© Springer International Publishing Switzerland 2016
Mikel San-Julian (ed.)Cañadell’s Pediatric Bone Sarcomas10.1007/978-3-319-24220-0_22. Non-surgical Treatment of Pediatric Bone Sarcomas
(1)
Department of Pediatrics, University Clinic of Navarra, Avda. Pio XII s/n 31080, Pamplona, Navarra, E31080, Spain
(2)
Centro de salud de La Rochapea, Pamplona, Spain
(3)
Department of Pediatrics, Hospital San Joan de Deu, Barcelona, Spain
Abstract
The functional results of surgery are important, but only when survival of a patient is achieved. The key to success in the overall treatment of malignant bone tumors in children lies in multi-disciplinarity. A good response to chemotherapy together with function-preserving surgery of quality (that assures local control of the disease) are both fundamental to treatment success.
Keywords
ChemotherapyBone sarcomasRadiotherapyLocal ControlRelapseOsteosarcomaEwing’s SarcomaLimb Salvage2.1 Introduction
Pediatric bone sarcomas are malignant tumors of the bone. The optimum treatment of bone tumors affecting children and adolescents requires a multidisciplinary approach combining surgery, radiotherapy and chemotherapy. Treatment success is based on early diagnosis and on adequate experience of the medical team; for this reason it is advisable that these cases be treated in centers highly specialized in the management of this pathology [1, 2].
Not all bone tumors are malignant. Benign bone tumors are more common than malignant ones [3]. There are no epidemiology data specifically comparing pseudotumoral and benign bone lesions. Benign conditions are 100 times more frequent than malignant primary bone tumors. Simple bone cyst and Langerhans cells histiocytosis represent more than 50 % of all pseudotumoral lesions; typically, they affect children and teenagers with a median age of 12 years. With respect to benign lesions, the most frequent are the benign chondroblastic lesions (osteochondromas and chondromas) and osteoid osteoma. Aneurysmal bone cyst is also considered a benign entity.
Malignant bone tumors, with average rates in Europe of the order of 6–7 cases per million, generally represent between 5 and 7 % of childhood cancers. The two most frequent types are osteosarcomas and Ewing’s tumors. The former constitute a little more than half of bone tumors, the Ewing’s tumors over 40 %. Chondrosarcomas represent about 2 % (rates of the order of 0.1 cases per million). Bone tumors are very infrequent in babies and infants. Incidence increases with age, peaking in the age range of 10–14 years, in which a little over 10 % of all such tumors occur. Osteosarcomas are rare before 5 years of age. Until that age, Ewing’s tumors are slightly more frequent than osteosarcomas. The incidence of both types becomes practically equal in the 5–9 year-old group and, in the 10–14 year-old group the incidence of osteosarcoma (OS) overtakes that of the Ewing’s tumors. Ewing’s sarcomas (ES) are slightly more predominant in males [4].
2.2 Treatment of Osteosarcoma (OS)
The bad prognosis initially associated with OS treated exclusively with local therapy (radical surgery) took a turn for the better when systemic chemotherapy was introduced into the treatment schemes in the 1980s. The majority of patients with localized disease develop metastasis within 1 year if they only receive local treatment. However, at the time of diagnosis, the best available methods only detect metastatic disease in 10–20 % of cases [5]. This means that micro metastases exist at the moment of diagnosis in the majority of patients [6]. Currently, treatments based on chemotherapy in conjunction with surgery achieve a 5-year event-free survival rate of 60–70 % in extremity localized, non-metastatic disease. Two major problems are the poor prognosis after metastatic relapse or recurrence, and the poor prognosis for patients with unresectable axial tumors [7].
2.2.1 Surgery
The objective of surgery is to achieve en-bloc resection of the tumor and preserve as much function as possible. Complete surgical resection, if feasible, remains essential for cure. Local control can only be achieved with complete resection margins [8]. In accordance with the definitions of Enneking, complete resection implies the total extirpation of the tumoral tissue (including the biopsy scar) surrounded by an envelope of normal healthy tissue [9]. In the case of a localized OS, as opposed to other sarcomas, achieving surgical remission is important for the overall cure, and so the fundamental objective is to establish adequate surgical margins at the time of local control surgery. In the past, there was controversy over the suitability of conservative surgery as opposed to amputation; currently, with modern treatments for conservation of the extremity, local relapse occurs in 4–10 % of patients, and there do not seem to be clear differences in this respect between ablation surgery and limb salvage surgery [8]. One study found local recurrence rates of 2–3 % after amputation and 5–7 % after conservative surgery, with no significant differences in survival [10]. Amputation is currently reserved for those cases in which it not considered possible to resect the primary tumor; in the great majority of cases it is possible to use surgical techniques that conserve the extremity [11, 12]. During the last few years, the role of conservation surgery has increased markedly. As a result of refinements in neoadjuvant chemotherapy, biomechanical engineering and imaging studies, approximately 80–90 % of patients with OS are now candidates for conservation surgery [13]. Local recurrence has been attributed to insufficient surgical margins, with only a wide margin being considered appropriate [14], although no general agreement exists on what constitutes an adequate margin thickness. Also, surgical margin width in bone has been found not to correlate with the local recurrence rate [15].
