Omics and perinatal medicine
Preeclampsia
Piya Chaemsaithong and Liona C. Poon
Preeclampsia: Disease theory
Preeclampsia (PE) is a multisystem disorder of pregnancy traditionally characterized by the onset of hypertension with proteinuria after 20 weeks of gestation (1–7). This disorder typically affects 2%–5% of pregnant women and is one of the leading causes of maternal and perinatal morbidity and mortality, especially when the condition is of early-onset (8,9). Globally, 76,000 women and 500,000 babies die from this disorder each year (10).
PE is a disorder with multiple etiologies, and the underlying pathophysiology for the development of PE remains largely unknown. The current theory comprises two pathologic events (also called the “two-hit hypothesis”); the first hit is caused by shallow invasion of the trophoblast resulting in inadequate remodeling of the spiral arteries (1,11). This is presumed to lead to the second hit, which involves the maternal response to endothelial dysfunction and imbalance between angiogenic and antiangiogenic factors, causing the clinical features of the disorder (12). The current paradigm of PE management relies on clinical phenotypes (i.e., the presence or absence of maternal/fetal compromise, timing of onset of PE), which convey little information about the underlying mechanisms that lead to the development of the disorder. Therefore, this hampers the process of establishing effective methods of prediction, prevention, and treatment of PE.
The long-held belief of the two types of PE (maternal and placental) has originated from a growing body of evidence (13,14). Early-onset PE is more frequently associated with features of impaired placentation (15–19), including fetal growth restriction (20–23), abnormal uterine or umbilical arteries, Doppler velocimetry, and angiogenic/antiangiogenic imbalance (24–33). Specifically, placental lesions associated with maternal underperfusion have been observed in 70%–80% of pregnant women with early-onset PE, while these lesions have been found in only 30% of women with late-onset PE (18). The frequency of such placental lesions has an inverse relationship with gestational age at delivery for PE (18). Placental lesions of underperfusion could be consequences of shallow trophoblastic invasion and failure of transformation of the spiral arteries (34,35). Cardiovascular maladaptation and preexisting maternal cardiovascular risk factors are more frequently observed in late-onset PE (36–40). Women with late-onset PE have been observed to have higher stroke volume, cardiac output, and cardiac workload but lower peripheral vascular resistance than those with early-onset PE. Such changes can be detectable prior to the onset of the disorder and persist for at least 1 year postpartum (38). Nevertheless, classifying PE into maternal and placental subtypes is too simplistic, as there is significant overlap between the two. For example, the presence of maternal inflammation, as observed in obesity and diabetes mellitus, affects spiral artery remodeling and therefore placentation (41). In addition, there is no distinct gestational age cut-off that differentiates the presence or absence of placental lesions related to placental underperfusion, suggesting that placental underperfusion is a continuum phenomenon (18).
Other subclassifications of PE have been proposed such as those based on timing of delivery for PE (early-onset, PE requiring delivery <34 weeks; preterm, PE requiring delivery <37 weeks; late-onset PE, PE requiring delivery ≥34 weeks; term, PE requiring delivery ≥37 weeks). Nevertheless, none of these subclassifications have been validated. The aims of having the ideal classification of PE are to (1) improve the understanding of the mechanisms of disease, (2) facilitate biomarker discovery for effective prediction, and (3) develop targeted therapies for prevention and management. Biomarkers derived from the ideal PE classification might provide stronger associations with disease subtypes than those derived from when disease is considered as a single entity (42).
Current status and challenges in prediction and prevention of preeclampsia
The quest to predict PE effectively in the first trimester of pregnancy is driven by the need to identify women who are at high risk of developing the disorder, so that necessary measures can be initiated in early pregnancy to improve placentation and reduce the prevalence of the disease. The presence of a subclinical phase prior to clinical manifestation has allowed the development of prediction and prevention strategies (43). Further, the identification of high-risk women enables adequate antenatal surveillance that facilitates prompt diagnosis and management of PE.
