Fig. 9.1
Development of uterine neoplasms in Psmb9/β1i −/− mice. Uterine neoplasms in Psmb9/β1i −/− mice. Photographs of H&E staining and immunohistochemistry (IHC) with anti-ki-67/MIB1 antibody show the characters of uterine neoplasm. Histological examinations of the Psmb9 −/− uterine tumors revealed the common characteristic abnormalities of uterine leiomyosarcomas (LMS). Presentation of these histological data in this manuscript was approved by Prof. Susumu Tonegawa (Picore Inst. and Dept. of Biology, M.I.T., MA)
9.3 Correlation Between Defective PSMB9/β1i Expression and Human Uterine LMS
Several reports suggest that IFN-γ-induced restoration of antigen-processing machinery improves antitumor-specific antigen cytotoxic T-lymphocyte (CTL) recognition in some patients; thus, approaches to activate this pathway may be of benefit to patients with PSMB9/β1i deficiency. Furthermore, it should be demonstrated whether human Ut-LMS shows a weak expression of PSMB9/β1i. The effects of IFN-γ on PSMB9/β1i expression were examined using five human Ut-LMS cell lines [13]. PSMB9/β1i expression were not markedly induced by IFN-γ treatment in human Ut-LMS cell lines, although cervical epithelial adenocarcinoma cell lines and normal human Ut-SM cells underwent strong induction of PSMB9/β1i following IFN-γ treatment [13]. Furthermore, the experiments, performed separately at several medical facilities, revealed a serious loss in the ability to induce PSMB9/β1i expression in human Ut-LMS tissues in comparison with normal myometrium tissues located in the same tissue sections: normal myometrium, total 72 cases; LMA, total 51 cases; bizarre LMA, total three cases; and LMS, total 58 cases [13]. In addition, immunohistochemistry (IHC) showed marked PSMB9/β1i expression in cervical epithelial adenocarcinoma tissues as well as cell lines treated with IFN-γ.
The defect was localized to Janus-activated kinase 1 (JAK1) activation, which acts upstream in the IFN-γ signal pathway since IFN-γ treatment could not strongly induce JAK1 kinase activity in human Ut-LMS cell lines. Sequence analysis demonstrated that the loss of IFN-γ responsiveness in the human Ut-LMS cell line was attributable to the inadequate kinase activity of JAK1 due to a G781E somatic mutation in the ATP-binding region on human JAK1 molecule [13].
9.4 Mutations in IFN-γ Signaling Pathway in Human Uterine LMS Tissues
IFN-γ treatment markedly increased the expression of PSMB9/β1i, a subunit of the proteasome, which alters the proteolytic specificity of proteasomes. After binding of IFN-γ to the type II IFN receptor, JAK1 and JAK2 are activated and phosphorylate the signal transducer and activator of transcription 1 (STAT1) on the tyrosine residue at position 701 (Tyr701) and the serine residue at position 727 (Ser727) [20, 21] (Fig. 9.2). The phosphorylated STAT1 forms homodimers that translocate to the nucleus and bind IFN-γ-activated site (GAS) elements in the promoters of IFN-γ-regulated genes [20, 21] (Fig. 9.2).


