37 Musculoskeletal Disorders
Musculoskeletal complaints are reported in 4% to 30% of young people. In most cases the causes of pediatric musculoskeletal symptoms are benign and self-limiting (Jandial et al, 2009). Sports-related injuries are found to be the highest causative factor of musculoskeletal complaints in 5- to 24-year-olds (Benjamin and Hang, 2007). Common musculoskeletal disorders include athletic injuries, back pain, foot injuries, knee disorders, shin splints, and stress factors. Inborn problems include spinal deformities, hip and foot anomalies, growth disorders and developmental delay, metabolic disorders, and neuromuscular disorders ranging from cerebral palsy to muscular dystrophy. A variety of other conditions can cause musculoskeletal findings, including child abuse, trauma, cancer, and juvenile idiopathic arthritis. Iatrogenic deformities that result from cultural practices, such as using a cradleboard, or from in utero packing problems can also cause deformities. Disorders of the musculoskeletal system present unique problems because growth and development of this system contribute to the evolution of pathologic conditions over time. Limited mobility, pain, and deformity can interfere with the lifestyle of the child. Children with functional disabilities may not be able to fully participate in all activities with peers and family or have access to various occupations. They may also face challenges related to self-esteem. Primary care providers must be vigilant and seek to help children and their families prevent these problems.
Primary care providers play a significant role in the early identification and management of children with orthopedic problems. Primary care providers assess development of the musculoskeletal system, identifies problems for early intervention, focus on lifestyle and injury prevention, and monitor the long-term outcomes of orthopedic care. They are often the first to refer to specialists as needed for early diagnosis and treatment. When necessary, primary care providers help families integrate orthopedic care within the daily living activities at home and school and assist families to cope with the issues of disability, deformity, and long-term care.
Anatomy and Physiology
Limb formation occurs early in embryogenesis (4 to 8 weeks of gestation); primary ossification centers are present in all the long bones of the limbs by the twelfth week of gestation. Limb abnormalities occur in approximately 6 in 10,000 live births (Koifman et al, 2008). Development of the skeletal system begins around the fourth week of gestation, with ossification of the fetal skeleton beginning during the fifth month of gestation. The clavicles and skull bones are the first to ossify, followed by the long bones and spine. The epiphyses of the newborn’s long bones are composed of hyaline cartilage. Soon after birth the cartilage along the epiphyseal plate begins secondary ossification. The shape of the spine also changes from a C shape at birth to a double S curve by late adolescence. As the child starts to walk, the lumbar curve develops. The sacrum starts out as five separate bones at birth only to become fused as one large bone by 18 to 20 years old (Duderstadt and Schapiro, 2006).
Bone age, measured by radiographs of the left hand and wrist, can be used to quantitatively determine somatic maturation and serves as a mirror that reflects the tempo of growth. In adolescents, the skeletal growth spurt begins at about Tanner stage 2 in girls and Tanner stage 3 in boys. Growth peaks around stage 4 and then ends with stage 5. The growth spurt lasts longer in boys than in girls. The pelvis widens early in pubescent girls. In both sexes the legs usually lengthen before the thighs broaden. Next the shoulders widen, and the trunk completes its linear growth. Bone growth ends when the epiphyses close.
Long bones have a growth plate, or physis, at each end that separates the epiphysis from the diaphysis or shaft. Openings through this plate allow blood vessels to penetrate from the epiphysis. In the growth plate, chondrocytes produce cartilage cells, dead cells are absorbed, and the calcified cartilage matrix is converted into bone. The entire growth plate area is weaker than the remaining bone because it is less calcified. Because the blood supply to the growth plate comes primarily through the epiphysis, damage to epiphyseal circulation can jeopardize the survival of the chondrocytes. If chondrocytes stop producing, growth of the bone in that area stops (Fig. 37-1).
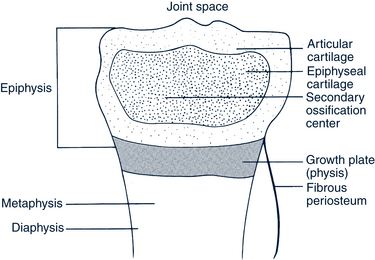
FIGURE 37-1 Anatomy of long bones.
(Modified from Shapiro F: Epiphyseal disorders, N Engl J Med 317:1702-1710, 1987.)
There are two ways that children’s bones grow. Longitudinal growth occurs in the ossification centers; changes in bone width and strength take place via intramembranous ossification. The length of long bones comes from growth at the epiphyseal plates while their diameter increases as a result of deposition of new bone on the periosteal surface and resorption on the surface of the medullary cavity. Growth of the small bones, hip, and spine comes from one or more primary ossification centers in each bone. Apophyses are the sites for connection of tendons to bone. In children these sites, similar to epiphyses, allow for growth and are weaker than bone. These sites can become inflamed with stress.
The development of bones and muscles is influenced by use. Thus in infants and toddlers, the legs straighten and lengthen with the stimulus of weight bearing and independent walking. The infant is born with the full complement of muscle fibers. Growth in muscle length results from lengthening of the fibers, and growth in bulk comes from hypertrophy. Length of muscles is related to growth in length of the underlying bone. If a limb is not used, it grows minimally. Furthermore, if muscles and bones are not used in their intended normal manner, such as occurs with spastic diplegia, the forces for development tend to stimulate growth in abnormal patterns. Thus scoliosis can develop or bowlegs may increase in severity. Muscle contractures occur if muscles are not used regularly and put through their full range of motion. The growth of fibrous tissue, tendons, and ligaments is also dependent on mechanical demands.
Nutritional, mechanical, and hormonal factors during the growth process influence the thickness of bones and the health of the marrow. Adequate protein, calcium, and vitamin D in the diet are key nutritional elements that affect the growth and development of a child’s musculoskeletal system.
The muscle structures originate from the embryonic mesoderm and include tendons, ligaments, cartilage, and joints. Muscle fibers are developed by the fourth or fifth month of gestation and grow in tangent with their respective bones. The rate of muscle growth (muscle mass and cell sizes) speeds up dramatically around 2 years old, with girls exhibiting a greater rate of growth than boys until this gender trend is reversed at puberty (Duderstadt and Schapiro, 2006).
