© Springer Science+Business Media Singapore 2017
Ikuo Konishi (ed.)Precision Medicine in Gynecology and ObstetricsComprehensive Gynecology and Obstetrics10.1007/978-981-10-2489-4_1414. Pathogenesis of Preeclampsia
(1)
Department of Gynecology and Obstetrics, Kyoto University Graduate School of Medicine, Kyoto, Japan
Abstract
Preeclampsia is a leading cause of maternal and neonatal morbidity and mortality. Preeclampsia is typified by a systemic inflammatory state and impaired endothelial function. As a systemic inflammation increases with advancing gestation even in normal pregnancy, pregnancy is thought to be a “stress test for endothelial function” for women. The etiology of preeclampsia remains largely unknown, and a number of theories have been proposed to explain its cause. It has been postulated that poor placentation results in the release of factors that lead to excessive maternal inflammatory response and endothelial dysfunction. However, the heterogeneous clinical manifestations of the disease suggest multifactorial pathogenesis of preeclampsia, such as poor placentation, oxidative stress, inflammation, immune maladaptation, and angiogenic imbalance. This chapter covers recent progress in molecular studies of the pathogenesis of preeclampsia.
Keywords
Endothelial functionPathogenesisPlacentationPreeclampsia14.1 Introduction
Preeclampsia is the most common hypertensive disorder of pregnancy, affecting 2–8% of pregnant women [1]. Preeclampsia is a syndrome of new-onset hypertension and proteinuria typically presenting after 20 weeks of gestation. Preeclampsia can lead to serious maternal complications, such as pulmonary edema, HELLP syndrome, placental abruption, eclampsia, and intracerebral hemorrhage. Preeclampsia is also associated with adverse fetal conditions such as growth restriction, oligohydramnios, and absent or reversed end-diastolic velocity in the umbilical artery. Consequently, preeclampsia is a leading cause of maternal and neonatal morbidity and mortality [1]. While it is well known that the placenta is essential to the development of preeclampsia, the heterogeneous clinical manifestations of the disease suggest multifactorial pathogenesis of preeclampsia. Because the pathogenesis has not been fully elucidated, prevention is not possible, and, even today, the fundamental therapy is to terminate the pregnancy. However, recent progress on genomic analyses using the placenta has gradually revealed molecular mechanisms underlying the pathogenesis of preeclampsia.
14.2 Two-Stage Hypothesis
The etiology of preeclampsia remains unknown, and a number of theories have been proposed to explain its cause. Zweifel described preeclampsia as a “disease of theories” [2]. Preeclampsia is clinically heterogeneous in its manifestation including the onset of the disease and the fetal growth. Abnormal trophoblast invasion with insufficient uterine spiral artery remodeling often occurs in early-onset preeclampsia and fetal growth restriction [3]. In contrast, normal spiral artery remodeling is usually observed in late-onset preeclampsia with normal fetal growth. So, Ness and Roberts hypothesized that there are distinct origins of preeclampsia, and both poor placentation (placental preeclampsia) and latent vascular dysfunction (maternal preeclampsia) are attributed to the development of preeclampsia [4]. Abnormal placentation is hypothesized to be associated with early-onset preeclampsia with fetal growth restriction. It has been postulated that poor placentation (stage 1) results in the release of factors that lead to excessive maternal inflammatory response and endothelial dysfunction (stage 2) [5, 6]. Thus, in early-onset preeclampsia, the sequence of abnormal trophoblast invasion to systemic endothelial dysfunction has been proposed to occur in two stages. On the other hand, preexisting maternal endothelial dysfunction (as with chronic hypertension, obesity, or diabetes mellitus) is thought to predispose to late-onset preeclampsia, which is more often linked to a normal placenta and normal fetal growth [5, 6]. As a systemic inflammation increases with advancing gestation even in normal pregnancy, pregnancy is a “stress test for endothelial function” for women. This burden to latent vascular dysfunction is enough to induce a severe systemic inflammatory response and subsequent endothelial dysfunction that is characteristic of preeclampsia [6].
