Understanding the series of operations that a child with single ventricle physiology may undergo can be daunting. Amidst the complex terminologies that accompany these procedures, the neurologist and intensivist should pay attention to how cerebral venous drainage is achieved, whether and where mixing of oxygenated and deoxygenated blood takes place, and what risk factors for thrombotic or hemorrhagic complications are present.
A summary of the classic three-staged surgical palliation for hypoplastic left heart syndrome (HLHS), a prototypical single ventricle CHD lesion, is provided in Table 23.2. With the first stage, a child’s venous drainage is accepted into the actively pumping and relaxing ventricle, as with a normal child. With the second and third stages, venous drainage becomes a passive flow phenomenon wherein vena caval blood must empty directly into the pulmonary arteries. The resulting central venous pressure may be quite high, leading to frequent headaches and an increased risk of cerebral venous sinus thrombosis. Stasis of flow may also lead to thrombus formation in the cavopulmonary pathway itself. The acute increase in central venous pressure that results from the obligate passive venous return from the systemic vena cavae to the pulmonary vasculature may lead to pleural effusions. Procoagulant and anticoagulant factors may be lost through a draining pleural effusion or via protein losing enteropathy. This, along with the loss of overall body fluid, may place the child after stage 2 or stage 3 palliative surgery at higher risk of thrombotic and thromboembolic complications. Careful monitoring of hydration status and serum levels of factors such as antithrombin III are warranted, and management with hydration and fresh frozen plasma administration may be indicated.
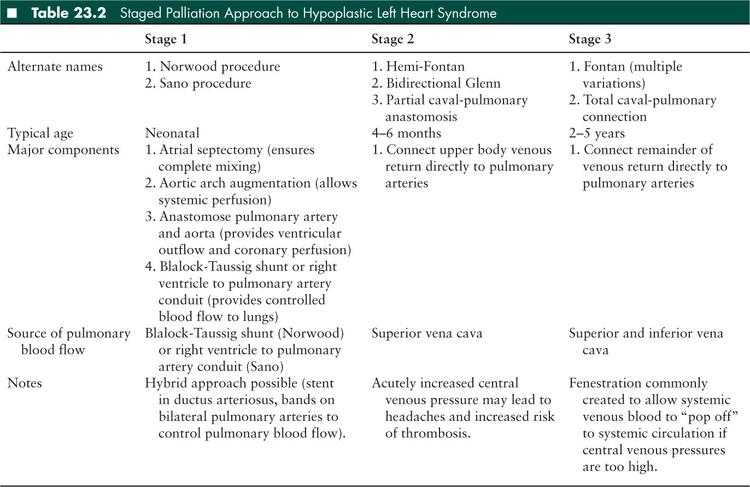
The first two stages of single ventricle palliation require mixing of the venous and systemic circulations in the heart, which means that venous blood may flow to the brain without the pulmonary bed as an intermediary filter. In the third stage, most venous blood flows through the pulmonary bed, but a communication called a fenestration may be created between the pulmonary and systemic circulations, allowing right-to-left shunting of venous blood to the arterial circulation. Such fenestrations are commonly created to aid in postoperative recovery by preserving cardiac output in the face of acutely elevated central venous pressure. Many Fontan fenestrations endothelialize and close spontaneously, although those that do not can usually be closed later by percutaneous device in the cardiac catheterization laboratory.
Children with biventricular heart disease may warrant special neurologic attention as well. Those with ventricular dysfunction will be discussed further in the section on heart failure. Shunt lesions including septal defects and patent ductus arteriosus (see Table 23.1) can provide a conduit from the venous to the systemic circulation. Prosthetic material on either side of the circulation can serve as a nidus for infection (e.g., endocarditis) or thrombus formation. Current American Heart Association guidelines recommend antibiotic prophylaxis for bacterial endocarditis at times of increased risk for patients with prosthetic heart valves, with a history of infectious endocarditis, with unrepaired cyanotic CHD, in the first 6 months after completing a CHD repair using prosthetic material or device, with repaired CHD and a residual defect near prosthetic material, and in cardiac transplant recipients with valvulopathy (2). Anticoagulation is recommended for those with a mechanical prosthetic valve replacement, with a goal international normalized ratio (INR) of 2.5 (range 2.0–3.0) for those placed in the semilunar valve position (as with bioprosthetic valves in any position), and a goal INR of 3.0 (range 2.5–3.5) for those placed in the tricuspid or mitral valve positions (3).