Pathophysiology and Defense Mechanisms
Pathophysiology
Muscles and bones can be affected by localized or systemic problems. Thus the initial orthopedic problem can be symptomatic of a larger problem.
Systemic Problems
Musculoskeletal presentation in children and adolescents can be a feature of potentially life-threatening conditions such as sepsis, malignancy or nonaccidental injury, and chronic pediatric conditions such as inflammatory bowel disease, cystic fibrosis, and juvenile idiopathic arthritis.
Systemic problems can include chronic conditions such as hemophilia, sickle cell disease, and arthritic diseases; neurologic problems such as cerebral palsy; and various cancers, including osteosarcomas and leukemias. Children with metabolic problems, such as vitamin D–resistant rickets, have bony deformities. Acute systemic problems can also affect the musculoskeletal system. For example, viruses and bacteria can infect joints and bones. In developing countries, tubercular infections of bones are common and devastating. Thus the pediatric provider must assess patients from a broad perspective, asking questions about other body systems and ordering appropriate laboratory studies that might identify systemic problems.
Genetic Problems
Many genetic problems have an orthopedic component. Osteogenesis imperfecta (OI) is a genetic disorder characterized by decreased levels of collagen, the major protein of the body’s connective tissue. Children with OI have bones that break easily, even from minor trauma. Down syndrome is a consequence of a genetic anomaly of chromosome 21. The main orthopedic pathology is hypotonia and the possibility of loose capsule and ligaments. Children with Down syndrome have a higher incidence of scoliosis, dislocation of the hip, Legg-Calvé-Perthes disease (LCPD), instability of the patella, and pes planus (flat feet). Children with Marfan syndrome may have long spider-like fingers, low muscle tone, and lax joints that are prone to dislocate. Severe scoliosis may develop in children with neurofibromatosis, Turner syndrome, and Noonan syndrome. Girls with Turner syndrome may present with webbed neck, short stature, valgus deformity of the elbow, and short fourth metacarpal deformity. Children with Noonan syndrome can present with webbed neck, clumsiness, poor coordination, and motor delay.
Many orthopedic problems have a multifactorial inheritance pattern. Thus if one child in a family has a dislocated hip or scoliosis, the risks increase for other children. The pediatric provider needs to understand the genetic disorder to monitor related orthopedic problems, consider the genetic implications, and provide families with appropriate genetic information or refer them for genetic counseling (see Chapter 40).
Uterine Packing Deformations
The developing fetus moves its body parts frequently, and this movement influences musculoskeletal development. When the baby fills the uterine space, movements are restricted and body parts begin to assume a shape in which they are fixed. With in utero positioning, joints and muscle contractions can develop and are considered generally physiologic in nature. Orthopedic deformities of uterine compression include clubfoot, torticollis, hip dislocation, metatarsus adductus, equinovarus foot, calcaneovalgus foot, tibial bowing, hyperflexed hips, hyperextended knees, contractures, and internal tibial torsion (Wynshaw-Boris and Biesecker 2007). Fetal movement is required for proper development of the musculoskeletal system, and anything that restricts fetal movement can cause deformation from intrauterine molding. Two major intrinsic causes for deformations are neuromuscular disorders and oligohydramnios. Extrinsic causes are related to fetal crowding that restricts fetal movement. For a fetus in the breech position the incidence of deformations is increased 10-fold (Wynshaw-Boris and Biesecker, 2007). Infants with deformations caused by extrinsic causes have an excellent prognosis with corrections occurring spontaneously. Torsional and angular alignment issues can affect the long bones, most commonly those of the lower extremities. Because much of the bony structure is cartilaginous, molding occurs with relative ease. Thus in normal newborns, the tibias are normally bowed, and the hips have a 20- to 30-degree flexion (Hosalkar and Wells, 2007). Occasionally, a foot may be turned awkwardly, the legs might be fixed straight up with the feet near the ears, or the neck may be tipped to one side. Such positioning issues are outside the range of normal. The outcomes are deformities in various degrees. The longer the position is maintained, the more severe the problems are. In general, there is a tendency for bowing and late deformations to straighten; however, the effects related to in utero positioning may not fully abate until the child is 3 to 4 years old. More severe deformities (i.e., those significantly outside the range of normal) need to be referred to orthopedists for treatment as soon as they are found because a softer skeleton is easier to realign in a positive direction.
Injuries
There are approximately 2.6 million emergency department visits annually in the U.S. for sports-related injuries in children ages 5 to 24 years, with the highest percentage occurring in the 5- to 14-year-old male. This does not account for the children who present to their primary care physician for evaluation and treatment (Soprano and Fuchs, 2007). The unique differences in the pediatric skeletal system predispose children to injuries unlike those seen in adults. The important differences are the presence of periosseous cartilage, physes, and a thicker, stronger, more osteogenic periosteum that produces new bone called callus more rapidly and in great amounts. Joint injuries, dislocations, and ligament disruptions are infrequent in children (Gholve et al, 2007). Physeal fractures in preadolescent children are the most common musculoskeletal injuries seen. Clavicular fractures are seen at all ages ranging from a newborn birth injury to trauma in adolescence. They can occur as a result of direct or indirect injury and are most commonly associated with a fall (Benjamin and Hang, 2007).Tendinoses are seen infrequently in children but may occur in the young athlete in the rotator cuff from throwing motions and swimming, in the iliopsoas in dancers, and in the ankle of dancers, gymnasts, and figure skaters. Shoulder injuries can be acute or result from chronic overuse. Overuse injuries are common chronic injuries in children that are related to repetitive stress on the musculoskeletal system without sufficient time to recover. The repetitive use overwhelms normal reparative processes (Soprano and Fuchs, 2007). Management of traumatic injuries is discussed in Chapter 39.
