CHAPTER 2 Nature, Nurture, and Their Interactions in Child Development and Behavior Paul Wang, MD, FAAP Nature and nurture have long been regarded as rival influences on child development and behavior. One school of thought has contended that a child’s behaviors and developmental outcome are determined by nature—that is, by innate biology—while a rival school has argued that nurture—a child’s environment and experiences—is dominant in determining the child’s developmental outcome. While the nature-nurture debate raged among academics, intuitive parents and primary pediatric health care professionals have long known that both sets of factors—the innate and the experiential—are important in the complex processes of child development and behavior. Over the last several decades, science has amassed substantial evidence to document the importance of both nature and nurture.1 Moreover, current research is elucidating the complex ways in which nature and nurture interact throughout the childhood years. This chapter attempts to provide a framework in which to consider how nature, nurture, and their interactions shape children’s lives. Many examples are provided of both innate and experiential factors that influence children’s development and behavior, and of the mechanisms through which those factors are believed to act. Throughout the chapter, the reader is asked to hold 2 overarching concepts in mind: individual variability and developmental plasticity. Because of individual variability, children differ in how any factor may shape their development and behavior regardless of whether that factor is innate or environmental. As research is beginning to show, much of this variability may be rooted in the interaction of nature and nurture, also known as gene by environment interaction or GxE. Because of developmental plasticity, the effects of both innate and experiential factors can be either augmented or ameliorated by other factors over time. No developmental influence, whether innate or environmental, should be regarded as deterministic, strictly consigning a child to a certain fate. Rather, the processes of development continue throughout childhood, adolescence, and even adulthood, allowing biological and behavioral interventions to shape later outcomes. Innate Factors in Child Development and Behavior Nature can be variably construed in either a narrow or a broad manner. From the narrow perspective, a child’s nature is defined as the child’s innate biological endowment and heredity and, thus, consists primarily of genetics. From the broader perspective, all factors that are believed to operate through a direct biological mechanism are construed as being within the category of nature. Genetics Research on the influence of nature on children’s behavior and development started in earnest with twin studies, which examine the resemblance of monozygotic (identical) twins to each other and the resemblance of dizygotic (fraternal) twins to each other. Data from these twin studies are commonly summarized in a numerical parameter known as heritability, which can range from 0 to 1.00 and is symbolized as h2. For example, studies of attention-deficit/hyperactivity disorder (ADHD) estimate its heritability to be between 0.60 and 0.90, while IQ studies estimate the heritability of intelligence 0.50 to 0.85 with values tending higher with increasing age (ie, genetic factors have a larger influence in older ages).2 It should be understood that heritability is an abstract mathematical parameter that does not translate easily to tangible interpretation. That is, if the heritability of reading disability is 0.75, it does not imply that 75% of all cases of dyslexia have an exclusively genetic etiology, or that the child of a person with dyslexia has a 75% chance of having dyslexia, or any such implication. Heritability merely describes the proportion of the statistical variance in a trait that was attributable to genetics in a particular research study. Behavioral genetic studies commonly yield estimates of heritability that are greater than 0.50 for many developmental-behavioral diagnoses and traits,3 leading some commentators to claim that biology is more important than environment. Such a claim is misleading at best. First, the word more is confusing in this context. Even when the estimate of heritability is high, it cannot be concluded that any particular case of a disease is “more” caused by genetic or environmental factors or that more cases of that disease are caused by genetics than environment. Since no study of any trait has shown complete genetic heritability (ie, heritability has always been found to be <1.00), and since even identical twins do not show 100% concordance for any diagnosis or trait (eg, autism, schizophrenia, reading skills; Figure 2.1), it implies that environmental factors can make a clinically significant difference even when 2 individuals are genetically identical. It seems much more likely that all or almost all cases of a disease have both genetic and environmental influences in their pathogenesis. Robert Plomin, one of the most prominent researchers in the field, has commented that behavioral genetic studies can be regarded as providing some of the best evidence of the importance of environmental factors in shaping health and disease.4 A further argument against the overinterpretation of heritability parameters is that they are dependent on the amounts of genetic and environmental variation in the populations under study. For example, if all the subjects in a study had identical environments, the study would show misleadingly high estimates of genetic heritability and low estimates of environmental influence. In fact, it is well known that extreme environmental manipulation can have enormous