Fig. 9.2
Key role of the IFN-γ signaling pathway on PSMB9/β1i expression in human myometrium. After the binding of interferon-γ (IFN-γ) to the type II IFN receptor, Janus-activated kinase 1 (JAK1) and JAK2 are activated and phosphorylate signal transducer and activator of transcription 1 (STAT1) on the tyrosine residue at position 701 (Tyr701). The tyrosine-phosphorylated form of STAT1 forms homodimers that translocate to the nucleus and bind to IFN-γ-activated site (GAS) elements that are present in the promoters of IFN-γ-regulated genes. The IFN-γ-activated JAKs also regulate, through yet unknown intermediates, activation of the catalytic subunit (p110) of phosphatidylinositol 3-kinase (PI3K). PI3K activation ultimately results in downstream activation of protein kinase C-δ (PKC-δ), which in turn regulates phosphorylation of STAT1 on the serine residue at position 727 (Ser727). The phosphorylation of Ser727 is not essential for the translocation of STAT1 to the nucleus or for the binding of STAT1 to targeting DNA, but is required for full transcriptional activation. IFNGR1, IFN-γ receptor subunit 1; IFNGR2, IFN-γ receptor subunit 2
Genetic alterations in tyrosine kinases have previously been firmly implicated in tumorgenesis, but only a few serine/threonine kinases are known to be mutated in human cancers [22–25]. For instance, mice carrying homozygous deletion of Pten alleles developed widespread smooth muscle cell hyperplasia and abdominal leiomyosarcomas [26] and JUN oncogene amplification and overexpression block adipocytic differentiation in highly aggressive sarcomas [27]. Most frequently, leiomyosarcomas (LMS) have appeared in the uterus, retroperitoneum, or extremities, and although histologically indistinguishable, they have different clinical courses and chemotherapeutic responses. The molecular basis for these differences remains unclear. Therefore, the examination of human Ut-LMS tissues (32 human Ut-LMS tissue sections and normal myometrium tissue sections located in the same tissue) was performed to detect somatic (tumor-specific) mutations in the IFN-γ signal cascade. The genetic approach has already addressed that somatic mutations in JAK1 molecule correlate to the initiation of several cancer progressions and other disorders [28–30]. Overall, nearly 37.5% (6/16) of human Ut-LMS tissues had mutations in the catalytic regions, the ATP-binding region or kinase-active site of JAK1. Furthermore, the genetic approach has already revealed that somatic mutations in the PSMB9/β1i molecule or its enhancer region correlate to the initiation of several cancer progressions and other disorders; 31.3% (5/16) of human Ut-LMS tissues had somatic mutations in the Psmb9 promoter region, which is required for transcriptional activation [31–35]. In addition, genetic examination has already demonstrated that somatic mutations in the STAT1 molecule correlate to the initiation of several disorders [36–39]. Nearly 37.5% (6/16) of human Ut-LMS tissues had somatic mutations in the STAT1 intermolecular region. Although the genetic approach has already addressed that marked JAK2 activation causes myelo- and lymphoproliferative disease, no somatic mutation in the ATP-binding region and kinase-active site of JAK2 was detected in human Ut-LMS [40–44]. In a recent report, high-resolution genome-wide array comparative genomic hybridization (CGH) analysis of human Ut-LMS cases gave gene-level information about the amplified and deleted regions that may play a role in the development and progression of human Ut-LMS. Among the most intriguing genes, whose copy number sequence was revealed by CGH, were loss of JAK1 (genome locus 1p31 ~ p32) and PSMB9 (genome locus 6p21.3) [45, 46]. The discovery of these mutational defects in a key cell signaling pathway may be an important development in the pathogenesis of human Ut-LMS.
It is probable that the list of new elements involved in IFN-mediated signal cascade will continue to grow during the next few years, whereas the contributions of known pathways might need to be reevaluated. At present, it seems that the activation of more than one signaling pathway is required for the generation of different biological properties of IFNs, and no signaling cascade alone is sufficient for the generation of any given biological endpoint. For example, the physiological functions of the STAT, NF-κB, and p38 signaling pathways are required for antiviral effects or antitumor effects of IFNs, but activation of these pathways alone is not sufficient to elicit an antiviral or antitumor response [47, 48]. Such a requirement for multiple signaling pathways also seems to be the case for IFN-dependent antiproliferative responses and might reflect the synergistic effects of various signals at the levels of gene transcription and translation; therefore, additional genetic analysis is required to completely elucidate the mutational activation of a key cell signaling pathway in human Ut-LMS.
9.5 Potential Role of Anti-Oncogenic Function by PSMB9/ β1i
The growth of cell lines with JAK1 kinase activity is strongly inhibited by IFN-γ treatment, whereas the growth of JAK1-deficient cell lines is unaffected [49]. Similarly, the cell cycle distribution pattern of freshly explanted tumor cells derived from JAK1-deficient tumors shows no response to IFN-γ treatment [49]. The growth of the original SKN human Ut-LMS cells, which had defective JAK1 activity, was unaffected by IFN-γ treatment (population doubling time (PDT) = 15.2 h) [13]. In contrast, the growth of JAK1-transfected SKN cells, which had strong exogenous JAK1 activity, was prevented by IFN-γ treatment (PDT = 18.1 h). Interestingly, analysis of PSMB9/β1i-transfected SKN cells showed that exogenous PSMB9/β1i expression resulted in cell growth arrest (PDT = 17.9 h) [13]. Conversely, the growth of PSMB9/β1i-transfected SKN cells was unaffected by IFN-γ treatment (PDT = 18.0 h). In SKN-PSMB9/β1i transfectants, there is a correlation between the levels of exogenous PSMB9 expression and the degree of suppression of the transformed phenotype [13, 50]. The physiological function of PSMB9/β1i with revertant-inducing activity on SKN cells has been demonstrated.