At the time of OS diagnosis, about 10–20 % of patients present macroscopic evidence of metastatic disease, most commonly (90 %) in the lungs, but metastases can also develop in bone (8–10 %) and rarely in lymph nodes [13]. The treatment of pulmonary metastases is surgical resection (thoracotomy with metastasectomy) plus effective adjuvant chemotherapy [16].
2.2.2 Radiotherapy
OS is considered a radioresistant tumor [17]. However, radiotherapy can be an adjuvant option as local treatment of unresectable tumors, or as palliation of symptomatic metastases. Conventional external beam radiotherapy with systemic chemotherapy treatment may provide a successful multimodal approach to local control and symptom relief [18]. Proton therapy to deliver high radiotherapy doses has been successfully used as local treatment for some patients with unresectable or incompletely resected OS [19].
2.2.3 Chemotherapy
Before the introduction of adjuvant chemotherapy, more than 80 % of osteosarcoma patients developed metastatic disease. The first studies using individual agents began in the 1960s and 1970s and established, on a non-randomized basis, the role of chemotherapy in the management of osteosarcoma. Prior to the 1960s, OS was considered a chemo resistant tumor. The agents initially investigated yielded inconsistent results. They included l-phenylalanine mustard (with a response rate of 16 %), mitomycin C (response, 24 %), cyclophosphamide (response, 15 %), vincristine, 5-fluorouracil and nitrogen mustard [20]. A regimen comprising mitomycin C, phenylalanine mustard, and vincristine proved fruitless [20]. Responses to individual chemotherapeutic agents, such as, high doses of methotrexate or doxorubicin, were described in 20–40 % of patients with metastatic disease [21].
However, the demonstration of the importance of systemic treatment in the management of osteosarcoma was not established definitively until the publication of the results of a multi-institutional study, which randomized patients with exclusively surgical treatment versus surgery combined with chemotherapy [22].
Current chemotherapy protocols include combinations of the following agents: high dose methotrexate, doxorubicin, cyclophosphamide, cisplatin, ifosfamide, etoposide, and carboplatin, which cure 50–75 % of patients with localized disease. Regimens containing three active chemotherapy agents have been shown to be superior to regimens containing two active agents; regimens with four active agents were not superior to regimens with three active agents; the meta-analysis suggested that three drug regimens that did not include high dose methotrexate were inferior to three drug regimens that did include high dose methotrexate [23–32]. Chemotherapeutic agents such as bleomycin, cyclophosphamide, and dactinomycin (actinomycin D) have been largely abandoned, as they have not proved to be as effective as the other aforementioned drugs [33].
Preoperative, or neoadjuvant, chemotherapy is generally administered for a period of about 8–10 weeks prior to surgery. Following surgical resection and a brief break to allow for wound healing, postoperative adjuvant chemotherapy is continued for a period of another 12–29 weeks [34]. The preoperative chemotherapeutic treatment allows time to plan limb salvage surgery and reconstructive procedures and to study the histological effect of preoperative chemotherapy on the primary tumor so that adjustment of postoperative chemotherapy can be considered [35].
2.2.4 Lessons from the T-10 Protocols
For over two decades, the treatment of OS has followed the basic principles dictated by the T-10 protocol [35]. The T-10 protocol and its variants consist in a chemotherapy regimen with high doses of methotrexate, doxorubicin, cisplatin and a combination of bleomycin, cyclophosphamide and dactinomycin. With the T-10 protocol, the disease-free survival (DFS) at 5 years, as described by its authors, approximates to 70 % [35]. In a study undertaken by the European Osteosarcoma Intergroup (EOI), patients were randomized to receive the T-10 protocol or a simpler protocol, with six cycles of a combination of cisplatin and doxorubicin. The final DFS results were equal in the two branches, which suggests that a simple regimen, with cisplatin and doxorubicin, can cure more than half non-metastatic osteosarcoma patients [36].
One of the greatest contributions of T-10 and its predecessor, T-7, has been the finding that the histological response to the neoadjuvant chemotherapy is the most important prognostic factor in patients with localized disease [37, 38].
Intensification of post-operative or pre-operative therapy with doxorubicin, cisplatin and ifosfamide (IFX) was not found to improve upon T-10 results [38–40]. In the overall setting of intensive multidrug treatment (with high-dose methotrexate [HDMTX], doxorubicin, cisplatin, and ifosfamide), there was no detectable correlation between higher dose intensities and better outcomes [41]. This conclusion is supported by the results of studies by the European Osteosarcoma Intergroup (EOI), the Italian Sarcoma Group (ISG) and the Scandinavian Sarcoma Group (SSG) [7].
2.2.5 Ifosfamide or the Ifosfamide/Etoposide Combination in the Treatment of OS
Ifosfamide (IFX) has been incorporated in osteosarcoma treatment regimens. As a consequence of the first studies, which indicated that IFX as sole agent achieved response rates of 10–60 % in patients with refractory disease, some researchers began to use the drug in the rescue of patients with a poor histological response [43]. Originally, IFX doses of 5–8 g/m2 were used although higher doses (12–18 g/m2) may be more effective [44]. A study by the Pediatric Oncology Group (POG) in patients with previously untreated metastatic disease demonstrated responses in 27 % of cases; the dose used was 12 g/m2 [45]. More recently, IFX has been incorporated as a frontline therapy in the treatment of OS under many regimens [33]. However, the final impact of this incorporation is still not clear [45].