14.3 Abnormal Trophoblast Invasion
Poor placentation is typified by insufficient remodeling of spiral arteries [7–9]. During normal placentation, extravillous trophoblasts (EVTs) invade into the decidua and the inner one third of the myometrium either interstitially or via spiral arteries. EVTs disrupt the endothelium and the smooth muscle layer and replace the vascular wall. These conversions allow spiral arteries to get widely dilated independently of vasomotor control, thereby providing enough blood supply in intervillous space (IVS) to meet the requirements of the fetus [7–9]. On the other hand, in preeclampsia, endovascular trophoblast invasion is restricted to the decidual segments of the spiral arteries, resulting in less dilated vessels and intermittent hypoperfusion due to retention of vasoactive smooth muscle [7–9]. Burton proposed that the impaired vascular remodeling of the spiral arteries may not cause chronic placental hypoxia in itself, but that the retention of smooth muscle will increase the risk of spontaneous vasoconstriction and ischemia-reperfusion injury, leading to generation of oxidative stress [10]. Moreover, high-velocity and turbulent flow into the IVS will damage villous syncytiotrophoblast [10], which may enhance placentally derived stimuli, including pro-inflammatory cytokines, anti-angiogenic factors, and trophoblast microparticles.
14.4 Hypoxia
Early placental development occurs in a low-oxygen environment, which appears to prevent trophoblast differentiation. As maternal blood flow into the IVS is blocked by endovascular plugs of extravillous trophoblasts prior to 10 weeks’ gestation, the oxygen concentration within the IVS is approximately 20 mmHg (2–3%) [11–13]. Once the plugs are displaced after 10–12 weeks’ gestation, chorionic villi are bathed in maternal blood. As a result, the oxygen concentration rises to 40–80 mmHg (5–10%) in the second trimester [11–13]. The oxygen concentration in the IVS gradually decreases thereafter to the third trimester (40 mmHg) in response to increased fetal oxygen consumption [11–13]. In preeclamptic placenta, the oxygen concentrations in the IVS are generally thought to be low because of defective vascular remodeling of the spiral arteries. However, to date there has been no direct evidence to support this assumption. Burton proposed that there was a slight alteration of total blood flow in the IVS due to insufficient spiral artery remodeling [10]. Moreover, the impairment of villous syncytiotrophoblasts will result in reduced oxygen removal in the IVS, which leads to placental hyperoxia and fetal hypoxia. So, it is unclear whether preeclamptic placentas are actually hypoxic [12], and it is plausible that exaggerated oxidative stress in preeclampsia is secondary to intermittent hypoperfusion rather than chronic placental hypoxia.
14.5 Hypoxia-Inducible Factors
The hypoxia-inducible factors (HIFs) are central mediators of cellular adaptation to low oxygen and regulate placental development and maturation [12, 14, 15]. For instance, HIF-1 regulates TGF-beta3, which inhibits trophoblast differentiation and invasion and is overexpressed in preeclamptic placentas [14]. Transcription of HIF-1alpha and HIF-2alpha is differentially regulated under hypoxia in neuroblastoma [16]. HIF-1alpha protein is transiently stabilized under hypoxia (1% O2), while HIF-2alpha protein gradually accumulates and controls prolonged hypoxic gene activation. Moreover, HIF-2alpha is increased to a greater extent than HIF-1alpha under mild hypoxia (5% O2). In the placenta, inconclusive results have been reported regarding HIF regulation in response to acute versus prolonged hypoxia [12]. Intriguingly, HIFs are activated through oxygen-independent factors including hormones (angiotensin II), cytokines (IL-1b, TNF-alpha, and NF-kB), and growth factors (TGF-b and IGF) [12, 15], and activation of HIFs during pregnancy is considered to play an important role in the pathogenesis of preeclampsia.