Defense Mechanisms
Fracture Healing
One of the major differences between the adult and the pediatric bone is that the periosteum in children is very thick. The major reason for increased healing speed of children’s fractures is the periosteum, which contributes to the largest part of new bone formation around a fracture. Children have significantly greater osteoblastic activity in this area because bone is already being formed beneath the periosteum as part of normal growth. This already active process is readily accelerated after a fracture. Periosteal callus bridges a fracture in children long before the underlying hematoma forms a cartilage anlagen that goes on to ossify. Once cellular organization from the hematoma has passed through the inflammatory process, repair of the bone begins in the area of the fracture. In most children, by 10 days to 2 weeks after fracture, a rubber-like bone forms around the fracture and makes it difficult to manipulate. As part of the reparative phase, cartilage formed as the hematoma organizes is eventually replaced by bone through the process of endochondral bone formation. The remodeling phase of fracture healing may continue for some time and is accelerated by motion of the adjacent joints and use of the extremity (Green and Swiontkowski, 2008).
Growth Plate Fractures
Fractures of the long bones can produce permanent deformities in children if the fracture occurs through the growth plate. The outcomes depend on the fracture location and type, the age of the child, the status of the blood supply to the physis, and the treatment. The Salter-Harris classification is based on the mechanism of injury, the relationship of the fracture line to the layers of physis, and the prognosis with respect to subsequent growth disturbance. There are five classifications (Fig. 37-2). Type I is the most frequent type of fracture that involves a fracture through the zone of hypertrophic cells of the physis with no fracture of the surrounding bone. Type II fractures are similar to type I except that a metaphyseal fragment is present on the compression side of the fracture. Growth disturbance in types I and II is rare.
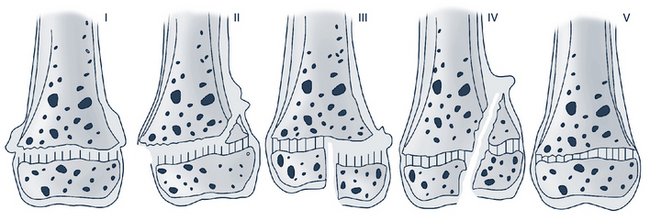
FIGURE 37-2 The types of growth plate injury as classified by Salter and Harris.
(From Salter RB, Harris WR: Injuries involving the epiphyseal plate, J Bone Joint Surg Am 45:587, 1963.)
Type III fracture involves physeal separation with fracture through the epiphysis into the joint. The fracture requires anatomic reduction, occasionally through an open approach. Type IV fracture involves the metaphysis, physis, and epiphysis. Type V fracture involves a compression or crushing injury to the physis. Type V fractures are rare and are difficult to diagnose initially due to the lack of radiologic signs. Types IV and V require anatomic reduction to prevent articular incongruity and osseous bridging across the physis. There are additional rare types of Salter-Harris fractures, types VI through IX. Type VI is an injury to the perichondral ring of LaCroix, a fibrous band that is continuous with the periosteum and a potential reservoir for growth-plate germ cells. Type VI fracture is seen in injuries about the medial malleolus and often requires later reconstructive surgery (Green and Swiontkowski, 2008).
Although 30% of Salter-Harris fractures result in growth disturbances, only 2% result in significant functional disturbance (Moore and Smith, 2009).
Shaft Fractures
The mechanism of injury is an important part of the history in evaluating a child for a traumatic injury. Closed pediatric fractures are largely caused by low-energy activities and play; open fractures are generally caused by more violent accidents. Open fractures in children younger than school age are rare because of their small body mass, large amount of protective subcutaneous fat, and their limited exposure to high-risk activities (Sabharwal and Bhrens, 2008).
There are a variety of shaft fractures. In children between 9 months and 6 years of age, torsion of the foot may produce an oblique fracture of the distal aspect of the tibial shaft without a fibula fracture. These fractures are usually the result of tripping while walking or running, stepping on a ball or toy, or falling from a modest height. The child is typically seen due to failure to bear weight, a limp, or pain when asked to stand on the involved extremity. Physical findings may be minimal and radiographs may show the characteristic faint oblique fracture line crossing the distal tibial diaphysis and terminating medially. Treatment is immobilization. Fractures of the forearm represent 40% of all fractures in children (Dolan and Waters, 2008). Fractures of the forearm in children most often result from a fall on an outstretched hand. This results in forceful axial loading with resultant bony failure in compression and bending. These forces generally cause a plastic deformity or partial fracture (greenstick fracture). The rotational malalignment may not be identified and may be undertreated. During physical examination the provider should observe for subtle rotational deformities and compare with the contralateral limb. Plain radiograph remains the gold standard for musculoskeletal evaluation and should be performed on all patients with a physical examination suggestive of fracture or dislocation (Dolan and Walters, 2008). Malrotation is indicated on x-ray by malalignment of the elbow in relation to the wrist. Failure to diagnose and treat rotational malalignment is the most common cause of loss of forearm rotation in children.
Assessment of the Orthopedic System
History







Physical Examination
Special orthopedic examination techniques are described in the following paragraphs.
Range-of-Motion Examination
Range of motion is the normal range, flexion, extension, and rotation of a joint. Joint hypermobility is the ability of the joint to move beyond its normal range. Hypermobility of joints generally does not cause problems, although there is a slight increase in dislocation and sprain of the involved joint. The normal values of joint motion are age related, which must be kept in mind (e.g., external hip rotation is greatest in early infancy). Passive range of motion, in which the examiner moves the joint, provides information about joint mobility and stability. It can also provide information about the limits of tendons and muscles that are contracted. Active range of motion, in which the child moves the joint, provides information about both muscle and bony structures working together for functional movement.
Limited range of motion can be the result of mechanical problems, swelling, muscle spasticity, pain, infection, injury or arthritis. Note pain, stiffness, limitations or deviations, and rigidity.
Gait Examination
A normal gait cycle consists of the stance phase, during which the foot is in contact with the ground, and the swing phase, during which the foot is in the air. The stance phase is further divided into three major periods: the initial double-limb support, followed by the single-limb stance, then another period of double-limb support. The gait undergoes developmental changes. Walking velocity, step length, and duration of the single-limb stance increase with age, whereas the number of steps taken per minute decreases. A mature gait pattern is well established by 3 years of age. By 7 years of age the gait is that of an adult (Sawyer and Kapoor, 2009).