The finding that administration of an alkylating agent with etoposide has a synergistic anti-tumoral effect has led to the combined administration of IFX and etoposide in fractionated form over a period of 3–5 days. In patients with refractory OS and who had previously received IFX, the IFX-etoposide combination achieved response rates of 15–48 % [46]. Recently, for patients with metastatic OS, researchers at the POG incorporated the IFX-etoposide combination in the basic treatment with cisplatin, doxorubicin and high dose methotrexate and achieved responses in 62 % of patients; the DFS at 2 years was 45 % [44]. In patients with metastatic OS, the combination of IFX with etoposide seems to be more effective than that of IFX alone. These results suggest that future studies should investigate this combination in the treatment of patients with localized disease. In particular, the use of the IFX-etoposide combination is being used more frequently for the rescue of patients with a poor histological response; however, there is no evidence that this modification to the treatment improves survival rates. A joint European-American (EURAMOS-1) study found that adding ifosfamide and etoposide to MAP (high-dose methotrexate, cisplatin, and doxorubicin) was associated with additional morbidity and had no effect on survival outcome [47]. Evidence from EURAMOS-1 does not support adaptation of postoperative chemotherapy based on histological response [47].
2.2.6 The Role of Methotrexate in the Treatment of OS
Methotrexate was one of the first drugs demonstrated to be active in OS [6] and has occupied a primordial role in the treatment of OS since then. A meta-analysis [32] of protocols for the treatment of OS concluded that three drug regimens that did not include high dose methotrexate were inferior to three drug regimens that did. Furthermore, certain evidence suggests that the pharmokinetics of methotrexate can influence the final result, although any such influence is inferior and less clear in the context of more-intensive protocols [48, 49]. In any case, the action of methotrexate seems to require that it be administered in high doses (normally 12 g/m2), and administration of lower doses apparently has less impact [50].
The role of HDMTX has not yet been fully clarified [31]. The first EOI study randomized patients to receive six cycles of cisplatin and doxorubicin or four courses of the same combination each preceded by a cycle of HDMTX [36]. In this study, cisplatin-doxorubicin without HDMTX gave better results: DFS at 5 years was 57 % without HDMTX versus 41 % with. A possible reading of these results is that high doses of methotrexate may not be necessary if other agents are intensified. This interpretation may principally be valid when treatment protocols are followed in countries with fewer resources: monitorization of levels is not possible. In Latin America excellent results have been obtained using protocols without methotrexate, protocols that intensify cisplatin, doxorubicin, IFX and etoposide [51]. Daw et al. [31] conducted a multi-institutional trial (OS99) that evaluated the efficacy of carboplatin, ifosfamide, and doxorubicin without HDMTX in patients with newly diagnosed, localized, resectable OS. The regimen used was found to produce outcomes comparable to the outcomes of regimens containing cisplatin or HDMTX. Carboplatin, ifosfamide, and doxorubicin given without HDMTX resulted in 5-year event-free survival (EFS) estimates of 66.7 % and survival estimates of 78.9 %.
2.2.7 Cisplatin and Carboplatin
Cisplatin is one of the most effective agents against osteosarcoma. However, the toxicity of this agent is significant; it can cause loss of hearing and renal failure, both of which can be permanent in some cases. Toxicity can be reduced by using prolonged infusions. The substitution of carboplatin for cisplatin has been recently evaluated at the St. Jude Children’s Research Hospital. In the context of an intense regimen with high doses of methotrexate, IFX and doxorubicin, the use of carboplatin resulted in a DFS at 3 years of 72 %, results comparable to treatments using cisplatin, but with much lower toxicity [45]. However, the incorporation of carboplatin in treatment protocols requires further careful evaluation because other recent studies have demonstrated low anti-tumoral effect when it is used alone in patients with metastatic disease [52]. Additionally, even in the context of a multiple agent regimen, carboplatin is inferior to cisplatin for patients with non-resectable or metastatic osteosarcoma [53].
2.2.8 Pre-/Post-operative Chemotherapy and Intra-arterial Chemotherapy
One of the greatest findings to come out of the T-10 protocol was that the grade of histological response to pre-operative chemotherapy is the most important prognostic factor in patients with localized disease [37]. To date this understanding still holds true, with patients who achieve a good histological response (usually defined as >90 % necrosis) having a better prognosis than those who do not [54, 55]. Studies have consistently demonstrated 5-year EFS rates of 35–45 % for poor responders and 70–80 % for good responders [56]. This observation has determined most current treatments; however, some findings suggest that although intensified chemotherapeutic regimens increased tumor necrosis, the overall survival remained unchanged [57]. Intensification of pre-operative chemotherapy with cisplatin, doxorubicin and IFX gives rise to a modest increase in the proportion of good responders, but the final impact is minimal. Modification of post-operative therapy by intensifying the use of cisplatin, doxorubicin or, more recently, with the incorporation of new agents such as IFX and etoposide have not resulted in significant improvement in the prognosis for patients with poor histological response [25, 40]. Exposure of tumoral cells to sub-optimal cytotoxic levels during pre-operative treatment can give rise to the development of chemo-resistance and increase the propensity to metastatic dissemination. Modification of postoperative therapy cannot reverse such adverse effects. Following this line of reasoning, the achievement of a fast, early response should be the principal objective. Alternatives to intensification of adjuvant chemotherapy could focus, therefore, on improving the pre-operative treatment.
