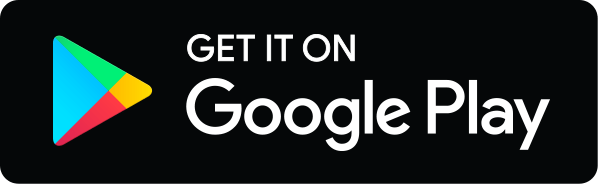