Currently, recommendation on the identification of high-risk women for PE is based on a checklist of factors from maternal characteristics and medical and obstetric history, which is endorsed by several professional bodies such as the National Institute for Health and Care Excellence (NICE) in the United Kingdom (44) or the American College of Obstetricians and Gynecologists (ACOG) in the United States (45). Such an approach essentially treats each risk factor as a separate screening test with additive detection rate (DR) and screen positive rate (46). In screening with the use of NICE guidelines, the DRs are 39% for preterm PE and 34% for term PE, at 10% false-positive rate (FPR); in screening with the use of the U.S. Preventive Services Task Force (ACOG) list of risk factors, the DRs are 90% and 89%, at 64% FPR (46).
Extensive studies in the last decade have established that the best performance for early prediction of PE can be achieved by a combination of maternal characteristics, medical and obstetric history, together with the measurements of the mean arterial pressure (MAP), uterine artery pulsatility index (PI), and maternal serum placental growth factor (PlGF) at 11–13 weeks of gestation (first trimester combined test) (47,48). This first trimester combined test utilizes a novel survival-time model based on Bayes’ theorem to combine the prior information from maternal characteristics and medical and obstetric history with the biomarker multiple of the median (MoM) to estimate patient-specific risk for development of PE (47,48). This approach, within the framework of competing risks, is based on a continuous model for the gestation at delivery with PE, treating births from causes other than PE as censored observations (49,50). Using a risk cut-off of 1 in 100 for preterm PE, at a screen-positive rate of 10%, DRs for early-onset, preterm and term PE were 90%, 75%, and 45%, respectively (47,48). The screening performance of the first trimester combined test has been prospectively evaluated in several populations including Italian (51), Australian (52), American (53), Brazilian (54), and mixed European (55–59) populations. Almost all validation studies have reported comparable predictive performance corresponding to the original studies (52,54–59), except in the Italian and American populations (51,53). Currently an Asia-wide prospective validation study of the first trimester combined test is being undertaken (ClinicalTrials.gov Identifier: NCT03554681).
There is substantial evidence from the Aspirin for Evidence-based Preeclampsia Prevention (ASPRE) trial demonstrating that the rate of preterm PE can be reduced by 62% by aspirin at 150 mg per night administered from 11 to 14 until 36 weeks of gestation to high-risk women identified by the first trimester combined test as previously described (60). Furthermore, the latest systematic review and meta-analysis, including 16 randomized controlled trials with a total of 18,907 participants, have demonstrated that the administration of aspirin is associated with a reduction in the risk of preterm PE (relative risk [RR] 0.62; 95% confidence interval [CI]: 0.45–0.87) (61). Only the subgroup in which aspirin is started at ≤16 weeks of gestation at a dose of ≥100 mg/day is associated with a reduction in the frequency of preterm PE (RR 0.33; 95% CI: 0.19–0.57, p = 0.0001). Neither the initiation of aspirin at >16 weeks nor the daily dose of <100 mg is associated with a reduction in preterm or term PE (61). Based on such a high level of evidence, the International Federation of Gynecology and Obstetrics (FIGO) has endorsed the use of the first trimester combined test with maternal factors, MAP, uterine artery PI, and serum PlGF for the screening of preterm PE in all pregnant women. Prophylactic aspirin (at ∼150 mg per day) starting at 11–14+6 weeks’ gestation should be given to women who are considered as high risk for preterm PE (62).
It is apparent that first trimester prediction and prevention of PE is not as effective for term PE (27,63–65). Explanations responsible for such observations might be attributed to disease heterogeneity. Substantial effort has been continued to unravel, identify, and characterize the different subtypes of PE, with the aim to provide etiology-based prediction, prevention, therapy, and management with a personalized approach.
Recently, through the use of “high-dimensional biology” or “omics” technologies, a large number of molecular features and pathways have been described, and global changes in genome (DNA), transcriptome (messenger (m)RNA), metabolome (metabolites), lipidome (lipids), or proteome (proteins) have been examined simultaneously (66–70) (Figure 25.1). Since these approaches do not target specific molecules but profile all those that are measurable with a given platform, they are considered “unbiased” “discovery tools” (68,71). In this chapter, we explore how omics can help decipher the complexity of PE, improve the understanding of underlying etiologies, and guide clinical management.