In the 1980s, Jaffe et al. [58] demonstrated that with intra-arterial administration it was possible to achieve high concentrations of cisplatin in a tumor without compromising systemic exposure. Following this, various groups included intra-arterial administration of cisplatin in their treatment of osteosarcoma. Despite the relevance of this development, only two teams endeavored to investigate the matter in a randomized manner. One of these teams, researchers at the Instituto Ortopédico Rizzoli, randomized patients to receive cisplatin intra-arterially or intravenously under the IOR/OS-3 and IOR/OS-5 protocols. Under both protocols, the pre- and post- operative chemotherapy included doxorubicin and high doses of methotrexate, with the addition of IFX in the second protocol. Under both protocols, the proportion of good responders was greater in the group of patients treated with intra-arterial cisplatin, but the final results were similar in both groups [59]. Using a similar design, the other team, a German study, COSS-86, also failed to demonstrate any advantage to intra-arterial administration [60]. In a recent study, however, Wilkins et al. have described excellent results with this technique [61].
2.3 Treatment of Patients with Metastatic Disease
2.3.1 Metastatic Disease at Diagnosis
At the time of OS diagnosis, about 10–20 % of patients present with macroscopic evidence of metastatic disease, most commonly (90 %) in the lungs, but metastases can also develop in bone (8–10 %) and rarely in lymph nodes [62, 63]. However, the remaining 80–90 % of patients are assumed to have micrometastatic disease, which is subclinical or undetectable using current diagnostic equipment. In view of the poor prognosis, treatment of OS patients with observed metastatic disease must include an aggressive, multi-disciplinary approach with intensive pre-operative and post-operative chemotherapy and resection of both the primary tumor and metastases [62, 63]. Using this approach, contemporary protocols that incorporate IFX or the combination of IFX with etoposide, as well as methotrexate, doxorubicin and cisplatin, have been described to attain rates of DFS at 2 or 5 years of 25–45 % [62–64]; a longer follow-up, however, is necessary.
The addition of either muramyl tripeptide or ifosfamide to a standard chemotherapy regimen that included cisplatin, high dose methotrexate, and doxorubicin was evaluated using a factorial design in patients with metastatic osteosarcoma [65]. The appropriate role of muramyl tripeptide in the treatment of osteosarcoma remains under discussion.
Some authors have investigated the use of high-dose chemotherapy and autologous rescue of hematopoietic cells. Whilst this approach is reasonable and practicable, it does not seem to confer any advantage over the conventional treatment [66, 67].
Complete resection of pulmonary metastatic disease can be achieved in a high percentage of patients with residual lung nodules after preoperative chemotherapy. Multiple metastatic nodules imply a worse prognosis than do just one or two nodules, and bilateral lung involvement is worse than unilateral. Patients with peripheral lung lesions may have a better prognosis than patients with central lesions. Patients with fewer than three nodules confined to one lung have been found to have 5-year EFS of approximately 40–50 % [68].
After the lung, the second most common site of metastasis is another bone. The prognosis is poor for patients with metastasis to other bones distant from the primary tumor or with transarticular skip lesions [69, 70].
Multifocal OS is a different entity, and patients have an extremely poor prognosis. No patient with synchronous multifocal OS has ever been reported to be cured, but systemic chemotherapy and aggressive surgical resection may achieve significant prolongation of life [71].
2.3.2 Recurrent OS
Approximately 50 % of relapses occur within 18 months of therapy termination, and only 5 % of recurrences develop after five or more years [72]. The incidence of recurrence by site has been estimated as follows: lung (65–80 %), bone (8–10 %), local (4–7 %), and combined relapse (10–15 %) [72]. Other localizations of metastases are rare but may occur as late as 4 years after diagnosis [73].
For patients with OS in relapse, a very aggressive surgical approach is recommended. The survival rate at 4 years after relapse, for patients in whom it is possible to obtain a complete new remission, is approximately 30–40 %; it is 0 % for patients in whom the disease cannot be resected [74]. The role of systemic chemotherapy for the treatment of patients with recurrent osteosarcoma is not well defined. The choice of systemic treatment depends on the previous primary treatment. Ifosfamide (in combination with etoposide) has proved active in as many as one third of recurrent OS patients who had not previously received ifosfamide [75]. Cyclophosphamide and etoposide are active in recurrent OS, as is the combination of gemcitabine and docetaxel [76, 77]. Grignani et al. [78] reported rare objective responses and disease stabilization with sorafenib in patients with recurrent OS.
The prognostic factors for survival after relapse include the presence of isolated pulmonary metastasis: patients with metastases limited to the lungs have a better outcome than do patients with metastases to other sites or to the lungs combined with other sites [79]; late relapse (>24 months); and a low number of pulmonary lesions [16]. Bielack et al. [80] reported survival estimates with second and subsequent OS recurrences. Five-year overall survival (OAS) and EFS rates were 16 % and 9 % for second, 14 % and 0 % for third, 13 % and 6 % for fourth, and 18 % and 0 % for fifth recurrences, respectively [80]. The median interval from first to second recurrence was 9 months, and the median interval between subsequent recurrences was approximately 6 months [80].