Observe the child walking without shoes and minimal covering. The stance and swing phases should be compared in both legs, and the range of motion of each joint should be evaluated. Inspect from the front, side, and back as the child walks normally, on his or her toes, and then on the heels. The gait should be smooth, rhythmic, and efficient. Ankle, knee, and hip movements should be symmetric and full with little side-to-side movement of the trunk.
Limping is a disturbance in gait. Abnormal gait can be antalgic or non-antalgic. An antalgic gait is characterized by a shortening of the stance phase to prevent pain in the affected leg. Painful or antalgic gaits serve to reduce stress or pain at the affected area. The trunk shifts to the opposite side to keep balance and reduce stress; the stance phase and stride length are shortened as compensatory mechanisms. Causes of a painful gait include infection, trauma, or acquired disorders. A Trendelenburg gait in which the trunk tips over the affected hip indicates hip disease and might or might not be painful because it also involves muscle weakness around the hip joint.
Posture
To assess posture adequately, the child should be examined undressed to his or her underwear. The examiner needs to look at the child from the front, side, and back.
• Pelvis and hips should be level. Place hands on the iliac crest to test for a pelvic tilt caused by limb length discrepancy.
• Legs should be symmetric in shape and size. The patellae should be straight ahead.
• The feet should point straight ahead, with an imaginary line from the center of the heel through the second toe. There should be an arch (except in babies, in whom a fat pad obscures the arch) and straight heel cords.
• The spine should be straight, and the back should look symmetric, with shoulder and scapula heights and waist angles equal. There should be slight lordotic curves at the cervical and lumbar areas.
Special Examinations
Hip Examinations
Galeazzi Maneuver
The Galeazzi sign can signal conditions that cause leg length discrepancies. The Galeazzi maneuver includes flexing the hips and knees while the infant or child lies supine, placing the soles of the feet on the table near the buttocks, and then looking at the knee heights for equality (Fig. 37-3, A). The Galeazzi sign is positive if the knee heights are unequal. However, it is not reliable in children with dislocatable but not dislocated hips or in children with bilateral dislocation.
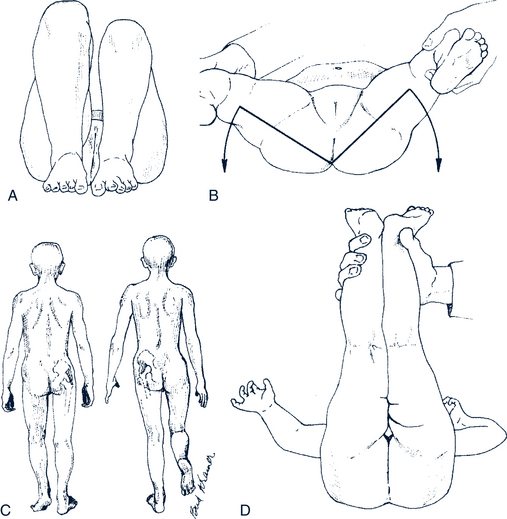
FIGURE 37-3 Physical findings in congenital hip dislocation. A, Leg length inequality is a sign of unilateral hip dislocation (Galeazzi sign). B, Limitation of hip abduction is often present in older infants with hip dislocation. Abduction of greater than 60 degrees is usually possible in infants. Restriction or asymmetry indicates the need for careful radiologic examination. C, Trendelenburg sign. In single-leg stance the abductor muscles of the normal hip support the pelvis. Dislocation of the hip functionally shortens and weakens these muscles. When the child attempts to stand on the dislocated hip, the opposite side of the pelvis drops. D, Thigh-fold asymmetry is often present in infants with unilateral hip dislocation. An extra fold can be seen on the abnormal side. The finding is not diagnostic, however. It may be found in normal infants and may be absent in children with hip dislocation or dislocatability.
(From Scoles P: Pediatric orthopedics in clinical practice, ed 2, St Louis, 1988, Mosby.)
Barlow Maneuver
The Barlow maneuver dislocates an unstable or dislocatable hip posteriorly (Fig. 37-4, A). The infant is placed in the supine position with knees flexed. The hip is flexed, and the thigh is brought into an adducted position applying downward pressure. With hip instability the femoral head slips/drops out of the acetabulum or can be gently pushed out of the socket; this is termed a positive Barlow. The dislocation should be palpable as this maneuver is performed. The maneuver needs to be done gently in a noncrying neonate to keep from damaging the femoral head. The hips should be examined one at a time. The hip generally spontaneously relocates after release of the posterior force.
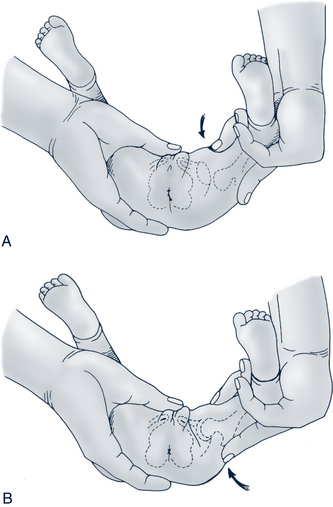
FIGURE 37-4 A, Barlow (dislocation) test. The “stabilizing hand” is positioned with the thumb on the symphysis and the fingers on the sacrum. The thumb of the abducting hand is placed on the inner aspect of the thigh and gives lateral pressure to the adductor region while the hand (wrapped around the knee with the index finger on the lateral side of the thigh) provides gentle downward pressure. If there is hip instability, dislocation is palpable as the femoral head slips out of the acetabulum. Diagnosis is confirmed with the Ortolani test. B, Ortolani (reduction) test. With the infant relaxed on a firm surface, the hips and knees are flexed to 90 degrees. The hips are examined one at a time. Grasp the infant’s thigh with the middle finger over the greater trochanter and lift the thigh to bring the femoral head from its dislocated posterior position to opposite the acetabulum. Simultaneously the thigh is gently abducted, reducing the femoral head in the acetabulum. In a positive finding, the examiner senses reduction by a palpable, nearly audible “clunk.” Test one hip at a time for both of these tests.
