Fig. 3.1
Schematic representation of the cell cycle control mediated by TP53 and RB1
3.1.2.2 The RB1 Pathway
The cell cycle control pathway that includes the retinoblastoma gene, RB1, is frequently altered in human tumors, especially in osteosarcomas [7] (Fig. 3.1). As described later, the loss of genetic material affecting the long arm of chromosome 13 (13q14) is a frequent genetic event in primary osteosarcomas, and this fact was interpreted to indicate the presence of a tumor suppressor gene at this chromosomal location. This suppressor has turned out to be RB1 [8].
The RB1 encodes a tumor suppressor protein, pRB, which regulates cell cycle progression (G1/S) by inhibiting E2F and other transcription factors of the same family. Cell cycle regulation involves cyclins, cyclin-dependent kinases (CDKs) and cyclin-dependent kinase inhibitors (CDKIs or CDKNs), and all of these genes and proteins, as key parts of the pRB pathway for cell cycle control, are often mutated or altered in osteosarcoma; examples include CDK4 amplification (10 % of cases), amplification of PRIM1 (40 %), deletion of 9p21, where CDKN2A/p16INK4, p14ARF and CDKN2B/p15INK4B are located (in about 5–20 %) [2].
About 20–70 % of osteosarcomas have a hemizygous deletion affecting the RB1 gene [9, 10]. About 30 % have structural rearrangements 18 and about 10 % have point mutations of the gene [11]. The presence of alterations at the RB1 locus can be considered as an early marker of malignancy and of unfavorable prognosis and, in addition, RB1 alterations are more frequently encountered in high-grade than in low-grade osteosarcomas [12, 13]. However, as with many of the molecular markers of this specific tumor, this prognostic association is not always found [14].
3.1.3 Growth Factors
1.
Members of the WNT Family (Wingless–Type). The WNT signaling pathway controls normal bone formation during embryogenesis and bone homeostasis in the adult. Various publications describe research into this pathway in the context of the osteosarcoma model. Recent data suggest that the WNT pathway may have a paracrine and autocrine effect involved in the metastatic potential of osteosarcoma, [15, 16] although these data remain to be reproduced.
2.
Her–2/neu (Epidermal Growth Factor Receptor). Overexpression of this tyrosine kinase is considered an indicator of poor prognosis in various types of carcinoma, since it is related with tumor growth and the metastatic process. Her-2 is expressed in a proportion of osteosarcoma cases but, despite the implied involvement of Her-2 in osteosarcoma, neither normal nor pathological levels of Her-2 expression have been unequivocally established and consequently the prognostic value of Her-2 is still being researched; different reports present contradictory results. However, Her-2 remains an attractive therapeutic target. To date, at least one study of trastuzumab for the treatment of osteosarcoma has been completed, and although the findings suggest that trastuzumab can be safely used with chemotherapy in osteosarcoma, its therapeutic benefit remains uncertain. The authors conclude that definitive assessment of trastuzumab’s potential role in treating osteosarcoma would require a randomized study of patients with Her-2-positive disease [17, 18].
There are many other genes of interest under investigation. One of these is ezrin, which is a prototypical member of the ERM (Ezrin/Radixin/Moesin) protein family. The encoded protein is involved in cell-to-cell interaction, in connecting the actin cytoskeleton and the plasma membrane and in signal transduction. Overexpression of ezrin promotes metastasis, probably by allowing metastatic cells to overcome the controls and limitations imposed on them during the metastatic cascade and by facilitating the cells’ interaction with the environment of the secondary, metastatic, site: metastatic cells must rapidly adapt to their new environment in order to survive, and it seems that, through participation in processes such as the translation of new proteins and the generation of ATP, ezrin expression could be related to this cellular adaption [19].