14.6 Oxidative Stress
Oxidative stress arises when the production of reactive oxygen species (ROS) exceeds the intrinsic antioxidant defenses [17]. ROS, such as superoxide anion, hydrogen peroxide, and hydroxyl radical, are chemically reactive molecules containing oxygen. ROS arise from the various sources such as mitochondria and cause cellular damage. The placenta is rich in mitochondria, is highly vascular, and is exposed to high maternal oxygen partial pressure, therefore resulting in increased production of ROS [18]. Indeed, increased production of lipid peroxides and oxidative stress are observed in normal pregnant women compared with nonpregnant women [19]. Moreover, preeclampsia is characterized by markedly decreased antioxidants such as glutathione or glutathione peroxidase activity, as well as increased oxidative stress [19, 20]. The higher production of oxidative stress in preeclampsia is probably due to insufficient remodeling of spiral arteries in the section of myometrial segments. Fluctuations in maternal blood flow to the placenta are thought to cause exaggerated oxidative stress. Consistently, labor, in which the placenta is exposed to repeated episodes of ischemia-reperfusion, produces high levels of oxidative stress [17, 21, 22].
Oxidative stress is one of many forms of cellular stress. ROS can activate redox-sensitive transcription factors (i.e., NF-kB, p53, AP1) and protein kinases (i.e., ERK1/2, SAPK/JNK, p38 MAPK) as physiological adaptive changes to alterations in the environment aimed at restoring homeostasis [17]. At higher levels, ROS lead to more extensive and irreparable cell damage, resulting in apoptosis or necrosis. ROS induce release of Ca2+ from the endoplasmic reticulum (ER) and are closely associated with mitochondrial and ER function [17]. Thus, oxidative stress rarely occurs in isolation, but is usually accompanied with other forms of cell responses, such as ER stress and unfolded protein responses [17, 23]. Placental oxidative stress causes diverse stress responses of syncytiotrophoblast including increased apoptosis and secretion of anti-angiogenic and pro-inflammatory products [6] and is thought to be an intermediary step in the pathogenesis of preeclampsia.
14.7 Inflammation
Inflammatory response is already well developed in normal pregnancy [24], and preeclampsia is a state of an exaggerated inflammatory response including abnormal cytokine production and neutrophil and endothelial cell activation [25]. Both normal and preeclamptic pregnancies exhibit an increased inflammatory response with advancing gestation [26]. There is now ample evidence to support that increased inflammatory response is associated with clinical manifestations of preeclampsia in animal models. For instance, chronic administration of endotoxin [27], TNF-alpha [28], IL-6 [29], and LPS [30] into pregnant rats causes preeclampsia-like symptoms. Activated neutrophils could cause vascular damage with activation of coagulation and platelets [31]. In addition to neutrophils, other immune effectors that appear to play important roles in preeclampsia are cytokines, which are major initiators and mediators of inflammation and endothelial dysfunction [25, 32, 33]. Cytokines can be generally classified as pro- and anti-inflammatory. Cytokines such as IL-1, IL-2, IL-8, IL-18, TNF-alpha, and IFN-gamma are pro-inflammatory. The expression of IL-1, IL-2, IL-18, and TNF-alpha are elevated in the preeclamptic placentas [34–38]. The anti-inflammatory cytokines, IL-4 and IL-10, are also secreted by placental tissues. IL-10 is a potent suppressor of pro-inflammatory cytokines such as TNF-alpha and IFN-gamma, and its placental production is decreased in the preeclamptic placentas [39, 40]. Indeed, IL-10 knockout mice exhibit mild hypertension with fetal growth restriction [41], and hypoxia induces preeclampsia-like features in pregnant IL-10 knockout mice [42]. Although pro-inflammatory cytokines are produced by trophoblasts and also by macrophages and stromal cells of the placenta, the high pro-inflammatory cytokine levels seen in peripheral blood in preeclamptic pregnancies are believed to be caused in great part by monocytes [25, 43, 44]. It has been proposed that the syncytiotrophoblast microparticles (STBM) stimulate the production of the pro-inflammatory cytokines through the activation of monocytes [43, 44]. As markedly increased amounts of STBM are shed into the maternal circulation in preeclampsia [45], STBM impairs maternal vascular endothelial function [46]. Cytokines may contribute to an increased release of STBM by stimulating enhanced trophoblast apoptosis [47], bringing about a vicious cycle of an excess of pro-inflammatory cytokines and the increased shedding of STBM.