effects on behavior and development. Common, real-life variability in the environment also affects estimates of heritability, as Turkheimer and colleagues5 demonstrated. They found that estimates of the heritability of IQ are higher in populations with higher socioeconomic status (SES), while heritability is near zero in populations of lower SES. Explanations for this finding are only speculative, but it seems possible that families with higher SES provide a more consistently beneficial environment to their children, thus minimizing the environmental differences between them and thereby making genetics a larger source of variance. In populations with lower SES, on the other hand, some children may encounter more beneficial environments (eg, a particularly nurturing teacher) while others do not, which results in higher estimates of environmental variance in IQ and minimal genetic heritability. Figure 2.1. Twin concordances for learning disabilities and for psychiatric disorders. Abbreviations: MZ, monozygotic; DZ, dizygotic. Reproduced from Haworth C, Plomin R. Quantitative genetics in the era of molecular genetics: learning abilities and disabilities as an example. J Am Acad Child Adolesc Psychiatry. 2010;49(8):783–793, with permission from Elsevier. With the advent of molecular genetic methods, twin studies have been supplanted by research that examines specific genes and their effects on child behavior and development. Genome-wide association studies (GWASs) provide one example of this new approach. In these studies, single nucleotide polymorphisms, which are commonly found in the general population, are studied in relation to phenotypic traits. In general, GWASs are suited to finding genetic differences that are relatively common but have relatively weak effects on the risk of having a medical condition, whether that disease is emphysema or a reading disability. More recently, next-generation genetic sequencing methods have been applied to the task of finding the genetic roots of behavior and development. These methods include whole exome sequencing and whole genome sequencing, in which every base pair in a person’s genome is sequenced, whether it is found in an exon, in an intron, or in the intervening segments that comprise 98% of our DNA (formerly referred to as “junk DNA”). These “next-gen” studies are finding variations that are rare in the population as a whole but that may carry much stronger disease risk.6 (It is reasoned that if a genetic difference carried a high risk for a serious medical condition, then it could not be common in the population, as it would decrease the fitness of individuals who have it.) Even when differences in genotype are found, science is faced with the daunting task of understanding how these differences in genetic sequence give rise to seemingly dramatic phenotypic differences. Genes and their protein products interact in enormously complex networks, regulating the expression and effect of other genes and proteins, and scientists will be busy for many decades unraveling this complexity. Genetic factors can have either large or subtle influences on child development and behavior. Examples of the former include genetic diagnoses that are associated with intellectual disability or severe behavioral abnormalities. These disorders can result from single gene mutations (eg, in the FMR1, HPRT1, or MECP2 genes associated with fragile X syndrome, Lesch-Nyhan syndrome, and Rett syndrome, respectively) or from genetic conditions that affect multiple genes (eg, contiguous gene deletion syndromes such as Williams syndrome or velocardiofacial syndrome, chromosomal aneuploidies such as Down syndrome and segmental chromosomal deletions or duplications). In some of these disorders, the exact pathogenic mechanism is not fully understood. For example, it is still not conclusively known which genes on the triploid chromosome 21 have significant roles in causing the neurobiological differences associated with Down syndrome. In other disorders, including fragile X syndrome and many metabolic conditions, such as Lesch-Nyhan syndrome, the pathogenesis is understood to a greater extent. In contrast to the large effects of mutations in genes such as FMR1, HPRT1, and MECP2, mutations and variants in other genes are believed to have more subtle effects on the risk for conditions such as reading disability, ADHD, and other learning disabilities. For some conditions, such as autism spectrum disorder (ASD), research suggests that there are both large-effect and small-effect genes. Mutations in the SHANK3 and CHD8 genes, for example, are associated with a large risk for autism, but mutations in large-effect genes such as these are estimated to account for only 30% to 40% of all cases of ASD, at most, while most cases of ASD are believed to be associated with small-effect mutations or common variants in other genes.7 These genetic differences may not give rise to autism on their own but may work with other genetic risk factors or with yet-unspecified environmental factors to cause autism. Because of the smaller effects of these genes, however, and their complex interactions with other factors, they have been difficult to identify and to confirm. Individual variability in clinical manifestations is present both for small-effect common variant genetic differences but also for the large-effect genes. For instance, only 25% to 30% of individuals with fragile X syndrome, associated with the FMR1 gene, meet full diagnostic criteria for autism. Even the level of intellectual disability seen in fragile X syndrome varies widely among those with the full mutation. Similarly, mutations in CHD8 are not fully penetrant in causing autism; perhaps only 50% of those with mutations are diagnosed with autism, while