The fact that the timing of the peak incidence of osteosarcoma overlaps with that of the pubertal growth spurt may indicate that insulin-like growth factor-I (IGF–I) and its receptor play a role in the pathogenesis of this disease. The IGF-I growth factor acts as a mitogen on both murine and human osteosarcoma cells, and osteosarcoma cell lines are dependent on IGF-I for in vitro growth. It has been hypothesized that even in osteosarcoma patients whose levels of IGF-I and its binding protein (IGFBP3) are not increased, other members of the IGF-I axis could be involved in the development and progression of osteosarcoma [20, 21].
3.1.4 Angiogenesis
The vascular endothelial growth factor (VEGF) stimulates microvascular growth and has a clear role in the development of certain tumors (such as those of the breast and colon) by increasing the supply of nutrients and blood to them. The use of anti-angiogenic agents (inhibitors of the VEGF pathway) is controversial in the case of osteosarcoma, and reports show contradictory results [22, 23]. Therefore, the utility of this and other related molecules (for example, pigment epithelium derived factor [PEDF]) as therapeutic targets in osteosarcoma have yet to be determined, as have the synergic effect of these molecules with chemotherapy. Studies are already in progress [24].
3.1.5 Matrix Metalloproteinases
Matrix metalloproteinases (MMPs) are enzymes that are physiologically involved in tissue remodeling and angiogenesis. Excessive production of MMPs, whether as a result of increased transcription of coding genes or as a result of a lack of inhibitors, is important in the process of invasion and metastasis. MMP9, a member of this family, seems to have a prominent role in bone remodeling diseases like osteosarcoma, and several publications have shown that MMP9 overexpression in osteosarcoma is a factor indicating poor prognosis (with an increase in metastatic potential and reduced overall 5-year survival) [25, 26]. MMP9 can be repressed by a variety of molecules, a fact that makes it an interesting target for attempts to decrease the invasive potential of tumor cells. Indeed, this effect has been demonstrated in animal models and cultured cells.
3.1.6 P Glycoprotein
P glycoprotein (P-gp), which is coded by the multidrug resistance 1 (MDR1) gene, is a membrane molecule involved in drug transport. For more than a decade, P-gp overexpression has been known to be a negative prognostic factor in osteosarcoma [27]. There are various reasons for this, one of them being that P-gp is what makes osteosarcoma cells resistant to doxorubicin, a prime cytostatic drug in the standard chemotherapy for this pediatric tumor [28]. Some authors have identified a link between P-gp and p53 overexpression. As described above, the p53 molecular pathway is another pathway frequently altered in osteosarcomas. According to this model, patients whose tumors have co-overexpression of P-gp and p53 would have significantly reduced survival and a more unfavorable Enneking stage [29].
3.1.7 Chromosomal Alterations
Conventional karyotype analyses show that, as a general rule, osteosarcomas have complex altered karyotypes with multiple numerical and structural aberrations; this is mostly due to an elevated rate of chromosomal instability (CIN) involved in the pathogenesis of osteosarcoma. The most frequently encountered alterations in primary tumors (as opposed to cell lines) are the duplication or gain of chromosome arms 1p, 1q, 6p, 8q, and 17p; and the loss of chromosomes or chromosome arms 3q, 6q, 9, 10, 13 (RB1 locus), 17p (TP53 locus), and 18q [30]. In general, regions frequently found to be deleted or lost are suspected to contain tumor suppressor genes, while genomic regions that are duplicated, gained or amplified are suspected to contain oncogenes [2, 31].
Metaphase comparative genomic hybridization (CGH) has proved a useful, high-resolution tool to unveil and characterize complex karyotypes, and studies based on CGH have identified high copy number regions or amplifications at 8q12–q21.3, 8q22–q23 (MYC gene) and at 17p11.2–17p12 [31]. The chromosomal regions that are more frequently gained or lost have been carefully identified and reviewed [32–34]. Work by Ozaki and colleagues establishes that the gain or loss of some of these regions, either as isolated aberrations or as specific combinations, might have prognostic value [35].