Figure 25.1 “High-dimensional biology” or “omics technologies” that can examine a large number of molecular features and pathways including changes in genome (DNA), transcriptome (messenger RNA), proteome (proteins), metabolome (metabolites), or lipidome (lipids). (Source: Ref. 70.)
Role of omics in preeclampsia: Potential clinical application
An important feature of high-dimensional biology is that it is a discovery tool allowing detection of global changes in biological variables. This approach does not require a specific hypothesis since it is seemingly unbiased in nature; thus, it can be utilized in comprehensive examination of the biological processes involved in complex disorders, such as PE.
In the field of oncology, transcriptomic and/or multiomics profiling have been used to generate a molecular taxonomy of leukemia (72,73), breast cancer (74–77), pancreatic cancer (78), ovarian cancer (79), hepatocellular carcinoma (80,81), and prostate cancer (82,83). Prognosis and response to treatment vary according to the molecular classification (84–86). Some molecular biomarkers can be detected in peripheral blood and used to monitor disease progression (87,88). Omics has been shown to have the following roles in hypertension disorder: (1) diagnosis of rare forms of hypertension and classification of subtypes of adrenal tumor by the use of genomics approach (89); (2) prediction of hypertensive patients at risk for the development of target organ damage by the use of proteomics signature (90,91); (3) targeting therapy by the use of proteomics (92) or genome-wide association study (GWAS) (93); and (4) monitoring treatment response by determining proteomics or metabolomics signatures in response to antihypertensive medications (91,94). Research is currently being undertaken to establish molecular classification of hypertension, which can potentially help redefine the disease. We can adopt the same principle of omics for PE (Table 25.1):
Table 25.1 Application of omics technologies in preeclampsia research | ||||
Diagnosis of PE | Prediction and prevention of PE | Monitoring prophylactic treatment response | Prediction of PE complication | Molecular definition of PE |
•Describe the molecular makeup of individual PE patients •Identify inherited condition that increases risk of PE | •Identify genetic predisposition to PE, such as inherited thrombophilia •Discover omics biomarkers, networks and pathways for early prediction •Tailor early prevention to an individual’s molecular signature | •Identify aspirin nonresponsiveness by pharmacogenomics or metabolomics approaches •Tailor treatment options to an individual’s molecular signature | •Understand the link between PE and its complications later in life in both mother and offspring •Identify patients at highest risk for developing complications related to PE | •Deeper understanding of pathophysiology of PE •Redefine PE based on comprehensive molecular characterization of the disorder |
Abbreviation: PE, preeclampsia. |
1.Diagnosis of PE: Currently the diagnosis of PE is based on the measurement of blood pressure and clinical features. Omics data, especially genomics, can provide a deep insight into a specific individual medical condition. For example, polymorphism associated with thrombophilia increases the risk of PE (95) and therefore supplements established diagnosis and guides specific therapies. The role of other omics techniques for the diagnosis of PE might be limited since transcriptome, proteome, and metabolome change in response to disease process, making it difficult to differentiate between cause and consequence.
2.Prediction and prevention of PE: PE has a long subclinical phase during which alterations in biochemical and biophysical markers can be detectable. With deeper understanding of the underlying genetic determinants, more effective prediction of PE early in gestation is possible. The genetic background of an individual is the principal risk, and this can be modified by environmental factors, which are potential targets for preventive strategies, such as calcium supplementation (96) and lifestyle modification (97). It can be expected that the subclinical phase of PE is characterized by alterations in gene, protein, and metabolite expression. Detection of altered profiles using transcriptomics, proteomics, and metabolomics techniques could provide information as to which patients are on a trajectory toward overt PE. Few studies or case reports of the subclinical phase of PE showed that PE could be prevented or at least delayed in some patients, such as those with inherited thrombophilia (98,99). Thus, it is possible that interventions would be even more effective if they were administered earlier and at a stage when the molecular alterations toward PE are less advanced.