■ PERIOPERATIVE CONSIDERATIONS
Surgical correction or palliation is often required to support infants and children with CHD. Given the variety of heart defects, surgical procedures are varied. The timing of intervention is linked closely to the severity of the heart lesion. A detailed discussion on the risks associated with each surgical intervention is beyond the scope of this chapter. Typical surgical risks involving anesthetics, mechanical ventilation, bleeding, or infections are present. Some perisurgical neurologic morbidity is specific to the type of heart defect or postoperative management, and not simply the conduct of the surgery itself. The following discussion will focus on specific risks related to the care of infants and children with CHD. The management of other complications such as arterial ischemic stroke, intracranial hemorrhage, and cerebral venous sinus thrombosis are discussed elsewhere in this text.
■ WHITE MATTER INJURY
Infant heart surgery refers to surgical procedures performed on children less than 30 days old with heart defects so severe that survival depends on surgical correction or palliation. For such patients, the mortality from these early surgeries has dropped dramatically since the late 1980s. In the more experienced centers with the highest surgical volumes, survival approaches 90% for the most complex and technically challenging surgeries (4). With this increase in survival, the focus of care has shifted to neurologic morbidity.
It has long been observed that the head circumferences in infants with severe CHD are smaller than infants of similar gestation ages without CHD (5,6). Infants with CHD are also prone to hypoxic-ischemic white matter injury that is not distinguishable from periventricular leukomalacia (PVL), an injury seen primarily in premature infants (Figure 23.1) (7,8). In infants with multiple forms of CHD, this injury is seen in 17% to 40% before surgery (9–11) and in over 50% after surgery (12,13). Research has now demonstrated that the primary risk for this injury is a brain-specific intrauterine growth retardation that arises as a consequence of the alterations in fetal hemodynamics created by the CHD (5,6,12). Fetal circulation in children with CHD has recently been reviewed (14).
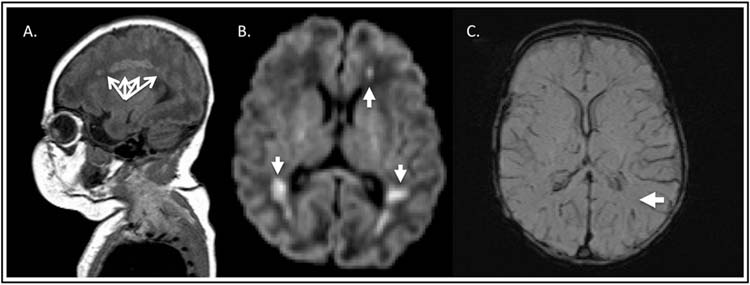
FIGURE 23.1 Magnetic resonance imaging of white matter injury (periventricular leukomalacia). (A), Sagittal plane, T1 hyperintensity in the white matter watershed (radial arrows). (B), Axial plane, diffusion weighted imaging—areas of periventricular restriction of water diffusion (arrows). (C), Axial plane, susceptibility weighted imaging (SWI) demonstrating the absence of hemorrhage within lesions (single arrow).
Brain magnetic resonance imaging (MRI) is required to diagnose PVL because the lesions are typically of a volume that is below the resolution threshold for head computed tomography (HCT) or head ultrasound imaging. The typical PVL lesion occurs in the white matter watershed zone, is hyperintense on T1 imaging (Figure 23.1A), may demonstrate restriction of water motion if acute (Figure 23.1B), and may have susceptibility signal on T2* (Figure 23.1C) that reflects either hemorrhage or mineralization. The lesions may demonstrate restriction of water motion if acute (Figure 23.1B). The limitation of diffusion-weighted imaging is that pseudonormalization may occur as early as 7 days after injury. MRI slice thickness is also important since interslice gaps may cause obscuration of lesions. There is controversy in nomenclature of these white matter lesions. Some centers refer to white matter lesions with restriction of water motion on diffusion-weighted imaging as arterial ischemic stroke (see later discussion) while other centers consider these lesions part of the spectrum of PVL.