2.3.3 Future Treatments
The survival rates for patients with metastatic disease at the time of diagnosis and for those in relapse are very low: less than 30 % survive [80]. For this reason it is necessary to develop new strategies, for example, strategies based on new drugs or new combinations of existing drugs, to treat patients with poor prognosis. The fractionated administration of cyclophosphamide with the inhibitor of topoisomerase, topotecan, seems to be a promising combination for the treatment of many pediatric neoplasias. However, in relapsed osteosarcoma patients, the rate of response was only 11 %, a response far lower than that observed in other neoplasias [81]. Sequential administration of gemcitabine and docetaxel has been demonstrated to be quite effective in the treatment of sarcomas in relapse [77]. In a study of a group of 35 adult patients with various relapsed sarcomas, the response rate was 43 %; the study included four patients with refractory osteosarcoma, of which two gave partial responses and two attained stable disease states [82].
Another agent that proved to have good activity in vitro against osteosarcoma is ecteinascidin-743 (ET-743). However, in spite of the promising results of preclinical studies, a phase II study in patients with recurrent osteosarcoma did not demonstrate any activity [83].
The majority of patients who relapse do so with lung metastases. In fact, most patients are considered to have micro metastatic lung disease at the moment of diagnosis. The possibility of controlling this microscopic lung disease on finalizing treatment for the primary tumor could give rise to a substantial improvement in survival. In an animal model, the administration of muramyl tripeptide encapsulated in liposomes (L-MTP-PE) activated pulmonary macrophages and brought about the eradication of lung micro metastases [84]. The Children’s Oncology Group (COG) performed a prospective randomized trial in newly diagnosed children and young adults with localized OS. All patients received cisplatin, doxorubicin, and high dose methotrexate. One half of the patients were randomly assigned to receive ifosfamide. In a second randomization, one half of the patients were assigned to receive L-MTP-PE beginning after definitive surgical resection. The addition of ifosfamide did not improve outcome. The group that received L-MTP-PE had a longer EFS, but the improvement did not meet the conventional standard for statistical significance (P = 0.08). The group did, however, show a significant improvement in OS (78 % vs. 70 %; P = 0.03) [85]. The appropriate role of L-MTP-PE in the treatment of osteosarcoma remains under discussion.
It is possible that one might effect an activation of alveolar macrophages with nebulized GM-CSF. In a phase I study carried out in patients with lung metastasis of various refractory neoplasias, nebulized GM-CSF was successfully administered with minimal toxicity. The dose tolerated was 250 mcg/dose, twice a day for seven consecutive days every other week. Only two patients with OS were treated in the study, and in one of them the disease was stabilized for over 11 months [86].
Another interesting approach, which has been developed in an animal model, is gene transfer of IL-12 by nebularization using a non-viral vector, such as the cationic DNA transporter polyethylenimine (PEI). Therapy with IL-12 is based on the known anti-tumoral activity of this cytokine. However, the clinical use of IL-12 in a systemic way is limited by its toxicity. Using the technology of PEI-based IL-12 transfer in immunosuppressed mice with OS lung metastasis, researchers demonstrated an increase in the expression of IL-12 in lung tissue, accompanied by an increase in tissue levels of IL-12. It is important to note that an increased expression of IL-12 in the liver was not observed, which suggests that the systemic effects were minimal. In this animal model, the aerosol therapy brought about a significant decrease in the number of metastatic nodules. Also, it has been demonstrated that IL-12 increases, in vitro, the sensitivity of osteosarcoma to alkylating agents. This occurs through a mechanism involving activation of the Fas pathway. The implication is that IL-12, by aerosol, could have a synergistic effect with IFX. The same PEI transfer technique has been used for transfection of p53, which demonstrated anti-tumoral activity [87].
Aerosolization technology has extended to the administration of chemotherapeutic agents directly to the lungs. For example, 9-nitrocamptothecin was nebulized into either mice with subcutaneous xenoinserts of a variety of neoplasias or mice with osteosarcoma lung lesions. There was good anti-tumoral effect on both the subcutaneous and the lung tumors, which suggests that there is not only a local effect but also a systemic one. Based on these preliminary studies, a phase I study in patients with lung metastasis from refractory neoplasias has recently been completed; phase II studies are being developed [88].
The degree of necrosis observed in the primary tumor after an initial period of chemotherapy correlates with subsequent EFS and OS. An international consortium (EURAMUS) was formed to conduct a large prospective randomized trial. All patients received initial therapy with cisplatin, doxorubicin, and high dose methotrexate. Patients with more than 90 % necrosis were randomly assigned to continue the same chemotherapy after surgery or to receive the same chemotherapy with the addition of interferon. The addition of interferon did not improve the probability of EFS [89]. Patients with less than 90 % necrosis were randomly assigned to continue the same chemotherapy or to receive the same chemotherapy with the addition of high dose ifosfamide and etoposide.