14.8 Immune Maladaptation
Maternal-fetal immune maladaptation is believed to be one of the underlying mechanisms that contribute to the development of preeclampsia.
14.8.1 Uterine Natural Killer Cells
Uterine natural killer (uNK) cells comprise approximately 70% of the decidual leukocyte population in early human pregnancy [48, 49]. uNK cells play a crucial role in trophoblast invasion and initiating spiral artery remodeling. uNK cells surround or infiltrate the vascular wall before trophoblast invasion, and the number of uNK cells is reduced in the second half of pregnancy [50]. uNK cells are a major source of IFN-gamma [51], which acts in an autocrine manner on uNK cells, stimulating further production of IFN-gamma, as well as angiogenic growth factors including angiopoietin-1 (Ang-1), Ang-2, TGF-beta1, and VEGF-C [52]. IFN-gamma inhibits extravillous trophoblast cell invasion. uNK cell-derived factors, such as MMP-2, MMP-7, and MMP-9, urokinase plasminogen activator, and Ang-2 are thought to be involved in remodeling of spiral arteries, leading to extensive disorganization and apoptotic loss of vascular smooth muscle cells and endothelial cells [50, 53–55]. uNK cell supernatants from 12 to 14 weeks’ gestation, but not 8–10 weeks, stimulate extravillous trophoblast invasion, suggesting that regulation of extravillous trophoblast invasion by uNK cells is dependent on gestational age [56].
14.8.2 Macrophages
Macrophages are the second most abundant leukocyte population in the decidua comprising approximately 20% of all decidual leukocytes [48]. The number of macrophages does not alter substantially with increasing gestational age. Decidual macrophages are thought to contribute not only to the process of apoptotic cell clearance but also to immune tolerance at the maternal-fetal interface. Decidual macrophages produce IL-10 and indoleamine 2,3-dioxygenase (IDO) [57, 58] and suppress IFN-gamma production by T cells in early pregnancy [59]. On the other hand, decidual macrophages secrete pro-inflammatory cytokines such as IL-6, IL-8, and TNF-alpha through HLA-G receptors [60]. Moreover, activated macrophages produce TNF-alpha and inhibit human cytotrophoblast invasiveness [61]. In preeclampsia, myometrial spiral arteries are surrounded by large numbers of macrophages in the absence of trophoblast invasion [62]. In contrast, in normal pregnancies, myometrial spiral arteries are surrounded by extravillous trophoblast cells with sparse macrophage infiltration. Because activated macrophages produce both TNF-alpha and IL-10, an imbalance of these opposing cytokines may be involved in the pathogenesis of preeclampsia [63].
14.8.3 Toll-like Receptors
Toll-like receptors (TLRs) play a key role in the innate immune system and appear to contribute to the development of preeclampsia. TLR4 expression is increased in interstitial trophoblasts of patients with preeclampsia [64]. In a rat model, TLR4 activation in the placenta is associated with poor placentation and preeclampsia-like syndrome [65], which are improved by inhibiting the TLR4 signaling [66]. In addition, TLR3 activation during pregnancy causes preeclampsia-like symptoms, which are exacerbated in pregnant IL-10 knockout mice [67].
14.8.4 The Complement System
The complement system plays a crucial role in innate immunity. The complement cascade is activated in normal pregnancy, and excessive complement activation is observed in preeclampsia [68–71]. It was reported that eculizumab, a humanized monoclonal antibody against terminal complement protein C5, was an effective treatment for a woman presenting with severe preeclampsia/HELLP syndrome at 26 weeks’ gestation [72], indicating that complement inhibition may be an effective treatment for severe preeclampsia/HELLP syndrome. Apart from adverse effects of complement activation, C1q is widely distributed in human decidual stroma and plays an important role in promoting trophoblast invasion [73]. C1q-deficient mice exhibit impaired trophoblast invasion and preeclampsia-like features [74].
14.9 Angiogenic Imbalance
Preeclampsia can be thought of as a two-stage disorder, and the second stage is typified by the placenta-derived soluble angiogenic and anti-angiogenic factors into the maternal circulation [75].