The risk for preoperative and postoperative injury is different for individual cardiac diagnoses. Higher preoperative risk is seen in transposition of the great arteries (TGA), and a higher rate of postoperative injury is seen in HLHS. Recent investigations (unpublished data from D.L. Licht) suggest that low arterial oxygen content coupled with increasing cerebral oxygen metabolic demand after either birth or cardiopulmonary bypass create the conditions that lead to the white matter injury in the form of PVL. For patients with TGA, the lowest oxygen saturations occur prior to surgery and should normalize after corrective surgery. Therefore proceeding to surgical correction at the earliest time possible as suggested by Petit and colleagues (11) should be protective against PVL. Conversely, for patients with HLHS or other single ventricle heart lesions, systemic oxygen saturations are typically in the mid 70% to 80% range before surgery and are not significantly higher after initial surgical palliation. Cerebral oxygen metabolism for these infants however, is greatest in the first 24 hours during the recovery from the moderate (∼25°C) or deep (∼18°C) hypothermia that is required for safe conduct of these intricate surgeries with cardiopulmonary bypass. Thus, the risk for PVL in HLHS and other infants with single ventricle physiology is increased after surgery (up to 75% risk) (12,13). Strategies to either reduce metabolic demand or increase oxygen delivery may thus be particularly useful in these populations.
In patients with HLHS and similar single ventricle lesions, the cerebral-systemic circulation is in series with the pulmonary circulation since all cardiac output is through the common pulmonary artery. Maintaining a balance of pulmonary to systemic blood flow ensures optimal systemic oxygen delivery. However, maintaining this balanced circulation is often difficult because the perinatal pulmonary vascular resistance drops after birth. Rising peripheral oxygen saturations in an HLHS patient may be a sign of pulmonary overcirculation and should thus prompt careful assessment of systemic perfusion. As a higher fraction of cardiac output flows to the lungs, organ ischemia may ensue with lactic academia and circulatory collapse. Early surgery may be warranted for those HLHS patients with peripheral oxygen saturations in the 90% range to prevent ischemic damage to other organs. Likewise, in the postoperative single ventricle patient after stage 1 palliation, permissive hypercarbia with slight respiratory acidosis is preferable to a respiratory alkalosis with overventilation to prevent pulmonary overcirculation through the systemic-to-pulmonary artery shunt.
■ ARTERIAL ISCHEMIC STROKE
The risk for arterial ischemic stroke in the perisurgical care of the infant with severe CHD is much debated, and controversy exists on how brain injury is defined in this setting. Classically, arterial ischemic stroke is defined by focal neurologic deficits that are referable to an arterial territory of the brain. In children, this definition has been expanded to include confirmatory neuroimaging. In infants, arterial ischemic stroke may be asymptomatic; thus the definition can be purely based on neuroimaging. McQuillen and colleagues (15) reported a very high incidence (30%) of preoperative arterial ischemic stroke in infants with TGA. Their group showed a higher risk of arterial ischemic stroke in those who underwent balloon atrial septostomy, a catheter-based procedure performed to rapidly improve arterial blood saturations by enhancing intracardiac mixing of oxygenated and deoxygenated blood. Two later publications by Petit and colleagues (11) and Beca and colleagues (9) both reported very low arterial ischemic stroke rates (0% and 4.5%, respectively) in patients with TGA. Petit and colleagues further argued that the performance of balloon atrial septostomy was necessary to protect infants with TGA from PVL and was not a risk for arterial ischemic stroke. It is possible that differences in lesion nomenclature (PVL versus arterial ischemic stroke) at different centers have led to the discrepancies in stroke incidence.
There is sparse literature on stroke in infants with severe CHD requiring infant heart surgery. In a retrospective study, Chen and colleagues (16) identified stroke in 12 of 122 infants (10%). Of these 12, 6 had arterial ischemic stroke and 6 had watershed stroke. This low prevalence rate is congruent with the rate of stroke seen by Beca and colleagues (9) and with our own experience of 2% to 4% preoperative and 8.6% postoperative, in mixed populations of infants with severe CHD (Goff et al. manuscript in preparation). With such small numbers, investigation of risk factors is not likely to be fruitful. Centrally placed catheters, such as umbilical catheters, right atrial catheters, and femoral catheters are potential thrombus sources.