(From Marcdante KJ, Kliegman RM, Jenson HB et al, editors: Nelson essentials of pediatrics, ed 6, Philadelphia, 2011, Saunders.)
Ortolani Maneuver
The Ortolani maneuver can be done after the Barlow maneuver or separately (see Fig. 37-4, B). The Ortolani maneuver reduces a posteriorly dislocated hip. It is done to reduce a recently dislocated hip and is not done forcefully. The infant is in the supine position with both knees flexed and supported by the thumb and forefinger of the examiner. The thumb is placed near the lesser trochanter, and the pad of the second finger is positioned on the bony prominence of the greater trochanter. The leg is flexed at the hip and then abducted while pushing up with the fingers located over the trochanter posteriorly. The femoral head is lifted anteriorly into the acetabulum. A clunk and a palpable jerk are obtained as the femoral head is relocated. A mild clicking sound is not a positive Ortolani sign. These are common and normal sounds radiating from the knee or ankle that are fine, of short duration, and high pitched (Duderstadt and Schapiro, 2006). Of note, the hip may be dislocated easily only during the first month or two of life. The Ortolani maneuver is most likely to be positive in infants 1 to 2 months old. The examiner should not still be charting “no hip click” on examinations at 6 months old. Dislocation can occur late in infancy. However, if this occurs, the provider will note limited abduction on the side of the dislocation (Fig. 37-5).
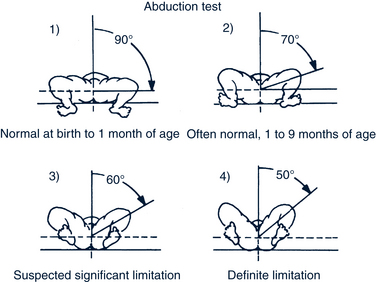
FIGURE 37-5 Hip abduction test. The child is placed supine, and the hips are flexed 90 degrees and fully abducted. Although the normal abduction range is quite broad, one can suspect hip disease in any patient who lacks more than 35 to 45 degrees of abduction.
(From Chung SMK: Hip disorders in infants and children, Philadelphia, 1981, Lea & Febiger.)
Klisic Test
The Klisic test provides an observational sign of hip placement. The examiner places the tip of the third finger of one hand over the greater trochanter and the index finger of the same hand on the anterior superior iliac spine. An imaginary line is then drawn between the index and third fingers. Normally, the imaginary line points to the umbilicus. If the hip is dislocated, the imaginary line points halfway between the umbilicus and the pubis (i.e., the line points below the umbilicus). The Klisic sign is another physical assessment marker of dislocation (Hosalkar et al, 2007b) (Fig. 37-6).
Trendelenburg Sign
The Trendelenburg test can be used to identify conditions that cause weakness in the hip abductors. The Trendelenburg sign is elicited by having the child stand and then raise one leg off the ground. If the pelvis (iliac crest) drops on the raised leg side, the sign is positive and indicates weak hip abductor muscles on the side that is bearing the weight. Normally the muscles around a stable hip are strong enough to maintain a level pelvis if one leg is raised (see Fig. 37-3, C). With bilaterally dislocated hips, a wide-based Trendelenburg limp is noted.
Medial (Internal) and Lateral (External) Rotations
The child is placed prone, and the knees are flexed 90 degrees. Medial rotation is measured as the legs are allowed to fall apart as far as possible, using gravity alone or with light pressure. The angle between vertical (0 degree) and the leg position is the medial rotation. It is measured for each leg (Fig. 37-7, A). Asymmetric hip rotation is abnormal. Lateral rotation is measured by allowing the legs to cross while the child is still prone. The angle between vertical and the leg position is measured for each leg (see Fig. 37-7, B). Again, asymmetric hip rotation is abnormal. By 1 year old, a normal child has approximately 45 degrees of internal and external hip rotation.
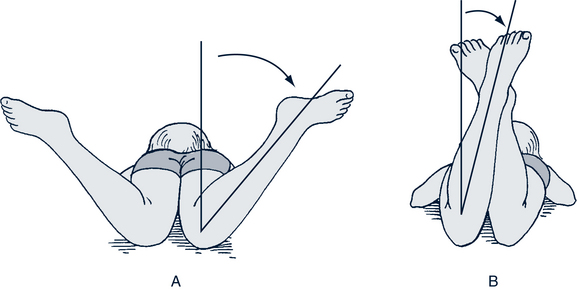
FIGURE 37-7 Hip rotation in extension. This is measured with the child in prone position and the knee flexed 90 degrees. The lower leg is vertically oriented. This is considered the neutral position. On outward rotation (A), the leg produces internal hip rotation, and on inward rotation (B), the leg produces external hip rotation.
(From Thompson GH: Gait disturbances. In Kliegman RM, editor: Practical strategies in pediatric diagnosis and therapy, Philadelphia, 1996, Saunders.)
Back Examination
Adams Test or the Adams Forward Bend Position
The Adams forward bend test (Adams test) looks for asymmetry of the posterior chest wall on forward bending. This position allows for evaluation of structural scoliosis. The child bends at the waist to a position of 90 degrees back flexion with straight legs, ankles together, and arms hanging freely or with palms together (in a diving position) but not touching the toes or floor (Fig. 37-8). The back is inspected for asymmetry of the height of the curves on the two sides or rib hump; the provider inspects the child’s back by looking at it from the rear and side positions. The examiner should be seated in front of the child to best visually scan each level of the spine. If a rib hump is present, a scoliometer, if available, can be used to measure the angular tilt of the trunk. A spinal rotation greater than 5 degrees measured by placing the scoliometer at the peak of the curvature indicates the need for further evaluation (Duderstadt and Schapiro, 2006). Other characteristics of scoliosis to look for include unequal scapula heights, unequal waist angles, unequal iliac crests or shoulders, asymmetry of the elbow to flank distance, and some deviation of the spine from a straight head-to-toe line. Looking primarily at the straightness of the spine, however, can be misleading because scoliosis involves both rotation and misalignment of the vertebrae. The Adams forward bending position accentuates the rotational deformity of scoliosis.