14.9.1 Placental Growth Factor
Placental growth factor (PlGF) is predominantly expressed by syncytiotrophoblast and exerts its biological function through the binding and activation of VEGF receptor 1 (also known as Flt-1), which is initially identified as a receptor for VEGF-A [76]. PlGF is expressed at a low level in endothelial cells and other organs including the heart, lung, thyroid, skeletal muscle, and adipose tissue [76]. In normal pregnancy, PlGF can be detected in the maternal circulation from 8 weeks’ gestation. Serum PlGF rises steadily to 29–32 weeks and falls thereafter until delivery [77]. The PlGF levels are significantly lower in the women who later develop preeclampsia than in the controls [77]. Statins have been shown to reverse various pathophysiologic pathways associated with preeclampsia, such as angiogenic imbalance and oxidative stress [78–80]. Pravastatin induces PlGF and improves features of preeclampsia in a mouse model [80]. It was recently reported that treatment with recombinant PlGF ameliorates preeclampsia-like symptoms in a primate model, as well as a rat model of preeclampsia produced by reduced uterine perfusion pressure [81, 82].
14.9.2 Soluble Fms-like Tyrosine Kinase
Soluble Flt-1 (sFlt-1), an antagonist of VEGF and PlGF, is highly expressed in the placenta. The extraplacental sources of circulating sFLT-1 include endothelial cells, peripheral blood mononuclear cells, and adipose tissue [83, 84]. In normal pregnancy, the sFlt-1 levels are stable during the early and middle stages of gestation, and there is a slow and steady increase beginning at 33–36 weeks [77]. sFlt-1 is increased in the placenta and serum of women with preeclampsia [85]. Exogenous administration of sFlt-1 into pregnant rats induces major features of preeclampsia [85], and elevated levels of sFlt-1 and decreased levels of PlGF are observed in a rat model of preeclampsia produced by reduced uterine perfusion pressure [86]. Extracorporeal removal of sFlt-1 contributes to a successful prolongation of pregnancy [87, 88]. The evidence suggests that excess circulating sFlt-1 is closely related to the pathogenesis of preeclampsia.
14.9.3 Soluble Endoglin
Endoglin (Eng) is a TGF-beta co-receptor that is expressed mainly on the surface of endothelial cells but is also expressed on placental syncytiotrophoblasts. Levels of soluble Eng (sEng), resulting from the cleavage of full-length Eng, are markedly elevated in preeclampsia [89]. sEng impairs binding of TGF-beta to its receptors and inhibits nitric oxide-dependent vasodilatation by the regulation of eNOS expression. Overexpression of sEng in pregnant rats induces preeclampsia-like syndrome, which is exacerbated by the coadministration of sFlt-1, leading to the development of HELLP syndrome and fetal growth restriction [89].
14.9.4 Angiogenic Biomarkers of Preeclampsia
An excess of sFlt-1 and sEng and lower PlGF are generally released into the circulation of preeclamptic patients. However, the altered levels of placenta-derived angiogenic markers are more pronounced in early-onset rather than in late-onset preeclampsia [90]. On the contrary, angiogenic biomarker profile may not differ among late-onset preeclampsia, gestational hypertension, and normotensive pregnancy [91]. These observations support that the angiogenic biomarkers can be used to predict early-onset preeclampsia, which develops differently from late-onset preeclampsia. With accumulating evidence that sFlt-1/PlGF ratio is a promising diagnostic and predictive biomarker, careful clinical examinations have been conducted to confirm their usefulness in the prediction of preeclampsia [92–94].