3.Monitoring prophylactic treatment response: Currently, prevention of early-onset PE is achievable by the administration of low-dose aspirin in high-risk women, defined by the first trimester combined test (60). Various tests have been used to assess aspirin response (100). However, such tests have not been standardized as a result of variable diagnostic cut-offs (101), limited correlation between the tests, and poor reproducibility (101,102). Pharmacometabolomics is an approach that leverages systems pharmacology to determine molecular pathways implicated in drug response. Profiles of changes in the metabolome can determine drug responsiveness and guide personalized treatment (103). In a healthy nonpregnant population, this approach has identified metabolomics signature of aspirin nonresponders determined by collagen-stimulated ex vivo platelet aggregation (104). Genetic markers will be of limited use in this respect because they are not sufficiently dynamic, but epigenetic, transcriptomics, proteomics, and metabolomics markers are suited to assess treatment response.
4.Prediction of complications of PE: Consequences of PE include cardiovascular, metabolic, and renal diseases later in life in the mothers and delayed neurodevelopmental outcome as well as metabolic diseases in the offspring (105). Early prediction of such complications and targeted preventive measures remains the “Holy Grail” in the management of patients with PE. Current clinical practice is guided by blood pressure values and simple clinical markers of organ damage such as albuminuria. Omics-based signatures may contribute in the determination of future target organ damage. To date, there are no studies providing evidence that omics signatures can be detected and linked to subclinical organ damage later in life in PE patients. This is a potential step toward early identification and treatment decisions.
5.Toward a molecular definition of PE: We have focused on the potential clinical application of omics technologies in the management of PE: diagnosis, early effective prediction and prevention of early-onset disease, prediction of its complications, and personalized treatment are all cornerstones of precision medicine and of clinical relevance. There is the additional aspect of a better understanding of the underlying pathophysiology of the disorder. It has been shown in other medical conditions, such as breast cancer, that a deeper understanding at the molecular level can help redefine the disease (74,75). It is anticipated that comprehensive molecular characterization of PE would define disease subtypes and lead us away from a diagnosis that merely relies on clinical features.
Genomics
A genetic predisposition to PE is a well-recognized risk factor for the disorder. Women with a positive family history have an estimated threefold increase in risk of developing PE compared to those without a family history (106,107). The presence of “PE susceptibility genes” or a combination of these genes or their alleles may increase the risk of PE by altering the regulation of blood pressure, placental function (108,109), and endothelial function (110). Existing proposed genetic models are either a single recessive gene or a single dominant gene (108,109). There have been reported associations between PE and polymorphisms of various genes such as angiotensinogen, TNF-α, factor V Leiden, and the 5,10-methylenetetrahydrofolate reductase genes (111–114).
In a recent systematic review of literature and meta-analysis (The Human Genome Epidemiology Review [115]), the authors have examined the association between maternal genotype and severe PE according to certain gene groups including immune, cell signaling, metabolic, thrombophilic, and vasoactive groups. They have analyzed 57 studies evaluating 50 genotypes in 5,049 cases and 16,989 controls and have found that an increased risk for severe PE is noted with coagulation factor V gene (proaccelerin, labile factor) polymorphism rs6025, coagulation factor II (thrombin) gene mutation G20210A, leptin receptor gene (LEPR) polymorphism rs1137100, and the thrombophilic gene group (115).
In another meta-analysis, the authors have specifically examined the relationship between the risk of PE and two thrombophilia single-nucleotide polymorphisms (SNPs), factor V G1691A SNP and prothrombin G20210A SNP. They examined 37 studies, including 5,048 patients with PE and 6,796 controls, and have reported that both of these polymorphisms are associated with an increased risk of all PE, including severe PE (95). The presence of a prothrombin 20210A polymorphism is associated with twofold and threefold increase in a patient’s risk of developing PE and severe PE, respectively. The authors have concluded that although associations between gene polymorphisms and PE have been illustrated, in order to explore this further and establish a causal relationship, there is a need for larger prospective studies.
The main disadvantage of the candidate gene approach is related to its lack of power to detect variants with small effects. Another limitation of the candidate gene approach is that it is dependent on the generation of a hypothesis based on our current limited knowledge of the pathophysiology of the disorder. Other limitations of existing studies include modest heterogeneity and various definitions of PE used. As a consequence, the results have been inconsistent. Until now, it is highly probable that no single gene is accountable for all the genetic risk in women with PE, and it is possible that different genetic variants are associated with various subtypes of the disorder.