Microarray analysis provides insight into the gene expression changes associated with malignant transformation. To investigate whether stable PSMB9/β1i expression contributes to cell growth phenotype in SKN cells, the experiment (using Affymetrix Human GeneChip HG U133 Plus2.0) demonstrated the gene expression profile of SKN cells transfected with plasmid without insert (pCEP9) compared with PSMB9/β1i coding DNA (pCEP9-PSMB9/β1i). Microarray analysis has elucidated that PSMB9 expression dramatically influences the expression pattern of cell cycle regulators, especially anti-oncogenic factor interferon regulatory factor 1 (IRF-1), which directly correlates to progressively worsen with the increasing stage and grade of the tumor [50–52] (Fig. 9.3). In the farther study, we show that PSMB9/β1i may negatively regulate prograssion of human Ut-LMS independently of its role in the proteasome. Moreover, several lines of evidence indicate that although CALPONIN h1 does not directly influence tumorigenesis, it clearly affects PSMB9/β1i-induced cellular morphological changes [51].


Fig. 9.3
Model for the initiation of sarcomagenesis of uterine leiomyosarcoma. The initiation of sarcomagenesis of uterine leiomyosarcoma development is attributed to defect in PSMB9/β1i expression, which results in marked cell proliferation
The downregulation of MHC expression, including the Psmb9/β1i gene, is one of the biological mechanisms that tumor cells use to evade host immune surveillance. Recently, the incidence of IFN-γ unresponsiveness in human tumors was examined in several cancers and revealed that around 33% of each group exhibited a reduction in IFN-γ sensitivity [53]. Nevertheless, PSMB9/β1i expression, rather than providing an escape from immune surveillance, seems to play an important role in the negative regulation of Ut-LMS cell growth. Defective PSMB9/β1i expression is likely to be a risk factor for the development of human Ut-LMS, as it is in PSMB9/β1i-deficient mice.
9.6 Tumor Suppressor and Oncogenic Pathways Involved in Sarcomagenesis
Tumor protein 53 (TP53) anti-oncogenic pathway is one of the most well-characterized signal cascades in tumorigenesis [54]. TP53 gene encodes a transcription factor required for the activation of numerous DNA damage cell cycle checkpoint response and apoptotic factors, and thus its activities are often ablated in many malignant tumors. In addition to the loss of TP53 physiological functions via inherited germ line mutations, the TP53 signaling pathway is commonly disrupted by point mutations in the TP53 gene during sporadic sarcomagenesis [55, 56]. However, even though TP53 gene alterations are widely regarded to have a significant impact on sarcomagenesis, many soft tissue sarcomas retain wild-type TP53 but phenotypically display a loss of TP53 physiological function. These research observations suggest that changes in other components of TP53 signal cascade, such as amplification of MDM2, a negative regulator of TP53 signal pathway, may result in TP53 inactivation [57, 58]. Furthermore, mice and humans with elevated levels of MDM2 due to a high frequency single nucleotide polymorphism in the MDM2 promoter (Mdm2SNP309) are both more susceptible to sarcoma formation [59]. Additionally, deletion or silencing of P19Arf (P14ARF in human), an inhibitor of the MDM2/TP53 axis, often results in the development of soft tissue sarcomas. Together, these findings indicate that while inactivation of the TP53 signal pathway is observed in the vast majority of human soft tissue sarcomas, the mechanisms leading to disruption of the pathway vary greatly.