other affected individuals may have other developmental-behavioral disorders (see Chapter 11, Making Developmental-Behavioral Diagnoses). The same general pattern is seen with copy number variations, in which a segment of DNA is either deleted, duplicated, or repeated more than 2 times. For example, among individuals with deletions of the 16p11.2 region, about 25% have ASD, more than half have a language-related disability, and a small percentage do not meet criteria for any developmental or psychiatric disorder at all.8 Related research has shown that the genetic risk factors for many psychiatric disorders, from schizophrenia to mood disorders to ASD to ADHD, overlap widely, suggesting that individual variability in outcome is a universal rule rather than an exception. What is the source of this variability? One undeniable answer is that environmental factors interact in very important ways with genetics, even for the classic, severe genetic disorders. These disorders were long thought to result in preordained, minimally changeable developmental outcomes. But for many metabolic disorders, with phenylketonuria serving as a particularly well-known example, early diagnosis and careful nutritional management can greatly enhance outcome. Even in chromosomal disorders such as Down syndrome where a very large number of genes are affected, the past decades have yielded powerful evidence for developmental variation that depends on environmental factors. When Down syndrome was first recognized in the medical literature, almost all affected individuals had moderate or severe intellectual disability, and virtually all were confined to state-run residential institutions. Today, children with Down syndrome typically grow up with their own families; they receive extensive early intervention and special educational support, and their cognitive and functional capacities are far greater than they were a generation ago.9 Epigenetics Even from the narrow perspective of what “nature” means, it is now recognized that, besides genes, there are epigenetic factors that must be considered a part of every child’s biological endowment. Epigenetic factors are biological differences related to genes but not involving the sequence of base pairs in a person’s DNA. The best understood example of this is imprinting, by which a child’s DNA is somehow marked as having come from either the mother or the father. For example, the genetic syndromes of Prader-Willi and Angelman both can result from a deletion at chromosome 15q11-q13. If this deletion occurs on the chromosome 15 that was inherited from the father, then the child is affected by Prader-Willi syndrome, but if the deletion occurs on the maternally inherited chromosome 15, then the child will have Angelman syndrome. Fragile X syndrome provides another example of an epigenetic phenomenon. In almost all cases, the mutation that causes the syndrome, which is found in the promoter region of the FMRP gene, is accompanied by methylation of the FMRP gene. This methylation blocks the gene from being expressed, causing the affected child to fully manifest fragile X syndrome. In rare cases, however, the mutated gene is not methylated, allowing some FMRP to be produced and resulting in less severe symptoms. A third possible example of an epigenetic modification is found in Turner syndrome.10 In Turner syndrome, girls have only one X chromosome, which can be inherited from either their father or their mother. According to some reports, social and cognitive skills in girls with Turner syndrome may differ depending on whether their only X chromosome comes from their father or from their mother. Other medical characteristics, such as body mass index, cholesterol levels, and the presence of renal malformations, may also be a function of the parent-of-origin of the X chromosome. Researchers hypothesize that there may be a gene or genes on the X chromosome that are active in males (who have only one X chromosome) but may be methylated and turned off on one of the female X chromosomes, and that some differences may also be found after the X chromosome is passed on to the child with Turner syndrome. Intriguing research has shown that monozygotic twins, who by definition have identical genetic sequences, can have differences in their epigenetic states.11 As a corollary, the twins can have differences in the extent to which the epigenetically regulated genes are expressed, which would lead to differences in their phenotypes. The data show that twins’ epigenetic differences increase with age, particularly if the twins live apart from each other. The tentative conclusion is that the different environments that the twins experience are the cause of their epigenetic divergence and, thus, of the phenotypic differences that arise between identical twins. This conclusion is supported by findings in animal research that an individual’s epigenetic state can change during life as a consequence of specific environmental experiences. Biological Experiences The broad perspective on nature’s influences includes not only genetic and epigenetic factors but also biological events that are experienced either prenatally or postnatally. Examples include traumatic brain injury, hypoxic-ischemic encephalopathy, meningoencephalitis, congenital brain malformations, and prenatal drug exposures. Scientists recently established that prenatal exposure to tobacco smoking leaves a biological signature on the child’s epigenome—specifically, the pattern of DNA methylation remains different, even at age 3 to 5 years, in children whose mothers smoked during pregnancy.12
Stay updated, free articles. Join our Telegram channel

Full access? Get Clinical Tree
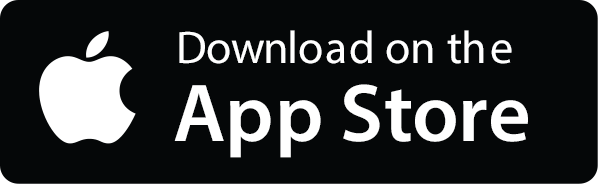
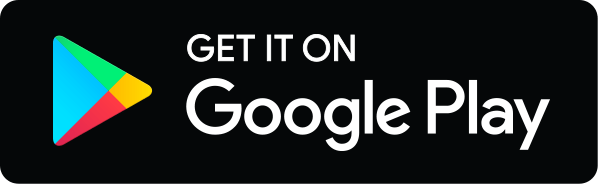