3.1.8 New Identification Studies, GWAS
Recently, new approaches such as genome-wide association studies (GWAS) have been applied to identify genes potentially involved in osteosarcoma. An international, multi-institutional collaborative study of osteosarcoma performed a GWAS including 941 osteosarcoma cases and 3291 cancer-free adult controls. The study found two regions of susceptibility: 2p25.2, an apparent desert region, and 6p21.3, containing the metabotropic glutamate receptor 4 (GRM4) gene. Although further studies are needed, GRM4 seems to be a significant gene in osteosarcoma and even a plausible candidate gene for the disease, and it is expressed in osteoblasts and osteoclasts. GRM4 has been implicated in intracellular signaling by inhibition of the cAMP cascade. In mice, a gene with similar function (Prkar1α) is an osteosarcoma tumor suppressor gene involved in tumorigenesis [36].
3.2 Ewing’s Sarcoma Tumors
3.2.1 Introduction
Tumors within the Ewing’s sarcoma family (EFTs) constitute the second most frequent type of bone-/soft- tissue sarcoma in children and adolescents [37, 38]. The family comprises classical Ewing’s sarcoma, peripheral primitive neuroectodermal tumors, and Askin’s tumor, all of which are highly aggressive and frequently metastatic [39]. Tumors often appear in tubular bones of the appendicular skeleton (58 %), although they also arise in the axial skeleton (33 %) and at extraosseous sites [40]. Histologically, Ewing’s tumors are characterized by the presence of small round cells with prominent and regular nuclei containing inconspicuous nucleoli, indistinct cytoplasm [38] and various degrees of neural and endothelial differentiation [41].
Until the advent of differential molecular techniques, an unambiguous diagnosis required experienced pathological assessment. None of the markers used in conventional immunohistochemistry showed complete specificity. The transmembrane glycoprotein MIC2/CD99, the most specific marker so far, is expressed in more than 98 % of EFTs [42].
Other tumors, including rhabdomyosarcoma and lymphoblastic lymphoma, also have immunohistochemical markers. Depending on the degree of neuroectodermal differentiation, EFTs may express neural markers, including S-100 synaptophysin, neural-specific enolase, CD57 and various neurofilaments [43]. Currently, the best tools for diagnosis are fluorescence in situ hybridization and RT-PCR with a combination of primers targeting the underlying chromosomal translocations [44].
Much of the progress over the last few decades has concerned improved pathological definition and staging [45]. Despite a parallel improvement in treatment by multi-modal combination of surgery with chemo- and radio- therapy, the 5-year survival rate has remained close to 50 % for patients with primary tumors [46] and only 25 % for patients with lung metastasis; the prognosis for patients with bone or bone marrow metastasis is even worse [47]. The tumor also exhibits a strong tendency to metastasize through hematogenous spread to the lungs and frequently to the skeleton. In addition to the stage, location and size of the tumor, metastasis is a reliable indicator of poor prognosis.
3.2.2 Molecular Biology
The demonstration that fusion proteins were the “culprits” of the transforming events giving rise to hematological malignancies provided a strong rationale for research into the role of fusion proteins in other tumors. EFTs belong to a growing family of sarcomas characterized by specific reciprocal chromosomal translocations, which generate fusion genes: the neoplasm has a relatively simple cytogenetic background [48]. At present, more than 15 different fusion proteins have been identified in EFTs. Chromosomal translocation t(11;22) q(24;q12) produces gene fusions between the amino terminus domain of EWS and the C-terminal region of a member belonging to the ETS family of transcription factors. Fusions resulting from this translocation give rise to transcription factors that function in an aberrant manner and can drive transformation in a permissive cellular context.