Reports on the use of high dose samarium-153-EDTMP radioisotope therapy as a method to provide palliation for patients with bone metastases indicate feasibility, but so far the role of this treatment modality is not well defined [90]. In addition, high doses of samarium-153-EDTMP are being used increasingly as a therapeutic agent.
2.4 Treatment of Ewing’s Sarcoma (ES)
In his initial description of ES as a diffuse endothelioma of the bone, James Ewing pointed out, among other characteristics of this tumor, that it has an elevated susceptibility to radiation therapy [91]. Over the following 50 years, radiotherapy continued to be the predominant way of treating ES, although 80 % of patients died after local relapse or metastatic disease during the 2 years after diagnosis.
The current therapeutic approach, which gives cause for much greater optimism in terms of cure rates, seeks to cure the patient while preserving the functionality of the affected body part and to minimize late secondary effects.
The approach comprehends a multi-disciplinary focus based on the following concepts:
1.
ES has a systemic character at the time of diagnosis. The therapeutic approach requires adequate local control of the macroscopic disease, together with systemic control of micrometastasis.
2.
Local control of the macroscopic disease must be multi-disciplinary: combining, according to necessity, surgery, radiotherapy and chemotherapy. The response to neoadjuvant chemotherapy and the surgical possibilities determine the doses and fields of radiotherapy. Local control measures must not compromise systemic control.
3.
Systemic control of micrometastasis must be carried out through the administration of a protocol of intensive cyclic polychemotherapy comprising agents of the greatest anti-tumoral activity available.
4.
Treatment must be adapted to the characteristics of each patient, taking into account the primary localization, the size of the lesion, the staging and therapeutic possibilities according to age. This tailoring is necessary to obtain the maximum therapeutic benefit with the best possible functional result.
2.4.1 Chemotherapy for ES
Current protocols of chemotherapy for ES include vincristine, doxorubicin, ifosfamide/cyclophosphamide, and etoposide [92–94]. The mode of administration and dose intensity of chemotherapy within courses differs markedly between protocols. A European Intergroup Cooperative Ewing Sarcoma Study (EICESS) trial suggested that 1.2 g of cyclophosphamide produced a similar event free survival (EFS) as 6 g of ifosfamide in patients with lower risk disease. The same study also identified a trend toward better EFS for patients with localized ES, and a tendency for patients with higher-risk disease to receive a treatment that included etoposide [95]. Protocols in the USA generally alternate courses of vincristine, cyclophosphamide, and doxorubicin with courses of ifosfamide/etoposide [96], while European protocols generally combine vincristine, doxorubicin, and an alkylating agent with or without etoposide in a single treatment cycle [97].
Standard chemotherapy in current protocols includes vincristine, doxorubicin, and cyclophosphamide, also known as the VAdriaC or VDC regimen, alternating with ifosfamide and etoposide (IE) [98]. The combination of IE has shown activity in Ewing sarcoma, and a large randomized clinical trial and a nonrandomized trial demonstrated that outcome was improved when IE was alternated with VAdriaC [92–94]. Dactinomycin is no longer used in the United States but continues to be used in EuroEwing studies. In a meta-analysis of studies that had been undertaken before the standard use of ifosfamide and etoposide, increased dose intensity of doxorubicin during the initial months of therapy was associated with an improved outcome [99]. A 4-year EFS of 82 % has been reported for high dose VAdriaC and IE [100]. However, in an intergroup trial of the Pediatric Oncology Group and the Children’s Cancer Group, which compared a dose-intensified chemotherapy regimen of vincristine, doxorubicin, cyclophosphamide, ifosfamide, and etoposide (VDC/IE) with standard doses of the same regimen, no differences in outcome were observed [101]. In a COG trial, patients were randomly assigned to receive chemotherapy (VAdriaC alternating with IE) given every 2 weeks (interval compression) versus every 3 weeks (standard). Patients treated every 2 weeks had an improved 5-year EFS relative to those treated every 3 weeks (73 % vs. 65 %, P = .048). There was no increase in toxicity observed with the every-2-week schedule [97]. The duration of primary chemotherapy ranges from 6 months to approximately 1 year.
For patients with a high risk of relapse with conventional treatments, certain researchers have utilized high-dose chemotherapy with hematopoietic stem cell transplant (HSCT) as consolidation treatment, in an effort to improve outcome [102–113]. A retrospective review using international bone marrow transplant registries compared, for patients with Ewing sarcoma at high risk for relapse, reduced intensity conditioning with high intensity conditioning followed by allogeneic stem cell transplant; outcomes were not found to differ [114]. Multiple small studies that report benefits achieved with HSCT have been published. However, these results are difficult to interpret because only patients who have a good initial response to standard chemotherapy are considered for HSCT [110].
2.4.2 Treatment Response Factors to Preoperative Therapy
Multiple studies have shown that patients with minimal or no residual viable tumor after presurgical chemotherapy have significantly better event free survival than patients with larger amounts of remaining viable tumor [115, 116]. For patients who receive preinduction and postinduction chemotherapy positron emission tomography (PET) scans, decreased FDG uptake after chemotherapy correlated with good histologic response and better outcome [117]. Patients with poor response to presurgical chemotherapy have a higher risk of local recurrence [118].