Older children with single ventricle heart lesions are highly predisposed to arterial ischemic stroke due to their unique anatomy. Lower extremity access should be avoided if possible in patients with bidirectional Glenn physiology (stage 2) because the inferior vena cava remains in direct communication with the systemic circulation. Fenestrations placed surgically at the time of Fontan completion act as a pressure pop-off valve, but are obligate right-to-left shunts that may also allow for paradoxical emboli. Chylous effusions and protein losing enteropathy from high central venous pressures can result in a prothrombotic state due to protein losses. Adequate hydration and factor replacement with fresh frozen plasma may be required.
■ INTRACRANIAL HEMORRHAGE
Detection of intracranial hemorrhage prior to surgery is of obvious importance because the conduct of heart surgery with cardiopulmonary bypass requires aggressive anticoagulation. The term intracranial hemorrhage includes epidural, subdural, subarachnoid, intraventricular, and intraparenchymal hemorrhages. In contrast, the term intracerebral hemorrhage encompasses only intraparenchymal hemorrhage and intraventricular hemorrhage. At the time of birth, some amount of intracranial hemorrhage has been found to be very common (17). These include subdural hemorrhages along the tentorial membrane and choroid plexus hemorrhages, which may have intraventricular extension (Figure 23.2A). It is important to note that these hemorrhages do not usually extend during the conduct of infant heart surgery with cardiopulmonary bypass despite the requirement for systemic anticoagulation during cardiopulmonary bypass.
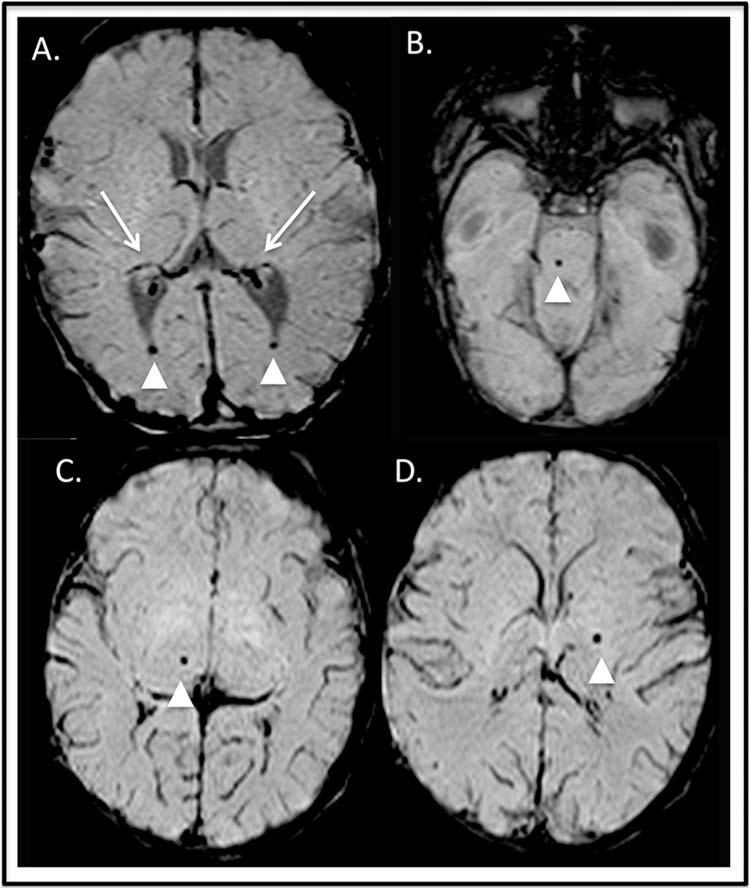
FIGURE 23.2 Intracerebral hemorrhage. Axial susceptibility weighted imaging. (A) Preoperative hemorrhage: bilateral choroid plexus hemorrhage (long arrows) with intraventricular extension (arrowheads). Postoperative punctate hemorrhages in the pons (B, arrowhead), right thalamus (C, arrowhead), and left globus pallidum (D, arrowhead).
Intraparenchymal hemorrhages found on preoperative head ultrasound, or incidentally as part of a research protocol, must be managed on a case-by-case basis since there is a paucity of information on the risk of hemorrhagic extension on cardiopulmonary bypass. If an incidental intracranial hemorrhage is found that is not a subdural hemorrhage along the tentorial membrane or choroid plexus hemorrhage, our practice is to postpone surgery when possible by 1 week. The patient is reevaluated with a follow-up, preoperative brain MRI before proceeding.