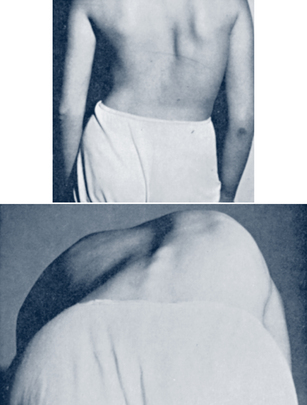
FIGURE 37-8 Adams position with rib hump of structural scoliosis. Lateral curvature of thoracic and lumbar segments of the spine, usually with some rotation of involved vertebral bodies. Functional scoliosis is flexible; it is apparent with standing and disappears with forward bending. It may be compensatory for other abnormalities, such as leg length discrepancy. Structural scoliosis is fixed; the curvature is visible both on standing and on bending forward. Note rib hump with forward flexions. At greatest risk are females 10 years old through adolescence.
(From Delp MH, Manning RT: Major’s physical diagnosis: an introduction to the clinical process, ed 9, Philadelphia, 1981, Saunders.)
Diagnostic Studies
Radiographs are an important diagnostic tool for the musculoskeletal system. Imaging should begin with standard radiographs of the area of concern. Anteroposterior and lateral views of the affected area, bone, or joint are typically ordered to analyze the anatomic structures. Views of both extremities may be ordered so that comparisons can be made. Computed tomography (CT) scans augment radiographs to detail specific areas of the body, especially in identification of soft-tissue lesions. CT is useful in detailing the relationship of bones to their contiguous structures. Magnetic resonance imaging (MRI) gives excellent visualization of joints, soft tissues, cartilage, and medullary bone. It can distinguish among various physiologic changes that occur in bone marrow related to age and disease process. Ultrasonography can provide information about cartilaginous areas or tissues not visible on radiograph. The test is highly sensitive for detecting effusion of the hip joint. Bone scans (scintigraphy) are more sensitive than radiographs, demonstrate abnormal uptake earlier than conventional radiographs, and are useful in detecting causes of obscure skeletal pain.
Laboratory studies can help to identify systemic disease, infection, or inflammation. Erythrocyte sedimentation rate (ESR), C-reactive protein (CRP), complete blood count (CBC), blood cultures, rheumatoid factor, and antinuclear antibodies are hematologic tests that can assist in the diagnosis and management of bone disorders. Other laboratory tests also can provide an understanding of muscle metabolism (e.g., lactic acid, pyruvates, carnitine). Some bony lesions may need to be biopsied, and muscle tissue frequently needs to be sampled to determine specific disease pathologic conditions.
Management Strategies
Counseling
Counseling for orthopedic problems involves several components. The family should understand and have time to ask questions about all of the following issues:
• The pathologic condition, including possible etiologies
• The prognosis with and without treatment
• Any genetic implications of the diagnosis
Counseling helps families cope with a poor or challenging diagnosis and its short-term and long-term implications. Congenital problems are often identified prenatally, at birth, or shortly thereafter. Families need to be given the diagnosis truthfully, humanely, and as soon as possible. Issues of etiology need to be discussed to address parents’ feelings of guilt for causing the problem and to discuss genetic implications, if any. A plan of care that is mutually agreed on by the family and the health care provider must be developed before the infant is discharged from the hospital or clinic.
Exercise
With childhood obesity on the rise, there is a push to improve fitness and activity levels in children and adolescents. Physical activity needs to be promoted at home, in the community, and at school. Toddler and preschool-age children should participate in unorganized, supervised play including running, swimming, tumbling, throwing, and catching. School-age children can be encouraged to increase activity by unorganized outdoor free play, personal fitness (dance, yoga, running), recreational activities, and organized sports. During late childhood and adolescence, strength training may be added. Even children with disabling conditions can exercise in some way. (Refer to Chapter 13 for more in-depth discussion about activities and sports. Tables 13-2 and 13-5 provide some guidance regarding some specific medical conditions and sports participation.) Exercise for children should be fun and perceived as play, not work. Often physical therapists or the child’s orthopedist can provide ideas for safe, therapeutic play or sports activities. At school, children with orthopedic problems should engage in physical activities that are as much a part of the regular physical education class as possible.
Anticipatory Guidance: Musculoskeletal Development
Families are sometimes concerned about problems that providers believe are within normal limits and do not require an orthopedic referral. The provider should provide the child’s family with a description of the child’s predicted musculoskeletal development. Timelines and markers that parents can use to monitor their child’s development are particularly helpful in allowing families to understand their child’s pattern of growth. Misperceptions about the implications of minor variations need to be clarified, and the family should always be given the opportunity to return for further assessment or discussion if concerns remain. Examples of common concerns are flat feet in infants and toddlers, “bowed” legs in toddlers, and “knock-knees” in preschool children.
Shoes
The use of therapeutic shoes to correct orthopedic problems is controversial. Studies confirm that therapeutic shoes do little to correct deformities. Shoes for the average child should keep the feet warm and protected from injury. Shoes should be selected to fit properly and comfortably with room for growth. High-top shoes for toddlers may have the advantage of staying on pudgy little feet better, but they do not provide additional support. Toddler feet do not need extra support. Features of a good shoe are as follows:
• Flexible sole—to allow as much free motion as possible; for young children, test to see if the shoe can be flexed in the parent’s hand
• Flat—do not allow high heels
• Foot shaped—avoid pointed toes or other shapes that are not the normal configuration of the foot
• Fitted generously—better to be too large than too small
• Friction similar to skin—the soles should have the same friction as skin so that they are not slippery
Well cushioned, shock-absorbing shoes are helpful in the child or adolescent athlete in order to decrease the chances of developing overuse syndrome. Shoe modifications may be needed in certain conditions. Shoe lifts are needed if limb length differences exceed 2.5 cm. Orthotics also can be used in certain orthopedic situations to more evenly distribute pressure on the sole of the foot and facilitate function.