2.4.3 Local Control of Disease
Analysis of local control in ES is complicated because of the difficulties involved in the interpretation of the radiological studies typically used in follow-up, after administration of combined treatments. On many occasions, after surgery, radiotherapy and chemotherapy, the imaging signal obtained is not that of normal bone and is difficult to interpret. Persistence of residual material corresponding to fibrosis, necrosis or both is frequent. In this respect, studies with magnetic resonance after administration of gadolinium are more reliable than other imaging techniques.
The analysis of results in terms of local control referred to in the various reported series, which are generally lacking in biopsy/necropsy studies, can under- or over- estimate the degree of control. Fernández et al. [119] registered a rate of clinical local relapse of 37.5 %, which after necroscopic studies increased to 47.5 %. In other studies, even larger differences have been detected: for example, a rate of local relapse being 65 % while clinical diagnoses suggested a rate of scarcely 25 % [120].
It is important to note that local control, while an indispensable condition for obtaining a full cure, can, however, accompany a prognosis of death. In the pre-chemotherapy era, rates of local control of 60–70 % were reported for series in which the rates of survival were below 20 % [121].
2.4.4 Therapeutic Strategy to Obtain Local Control
2.4.4.1 Radiotherapy
While surgery is effective and appropriate for patients who can undergo complete resection with acceptable morbidity, children who have unresectable tumors or who would suffer loss of function are treated with radiation therapy alone. Those who undergo gross resections with microscopic residual disease may benefit from adjuvant radiation therapy. Randomized trials that directly compare both modalities (i.e. resection with or without radiation therapy) do not exist, and so the relative roles of gross resection and radiotherapy remain controversial. Research addressing this issue are retrospective and nonrandomized, limiting their value. Krasin et al. [122] reported on a series of patients with localized ES who received both surgery and radiation. Local failure for patients with positive and negative margins was 17 % and 5 %, respectively, and overall survival (OS) was 71 % and 94 %, respectively. However, in a large retrospective study, 45 Gy adjuvant radiation therapy for patients with inadequate margins did not appear to improve either local control or disease free survival [123]. It is not known whether higher doses of radiation therapy could improve outcome. Adjuvant radiation therapy should be considered for patients with residual microscopic disease, inadequate margins, or viable tumor in the resected specimen and close margins. When preoperative assessment has suggested a high probability that surgical margins will be close or positive, preoperative radiation therapy has been used to shrink the tumor, thereby enabling surgical resection with clear margins [124].
Data for patients with pelvic primary ES from a North American intergroup trial showed no difference in local control or survival based on local control modality: surgery alone, radiation therapy alone, or radiation plus surgery [125]. For young children with ES, surgery may be a less morbid therapy than radiation therapy because radiation causes retardation of bone growth. Another potential advantage of surgical resection of the primary tumor is the information it can provide concerning the amount of necrosis in the resected tumor. Patients with residual viable tumor in the resected specimen have a worse outcome than those with complete necrosis. In a French Ewing study (EW88), EFS for patients with less than 5 % viable tumor, 5 % to 30 % viable tumor, and more than 30 % viable tumor was 75 %, 48 %, and 20 %, respectively [116].
ES shows great sensitivity to radiation [126]. The local control obtainable with irradiation is directly proportional to the dose administered and to the volume of tissue included in the field. The percentage of patients for whom radiotherapy alone achieves local control is between 50 and 80 %. With doses above 40 Gy, high percentages of local control are obtained. With lower doses, even when rapid clinical improvement and disappearance of macroscopic lesions are observed, the incidence of local relapse is very high [122].
For patients with residual disease after an attempt at surgical resection, the Intergroup Ewing Sarcoma Study recommends 45 Gy to the original disease site plus a 10.8 Gy boost for patients with gross residual disease and 45 Gy plus a 5.4 Gy boost for patients with microscopic residual disease. Radiation therapy is not recommended for those who have no evidence of microscopic residual disease following surgical resection. Radiation therapy is associated with the development of secondary malignant neoplasms. A retrospective study noted that the incidence of a secondary malignancy was 20 % for patients who had received 60 Gy or more and 5 % for those who received 48–60 Gy; none of those who received less than 48 Gy developed a second malignancy [127].
Hyperfractionated radiation therapy has not been associated with improved local control or decreased morbidity. A comparison of proton beam radiation therapy and intensity modulated radiation therapy (IMRT) has shown that proton beam radiation therapy can spare more normal tissue adjacent to the primary Ewing sarcoma than IMRT [128]. The study’s follow-up was relatively short, and there are no data available to indicate whether the reduction in dose to adjacent tissue will result in improved functional outcome or reduce the risk of secondary malignancy.
2.4.4.2 Chemotherapy Plus Radiotherapy
The addition of chemotherapy protocols to local irradiation have not only meant an advance in the control of systemic disease but have also increased the rates of local disease control. Numerous authors have demonstrated the beneficial effect of this therapeutic association, in comparison with historical series. Consequently, over the last 20 years, this therapeutic approach: the combination of high-dose irradiation with systemic chemotherapy has been considered as optimum in the treatment of ES [129].