Subdural hemorrhages are the most common clinically significant hemorrhages in the postsurgical patient, and these can occur acutely or subacutely after surgery. Anticoagulation, elevation in central venous pressures, and brain atrophy (from other injury) are all putative contributors to increased risk. Subdural hemorrhages requiring surgical intervention are uncommon in the cardiac ICU population.
Punctate microhemorrhages seen on brain MRI (Figure 23.2B, C, and D) after heart surgery with cardiopulmonary bypass or after catheter-based interventional procedures are of unclear clinical significance. These microhemorrhages may be the result of microthromboembolic material from bypass cannulation. The lesions are common, occurring in 30% to 50% of postsurgical patients. There is a single report that these lesions may contribute to the neurodevelopmental impairment that is seen in survivors of infant heart surgery (18).
■ CEREBRAL VENOUS SINUS THROMBOSIS
Cerebral venous sinus thrombosis has been described in the CHD population, particularly in children or infants with dehydration and/or protein loss through pleural effusions or protein losing enteropathy. Management is described in Chapter 11, but in general includes hydration, elevation of the head of bed when possible to promote venous drainage, and systemic anticoagulation.
■ HEART FAILURE
Heart failure is the syndrome that results from the inability of the heart to meet the body’s metabolic demands. Heart failure may be primary (due to intrinsic cardiomyopathy) or secondary to an underlying condition like valvulopathy (Table 23.3). A child in heart failure is at increased risk of neurologic complications due to several factors. First, a low cardiac output places a child at risk for decreased cerebral perfusion, a situation that can lead to watershed infarctions, hypoxic-ischemic brain injury, and secondary seizures or encephalopathy. Second, if the child’s symptoms are due to decreased ventricular systolic function, the resulting stasis of blood may increase the child’s risk of thromboembolic events. Third, arrhythmias may occur and may increase the risk of thromboembolism and thus arterial ischemic stroke. Some of those arrhythmias are secondary to the condition which has also caused heart failure, such as mitral valve stenosis and secondary atrial flutter. Some arrhythmias can result in complete circulatory collapse. This sudden complete cessation of circulation can result in hypoxic-ischemic injury to the deep gray nuclei, motor cortex, visual cortex, or other areas of the brain.
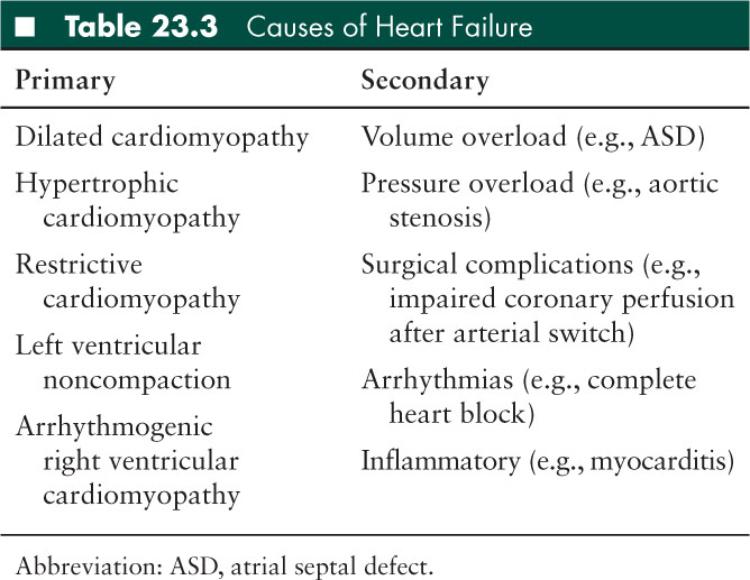
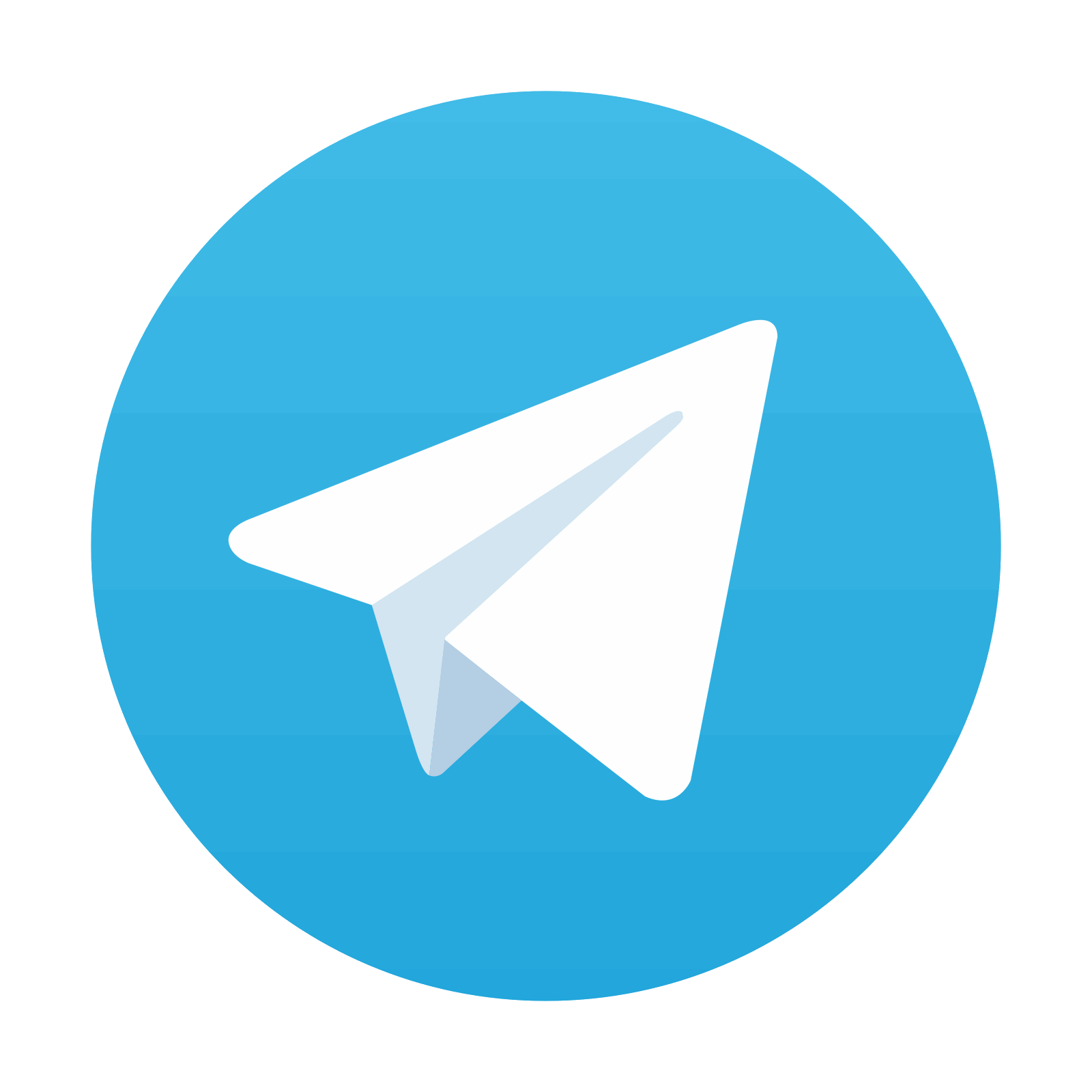
Stay updated, free articles. Join our Telegram channel

Full access? Get Clinical Tree
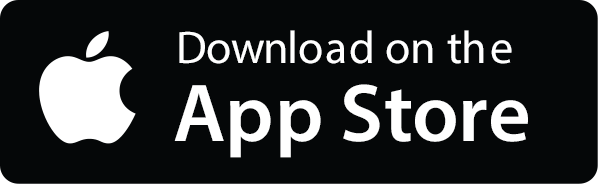
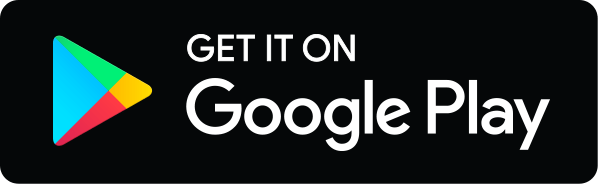