Care of Children in Casts and Splints
Casts and splints serve to immobilize orthopedic injuries. They promote healing, maintain bone alignment, diminish pain, protect the injury, and help compensate for surrounding muscular weakness (Boyd et al, 2009a). Splints are noncircumferential immobilizers that accommodate swelling. Splints are used in orthopedic conditions where swelling is anticipated: acute fractures or sprains and for initial stabilization of reduced, displaced, or unstable fractures before orthopedic intervention. Casts are circumferential immobilizers. They provide superior immobilization but are less forgiving and have a higher rate of complications. The use of casts and splints is generally limited to a short period of time. Excessive immobilization can lead to chronic pain, joint stiffness, and muscle atrophy (Boyd et al, 2009b).
The child’s cast should be kept cool, clean, and dry. Cover the cast with plastic wrap or a plastic bag when the child bathes or is in a situation in which the cast may get wet. The only cast that can go in water is a Gore-Tex cast. If the cast becomes wet, a hair dryer set on cool setting can be used for drying small areas. If the cast becomes soiled it can be cleaned with a slightly damp washcloth and cleanser.
The family should be taught how to do a circulatory inspection to check the function of nerves and blood vessels. The child’s toes or fingers below the cast should be pink and warm to touch. The child should be able to feel all sides or his or her fingers or toes when touched and should be able to wiggle the fingers or toes.
The family needs to know when to call the provider: if the toes or fingers are cold to touch and appear pale or blue, complaints of tingling or numbness, inability to move fingers or toes, and excessive swelling. Additional problems with casts, such as foul smell, breakage, or loosening, should be reported.
Physical Therapy
Children with developmental delays, cerebral palsy, spine disorders, and torticollis may benefit from physical therapy. Treatments focus on improving gross and fine motor skills, balance and coordination, strength and endurance, as well as cognitive and sensory processing. Structured physical therapy postorthopedic injury can be helpful.
Orthopedic Problems Specific to Children
Arm Problems
Brachial Plexus Injuries
Description
Brachial plexus injuries are typically classified using Narakas criteria types I through IV (Table 37-1). Brachial plexus injuries can also be classified as type I (Erb-Duchenne paralysis) with C5 through C6 involvement that includes the shoulder and upper arm; type II (Erb-Duchenne-Klumpke paralysis) involves the entire brachial plexus (i.e., C5 through T1 involvement) affecting the shoulder, arm, and hand; or type III (Klumpke paralysis) caused by injury to C8 through T1 with involvement of the lower arm and hand (Zafeirious and Psychogious, 2008).
TABLE 37-1 Brachial Plexus Injury Using Narakas Classification
Name | Nerve and Muscle Involved | Prognosis |
---|---|---|
Narakas type I | C5 and C6; shoulder and biceps | Recovery usually complete |
Narakas type II | C5-C7; shoulder, biceps, and forearm extensors | Recovery usually complete |
Narakas type III | C5-T1 | Variable with complete paralysis of limb; shoulder and biceps recovery is fair to poor with hand recovery variable |
Narakas type IV | C5-T1 | Complete paralysis of the limb and Horner syndrome; shoulder and biceps recovery is fair to poor with hand recovery variable |
Epidemiology
Obstetric brachial plexus palsy results from injury to the cervical roots C5-C8 and thoracic root T1. Most injuries are transient, with full return of function occurring in 70% to 92% of cases and prolonged and permanent disability in a small percentage (Zafeirious and Psychogious, 2008). Risk factors for brachial plexus palsies may be divided into three categories: neonatal, maternal, and labor-related factors. Maternal characteristics include diabetes, obesity, maternal age (more than 35 years), and maternal pelvic anatomy. Vacuum extraction or direct compression of the fetal neck during delivery by forceps can cause stretching of the cervical nerve roots and eventually brachial plexus injury. Other causes may include induction of labor, epidural anesthesia, and postdate gestation (Zafeirious and Psychogious, 2008).
Clinical Findings
History
History should include obstetric history, mode of delivery, and postnatal health of the infant.
Physical Examination
All limbs should be examined for fractures and postnatal neurologic deficit and comprehensive examination of the entire body to identify any other injures that may have occurred during delivery (Zafeirious and Psychogious, 2008). Passive range of motion of involved arm, forearm, hand, and shoulder is assessed. Findings may include:
• Erb palsy, presenting with an adducted arm, which is internally rotated at the shoulder. The wrist is flexed, and the fingers extended, resulting in a characteristic “waiter’s tip” posture.
• Absent bicep reflex with absent Moro reflex on the affected side
• Limp wrist and hand with absent grasp reflex (lower plexus involvement)
• Horner syndrome (ipsilateral ptosis, miosis, enophthalmos, anhidrosis) if the sympathetic fibers of the T1 nerve root are involved
• Occasionally hand paralysis with normal shoulder movement, which is a rare occurrence of an isolated C8 through T1 injury
• Ruptured intraabdominal structures, especially the liver and spleen, which require careful abdominal examination
• Limited neck movement due to damage to the sternocleidomastoid muscle; skull fracture
• Impaired respiratory effort as a result of diaphragmatic paralysis and flaccidity
Diagnostic Studies
Radiologic examination, electrophysiologic studies, and MRI are useful to confirm clinical diagnosis and the extent of the injury. X-rays of the chest and upper limbs are important because they reveal associated injuries such as rib, transverse process, clavicle, or humeral fractures. Chest x-ray is necessary to rule out phrenic nerve injury. Electrodiagnostic studies with electromyography and nerve conduction velocities are used to determine severity of the neural lesion.
Differential Diagnosis
The differential diagnosis of upper extremity paralysis in a newborn includes epiphyseal separation of the humeral head, fracture of the clavicle or humerus, septic arthritis of the upper extremity, spinal cord injury, cervical cord lesions, and congenital varicella of the upper limb (Zafeirious and Psychogious, 2008).