The retinoblastoma (RB) signal pathway represents a second major anti-oncogenic pathway that is deregulated in many soft tissue sarcomas. Individuals inheriting a germ line RB mutation typically develop cancers of the eye early in life. However, in addition to retinal malignant tumors, these children have a significantly higher propensity to develop soft tissue sarcomas than the general population [60]. While the inheritance of germ line RB alterations increases the risk of soft tissue sarcoma, there are also numerous examples of sporadic sarcomas harboring spontaneous mutations and deletions in RB, particularly osteosarcomas and rhabdomyosarcomas [61]. Furthermore, P16INK4A, a negative regulator of the CDK/CYCLIN complexes that phosphorylate and activate RB, is often deleted in soft tissue sarcomas [62]. These findings may illustrate the importance of RB signaling pathway in sarcomagenesis. Although we previously demonstrated that the abnormal expression of TP53 and Ki-67 and mutations in TP53 molecule were frequently associated with human Ut-LMS, the defective expression of PSMB9/β1i appeared to be more characteristic of human Ut-LMS than these factors [63].
Conclusion
To improve the prognosis of human Ut-LMS, research experiments were performed to identify the key role of pro- or anti-oncogenic factors that have an important function in their pathogenesis and that could serve as molecular targets for tumor treatment. For this purpose, several research facilities conducted a microarray procedure between human Ut-LMS and normal myometrium and showed that several known pro-oncogenic factors, such as brain-specific polypeptide PEP-19 and c-kit, may be associated with the pathogenesis of human Ut-LMS [64–66]. However, in terms of the tumorgenesis of human Ut-LMS, merely comparing the expression of potential pro-oncogenic factors between normal and malignant tissues is not sufficient because the results obtained may be the consequence of malignant transformation and, therefore, not necessarily the cause.
For almost all types of cancer studied to date, it seems as if the transition from a normal, healthy cell to a malignant tumor cell is a stepwise progression that requires genetic changes in several different oncogenes and tumor suppressors. In order to generate a malignant cell, a series of somatic mutations must occur in the same cell. Since the likelihood of any gene becoming mutated is very low, it stands to reason that the chance of several different mutations occurring in the same cell is highly unlikely. For this reason, cells in an elderly body have had more time to accumulate the changes needed to form cancer cells, whereas those in a child are much less likely to have acquired the requisite genetic changes. Importantly, clinical experiments have revealed loss of the ability to induce PSMB9/β1i expression in human Ut-LMS tissues in comparison with normal myometrium tissues. The discovery of somatic mutational defects in the IFN-γ-signaling pathway may be important for the initial development of human Ut-LMS. It is noteworthy that stable PSMB9/β1i expression contributes to cell proliferation, which directly correlates to the progressive deterioration with increasing stage and grade of the tumor. Recent advances in our understanding of the biology of human Ut-LMS have concentrated on the impaired IFN-γ signaling pathway. It is clear that mutations in key regulatory genes (tumor suppressors and proto-oncogenes) alter the behavior of cells and can potentially lead to the unregulated growth seen in malignant tumor. Therefore, continued improvement of our knowledge of the molecular biology of murine and human Ut-LMS may ultimately lead to novel therapies and improved outcome.
Acknowledgments
We sincerely appreciate the generous donation of PSMB9/β1i-deficient breeding mice and technical comments by Dr. Luc Van Kaer and Dr. Susumu Tonegawa, Massachusetts Institute of Technology (M.I.T.). We thank Isamu Ishiwata for his generous gift of the human Ut-LMS cell lines. We appreciate the technical assistance of the research staff at Harvard Medical School (H.M.S.). We are grateful to Dr. Tamotsu Sudo and Dr. Ryuichiro Nishimura, Hyogo Cancer Center for Adults, for their generous assistance with immunohistochemistry (IHC) analysis and helpful discussion. This work was supported by grants from the Ministry of Education, Culture, Science and Technology, the Japan Science and Technology Agency, the Foundation for the Promotion of Cancer Research, Kanzawa Medical Research Foundation, and The Ichiro Kanehara Foundation.
Disclosure: The authors report no conflicts of interest.
References
1.
Zaloudek C, Hendrickson MR. In: Kurman RJ, editor. Mesenchymal tumors of the uterus: Blaustein’s pathology of the female genital tract. 5th ed. New York: Springer; 2002. p. 561–78.