Genome-wide screening is an approach that is not dependent on the knowledge of the pathophysiology of the disorder and has been a useful methodology in researching a wide range of common human genetic traits. Genome-wide linkage study (GWLS) using sib-pair analysis have identified plausible, yet conflicting, positional candidate maternal susceptibility genes for PE. GWLS of PE-affected families have demonstrated significant linkage on chromosome 2p, 2q, 4p, 7p, 9p, 10q, 11q, and 22q (116). None of these significant or suggestive loci have been reproduced in another GWLS study. A meta-analysis of five GWLS has advised that the results should be interpreted with caution as the statistical significance is only modest (116). Candidate genes identified from GWAS include copy number variants (CNV) showing an enrichment of deletions in 19q13.31 that encloses the pregnancy specific β-1-glycoprotein 11 gene (117), and three SNP associations on chromosome 2q14.2, a region near the inhibin β gene (118). However, none of these genome-wide SNPs have reached statistical significance (117–119). A European Union–funded project—INTERPREGGEN (Genetic studies of preeclampsia in Central Asian and European populations)—has identified nine low-frequency or rare variants using GWAS technique, which merit further evaluation (120).
A recent GWAS study in the offspring from PE pregnancies has reported that a single genome-wide significant susceptibility locus located on chromosome 13, near FLT1 gene (rs4769613), is significantly associated with PE (p = 3.2 × 10−8) in 2,658 PE offspring and 308,292 controls (121). This variant has been replicated in an independent data set (1,722 cases and 1,946 controls). Interestingly, rs4769613 has been observed more frequently in the offspring from pregnancy affected by late-onset PE and with appropriate birth weight than in those from pregnancy affected by early-onset PE and with birth weight less than the 10th percentile (odds ratio [OR] 1.26 p = 1.2 × 10−7 versus OR 1.03; p = 0.72) (121). This study has highlighted the potential importance of the fetal genomes in the determination of placental response to hypoxia-ischemia in PE.
Recent advances in massively parallel sequencing technologies have transformed the field of genetics study of human diseases. However, optimal approaches to discovering rare pathogenic variants in complex diseases remain unclear. While whole-genome sequencing is the technology of choice for investigating all genetic information, it is currently too expensive for large-scale studies. An alternative is exome sequencing, a technique that captures and sequences the exons in the genome, which are short functionally important sequences of DNA representing the regions in genes that are translated into protein (122). Studies using exome-sequencing technique have identified genetic variants in PE (118,123,124). A novel variant (rs180538), which resides within the LIG4 gene, a susceptibility gene for cardiovascular disease has been found, thereby indicating that PE and cardiovascular disease share common genetic factors (118). In another study, however, none of the identified variants from exome-sequencing technique determined in a Finnish preeclamptic cohort reached genome-wide significance (123). There are several limitations in the study, including the use of pool DNA samples, exclusive Finnish population, and the use of various genotyping methods such as exome sequencing and association analysis. Recently, low-frequency protective maternal genetic variants in FLT1, which contribute to lower PE risk, have been discovered (124). In this study, 124 genes that are enriched in PE, based on previous studies, were examined. The investigators performed targeted exome-sequencing in 500 women with PE and 190 matched normal pregnant women from the Finnish Genetics of Preeclampsia Consortium. Subsequently, 122 and 1,905 parous nonpregnant women with and without a history of PE from the National FINRISK study were genotyped by exome sequencing for 124 genes. The strongest associations observed are in FLT1 with two variants (rs35832528 and rs141440705) (124). FLT1 encodes soluble Fms-like tyrosine kinase 1 (sFLT-1), a splice variant of the vascular endothelial growth factor receptor that exerts an antiangiogenic property by inhibiting signaling of proangiogenic factors (125).
Transcriptomics
The transcriptome is characterized by the examination of the whole collection of RNA transcripts across a variety of biological conditions (126). Comprehensive transcriptome analysis has been utilized to investigate placental development and dysfunction in several pregnancy complications, including PE (127–130).