Management
A multidisciplinary team approach is ideal with referral to health professionals who specialize in treating brachial plexus injuries. Referral should be made in the first week of life. Therapy is initially conservative; 95% of infants born with obstetric brachial plexus palsy recover complete function with physical therapy only (Zafeirious and Psychogious, 2008). The initial goal of therapy is to maintain passive range of motion, supple joints, and muscle strength. Radiographs should be taken twice yearly for persistent upper plexus palsy. Indications for surgical exploration and reconstruction of the brachial plexus include failure of recovery of elbow flexion and shoulder abduction from the third to the sixth month of life. The spectrum of nerve surgery includes neurolysis neuroma resection, nerve grafting, and nerve transfers.
Complications
Late sequelae include internal rotation contractures, hypoplasia of the arm, altered sensibility, flexion contractures of the elbow, dislocations of the radial head, and psychological and social consequences.
Shoulder Problems
Clavicle Fracture
Epidemiology
Clavicle fractures are seen in newborns, as a result of birth trauma and in very young children as a result of child abuse. They can occur as a result of a direct hit or indirect trauma and are most commonly associated with a fall. Approximately 80% to 85% of these fractures occur in the middle third of the clavicle and 12% to 15% in the distal third. Although the clavicle is the first bone to ossify, the physis does not close until 23 to 25 years of age (Benjamin and Hang, 2007).
Clinical Findings
Physical Examination
In all children, look for the following:
Diagnostic Studies
Imaging studies are recommended. Radiography with routine clavicle views is sufficient.
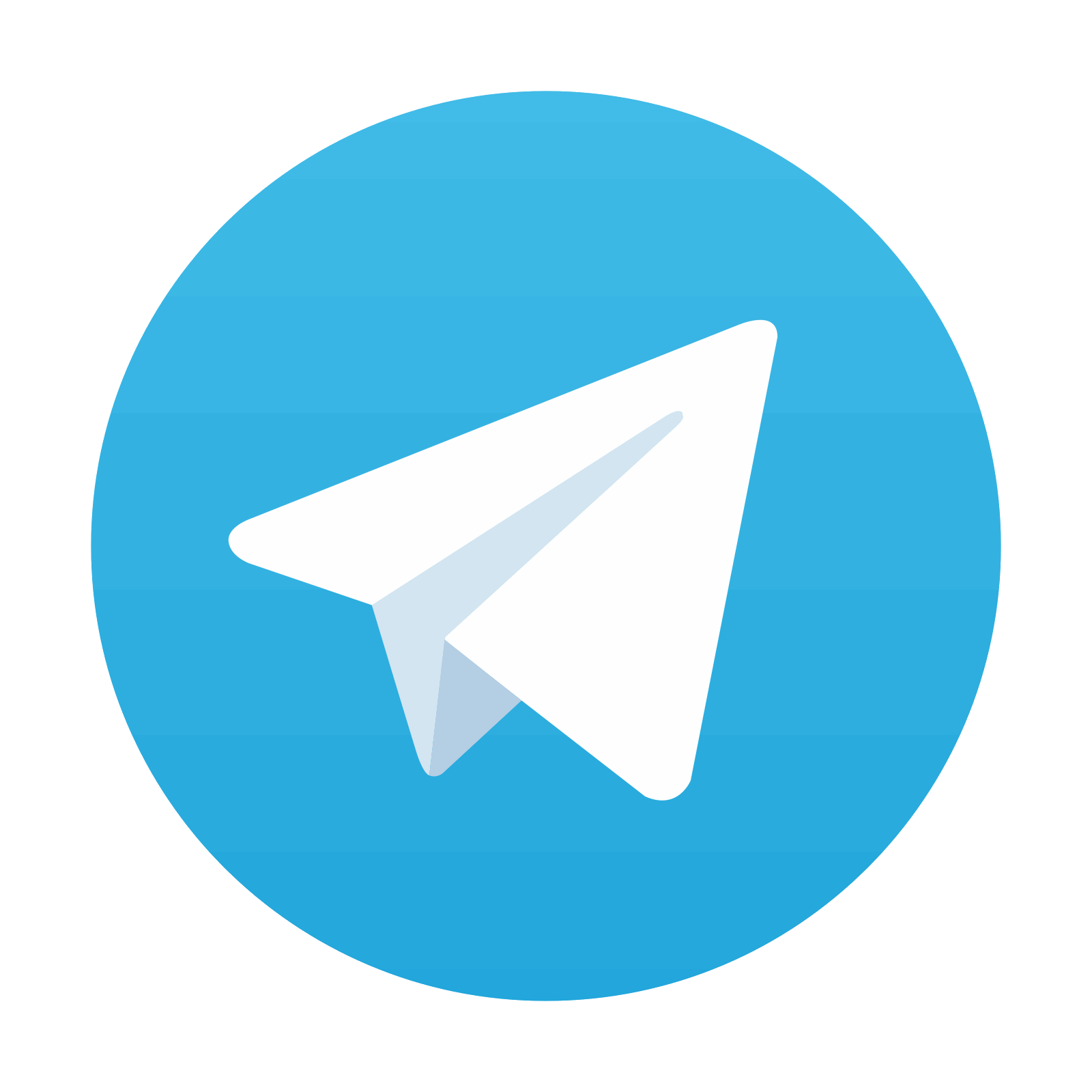
Stay updated, free articles. Join our Telegram channel

Full access? Get Clinical Tree
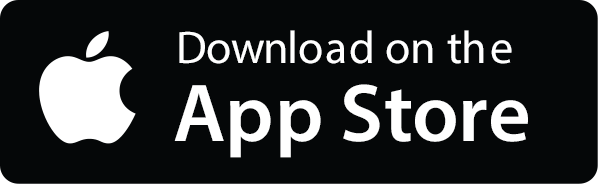
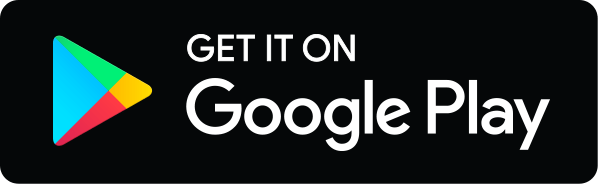