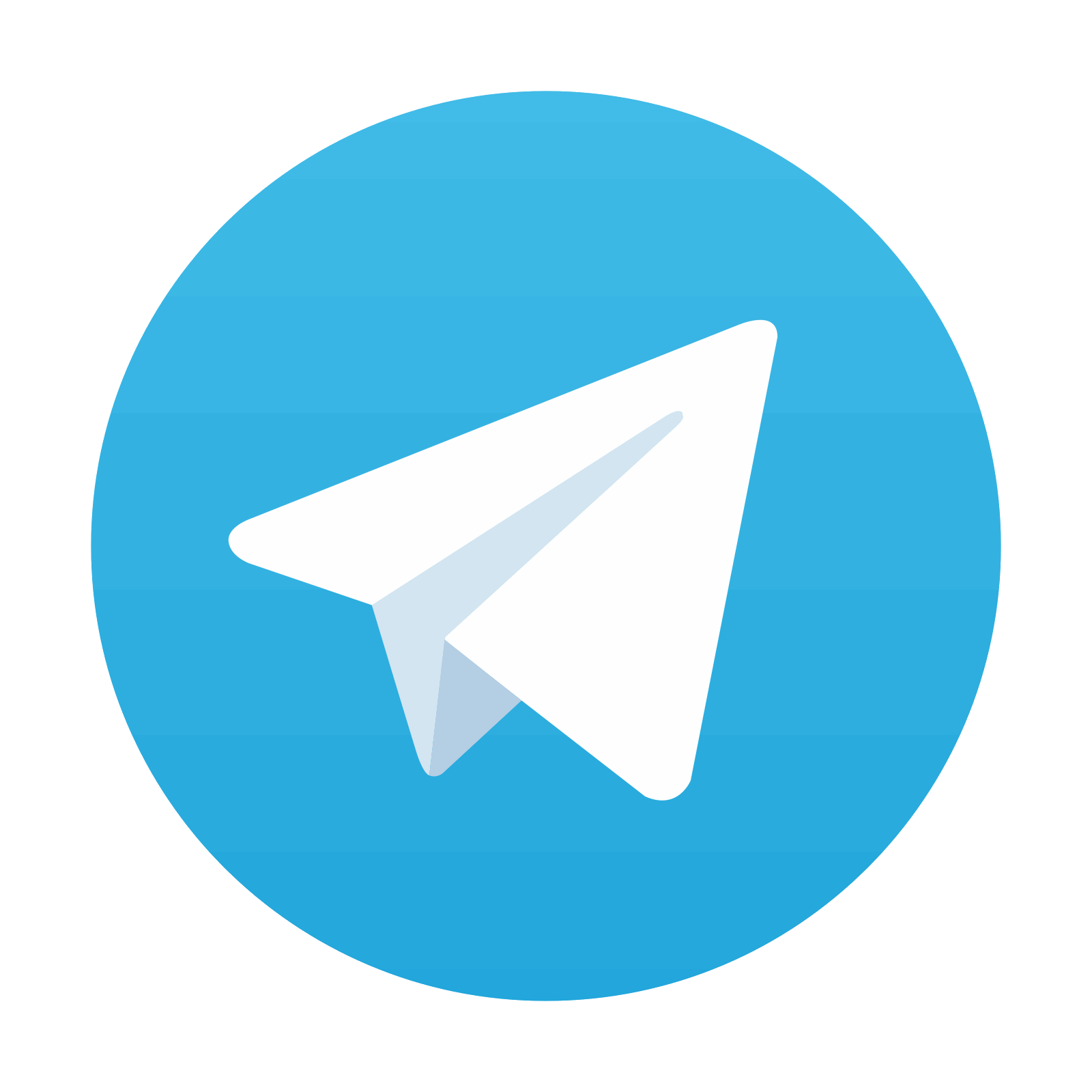
Stay updated, free articles. Join our Telegram channel

Full access? Get Clinical Tree
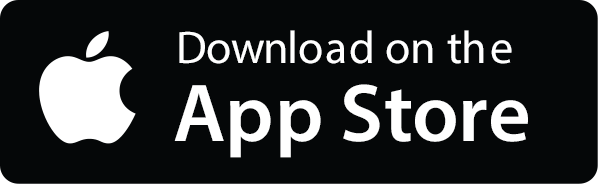
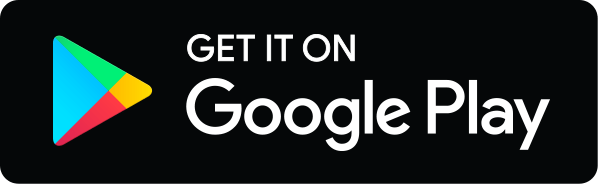