Previous research on transcriptome in PE has mainly focused on mRNA expression in the placenta (130). By integrating microarray data derived from the placentae of 89 preeclamptic women from six studies, 67 and 31 genes have been shown to upregulate and downregulate, respectively (131). The most consistently upregulated genes are LEP and FLT1 found in five studies; whereas, CLDN1 is the most consistently downregulated gene observed in all studies (131). Additionally, the authors have identified changes in several putative transcription factors involved in cell response to inflammation, hypoxia, DNA damage, and proliferation (131). Using a similar approach, Kleinrouweler et al. have determined meta-signatures of differentially expressed genes in third trimester placentae of 254 women with PE (132). Among the 1,343 differentially regulated genes, 40 gene transcripts are consistently differentially expressed in PE, of which most genes are upregulated (132). A recent systematic review with meta-analysis of microarray data using absolute gene expression approach in 167 placental samples, including 68 cases of PE and 99 cases of normal pregnancy, have demonstrated that expression of 88 genes has been identified in PE placentae (p < 0.005), ten of which (LEP, HTRA4, SPAG4, LHB, TREM1, FSTL3, CGB, INHA, PROCR, and LTF) are highly significant (p < 0.001) (133). Thus far, microarray analysis has significantly helped in improving the understanding of the underlying pathophysiology of PE. However, clinical application of transcriptomics based on traditional statistical approach for precise prediction and targeted treatment remains challenging as it relies on a binary method that separates the normal group from the disease group based on their gene expression profile (134).
An alternative approach that is currently used for disease molecular subtype classification is hierarchical clustering or unsupervised clustering. Examples of the use of transcriptomics for molecular classification and targeted treatment are derived from the field of oncology (75). Van’t Veer et al. demonstrated a specific gene-expression profile, which provided prognostic information about breast cancer (75). The expression patterns of 98 primary tumors from young patients with lymph node–negative breast cancer were analyzed with the use of oligonucleotide microarrays containing 25,000 genes. Information about clinical outcome in these patients was used in a statistical analysis called “supervised clustering” to identify a set of 70 genes with an expression pattern that allowed highly accurate classification of the patients into those with a poor prognosis and those with a good prognosis (Figure 25.2) (75). This 70-genes expression profile was proven to be a more powerful predictive tool of breast cancer outcome than the original system based on clinical and histologic criteria (74). This study highlighted the importance of hierarchical clustering or class discovery approach.
Figure 25.2 (a) Use of prognostic reporter genes to identify optimally two types of disease outcome from 78 sporadic breast tumors into poor prognosis and good prognosis groups. (b) Expression data matrix of 70 prognostic marker genes from tumors of 78 breast cancer patients (left panel). Each row represents a tumor and each column a gene, whose name is labeled between (b) and (c). Genes are ordered according to their correlation coefficient with the two prognostic groups. Tumors are ordered by the correlation to the average profile of the good prognosis group (middle panel). Solid line, prognostic classifier with optimal accuracy; dashed line, with optimized sensitivity. Above the dashed line, patients have a good prognosis signature; below the dashed line, the prognosis signature is poor. The metastasis status for each patient is shown in the right panel: white indicates patients who developed distant metastases within 5 years after the primary diagnosis; black indicates patients who continued to be disease-free for at least 5 years. (c) Same as for (b), but the expression data matrix is for tumors of 19 additional breast cancer patients using the same 70 optimal prognostic marker genes. Thresholds in the classifier (solid and dashed line) are the same as (b). (Source: Ref. 75.)