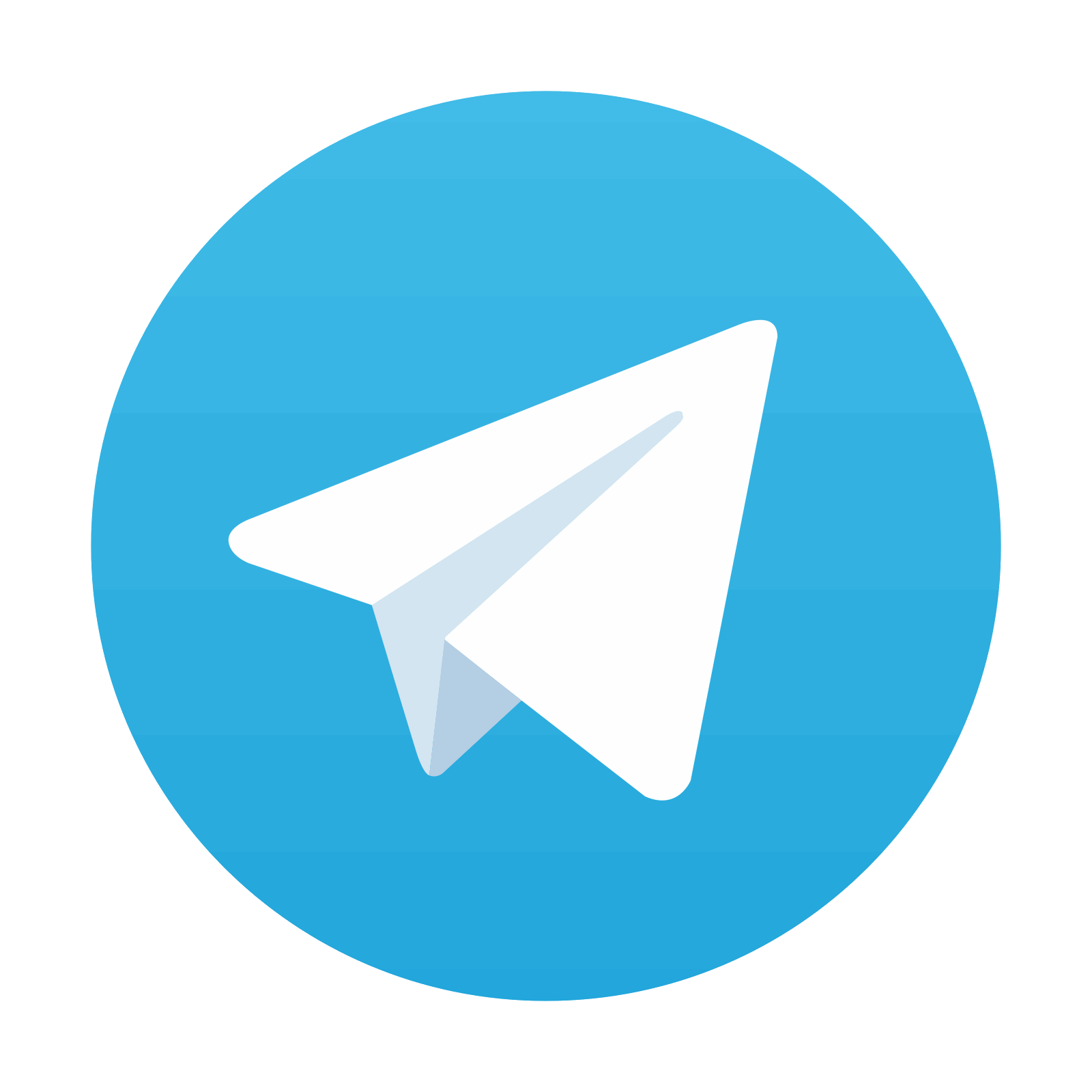
Stay updated, free articles. Join our Telegram channel

Full access? Get Clinical Tree
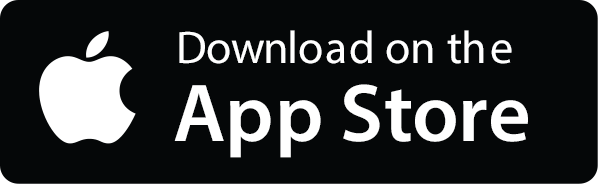
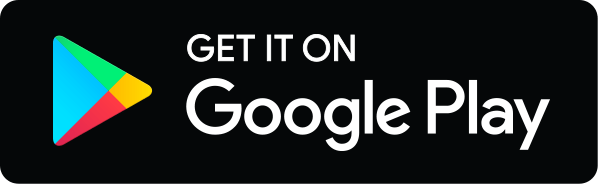