2.4.4.3 Chemotherapy Plus Surgery
With the combination of neoadjuvant chemotherapy plus surgery, local control rates similar to those obtained with radiotherapy (alone) have been reported [133]. However, it is difficult to compare the two approaches because no randomized comparative study has ever been undertaken. The data of CESS-86 are the closest approximation to a comparative study; differences between both groups were not found (DFS at 5 years was 67 % with chemotherapy versus 65 % with radiotherapy) [134]. Scrutiny of published comparative works indicates a clear selection of patients. The tendency has been to use surgery (with chemotherapy) for peripheral tumors or for localized tumors of reduced dimensions, which have a better prognosis than central tumors of greater volume [115]. In general there seems to be agreement in the idea of avoiding radiation in patients whose young age leads one to predict serious sequelae.
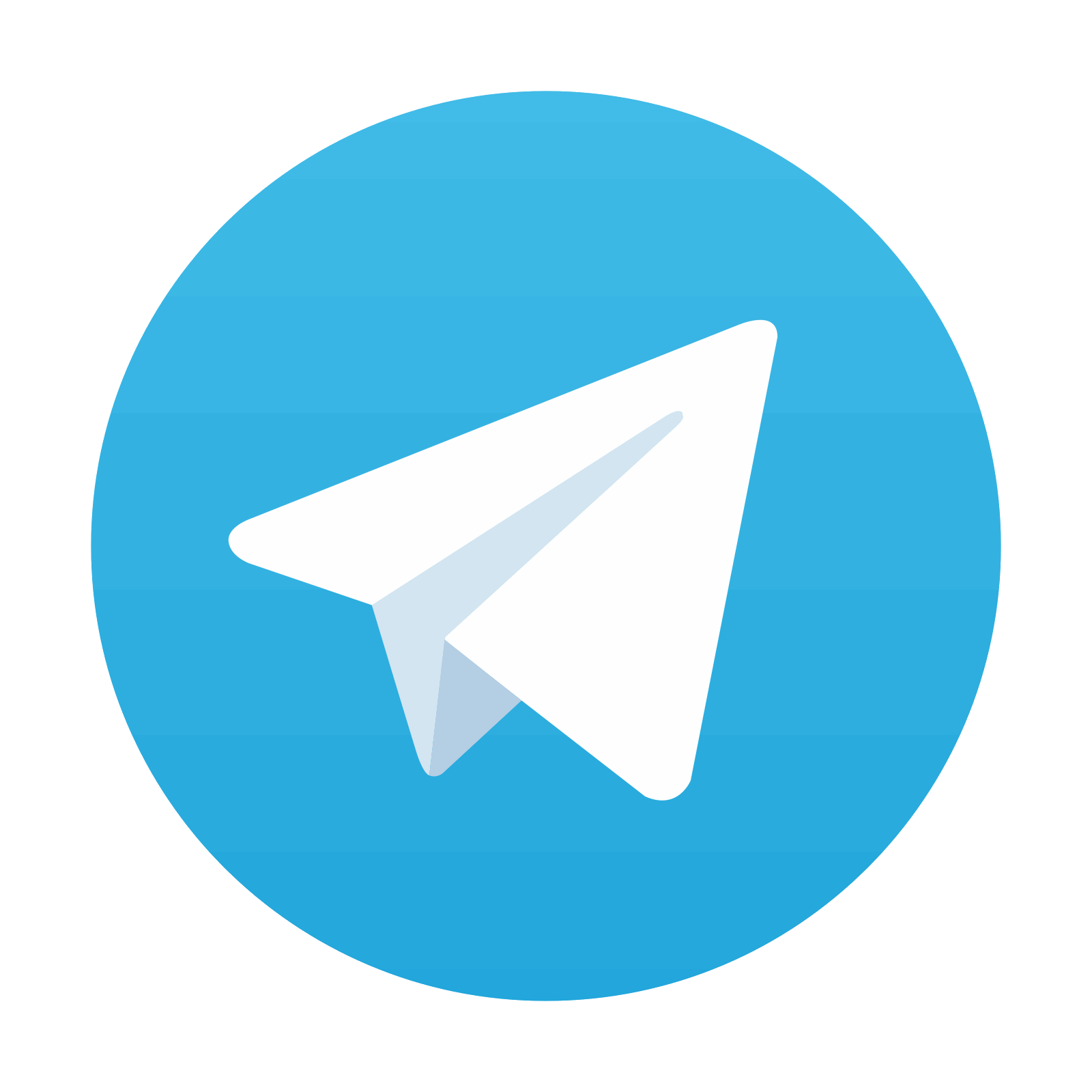
Stay updated, free articles. Join our Telegram channel

Full access? Get Clinical Tree
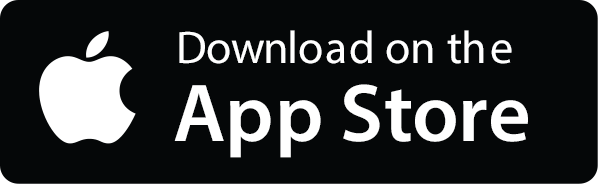
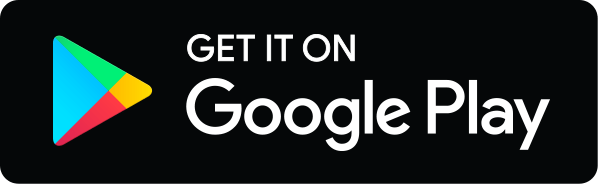