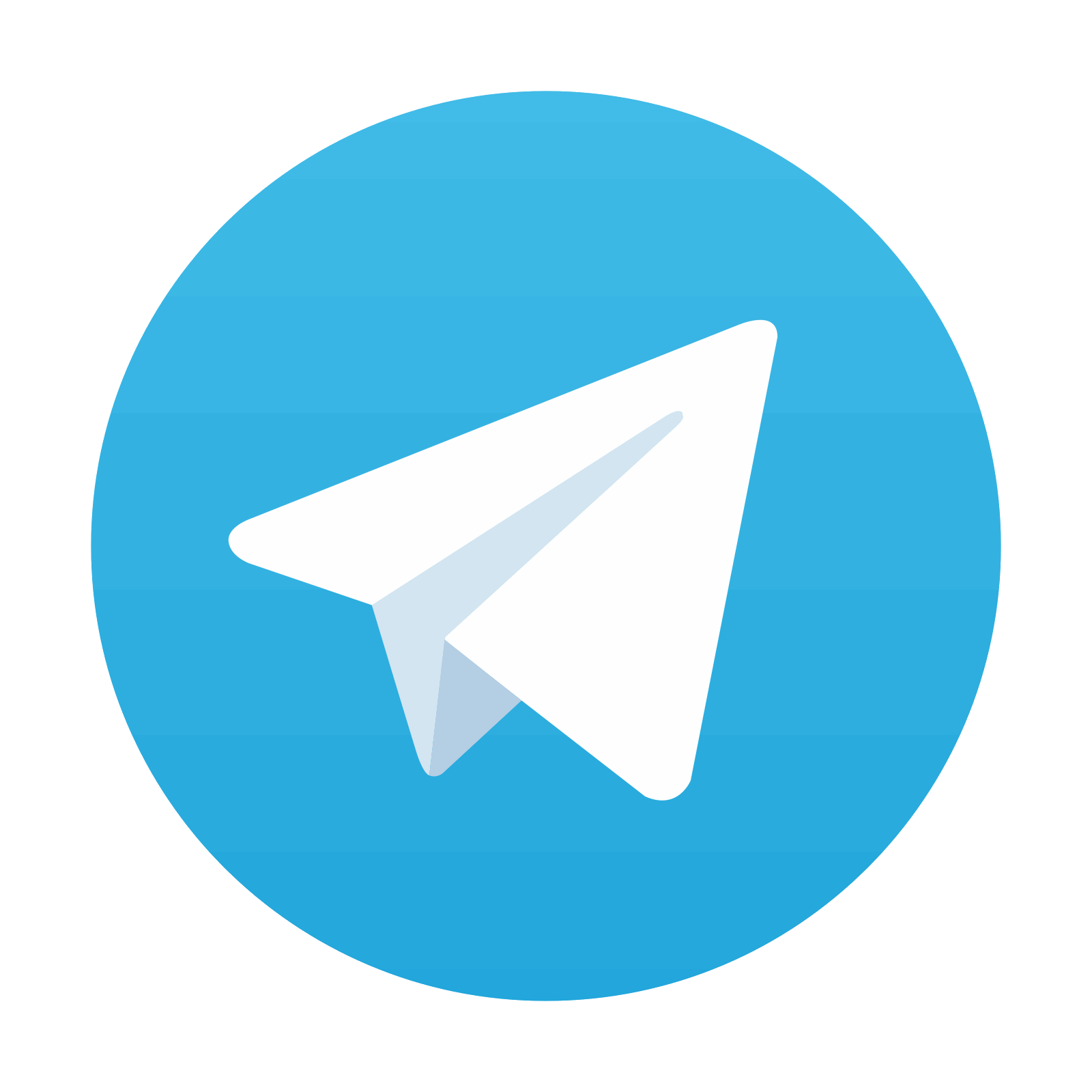
Stay updated, free articles. Join our Telegram channel

Full access? Get Clinical Tree
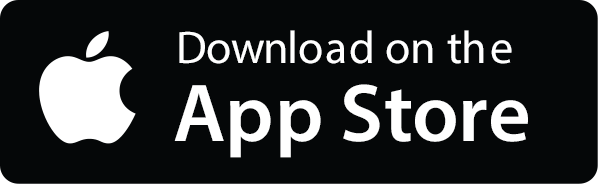
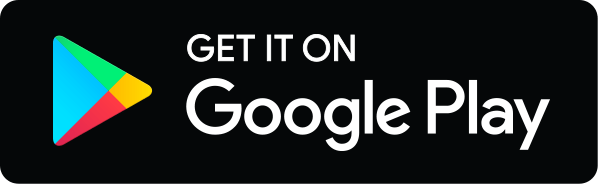