In 85 % of Ewing’s tumors, it is the Friend Leukemia Integration 1 transcription factor (FLI-1) that is the EWS partner that accounts for the different fusion subtypes observed [49]. Depending on the juxtaposed exons assembled by EWS and FLI-1 breakpoints, several subtypes have been described. Most tumors contain EWS/FLI-1 fusion types 1 (60 %) and 2 (25 %), which have been associated with different clinical features and prognosis [50, 51]
The FLI-1 gene displays a restricted pattern of expression: it is expressed mainly in hematopoietic cells, and to a lesser degree in heart, lung and ovaries [52]. During development, FLI-1 is also expressed in cells of neural crest-derived mesenchymal lineage and endothelial cells, which is consistent with its known role in angiogenesis and hematopoiesis [53].
In contrast to FLI-1, the EWS gene encodes a ubiquitously expressed protein that belongs to the TET family of RNA binding proteins [49, 54]. The TET family also includes TAFII68 (or TAF15) [55, 56] and FUS (or TLS), [57] both of which share similar structural domains with EWS and have been found to form gene fusions with non-ETS transcription factors, giving rise to non-Ewing sarcomas such as myxoid liposarcomas, [58] myxoid chondrosarcomas [59] and desmoplastic small round-cell tumors, [60] with different histopathological features. EWS contains three arginine–glycine–glycine (RGG) rich motifs that participate in RNA synthesis and processing through interaction with proteins of the basal transcription machinery; these proteins include TFIID, RNA polymerase II [61, 62] and coactivators such as CBP/p300 [55, 54]. In addition, proteins in the TET family interact with splicing proteins. Indeed, EWS/FLI-1 has been shown to bind with the splicing factor U1C and subsequently to modulate splicing activity [62–64]. EWS interaction with other proteins has also been described, for example, with BARD1, although the relevance, if any, of this to tumorigenesis has not been elucidated [65].
Most research into EWS has focused on its function as a transcription factor. The amino terminal domain of EWS contains a glutamine-rich N-terminal region containing a potent transcriptional activation domain [66, 67] that, when fused to the DNA-binding domain of FLI-1, generates an aberrant but active transcription factor capable of specifically binding DNA [68]. Since the majority of fusion subtypes do not encompass the RGG domain, there has been less research into the effects of EWS–ETS fusions on RNA synthesis. All members of the ETS family of transcription factors are characterized by a common DNA-binding domain. Fusions of EWS with other ETS family members have been described, [69] including fusions with ERG (in 10 % of Ewing’s tumors) [70] and with other, more rare, partners such as ETV1, [71] ETV4 [72] and FEV [73]. The fact that different combinations of EWS/ETS give rise to Ewing’s tumors of similar histopathology suggests that the potent transactivation domain of EWS, through interaction with other unknown proteins, is critical for transformation.
Complementary to this view, the DNA binding domains of ETS proteins are highly homologous and all recognize targets containing a similar core sequence. Therefore, despite their differences, all EWS–ETS fusion proteins can be expected to act in a similar manner that involves disruption of a tightly regulated pattern of gene transcription, resulting ultimately in the formation of Ewing’s tumors.
3.2.3 The Oncoprotein EWS/FLI-1 as a Paradigm
The discovery of EWS/FLI-1 underscores the attractiveness of an approach to research based on seeking to reveal common mechanisms in many tumors arising from specific translocations. Depending on the cellular background, EWS/FLI-1 induces a variety of responses that include transformation, senescence, differentiation, and cell lineage commitment. The presence of neural markers in Ewing’s tumor cells and the diverse sites of tumor origin have frequently led to the assumption or hypothesis that EFTs develop out of a cell type that possesses the potential to differentiate into multiple lineages.
In vitro experiments revealed that the chimeric EWS/FLI-1 acts as a potent repressor of normal cell fate. In murine primary marrow-derived stromal cells, EWS/FLI-1 represses osteogenic and adipogenic programs [74, 75]. Similarly, myogenic differentiation was suppressed by the chimeric protein in a murine multipotent mesenchymal cell line [74]. In contrast, in other cellular backgrounds, EWS/FLI-1 dictates cell lineage commitment by redirecting cells towards a neural-like phenotype [76]. Differentiation towards the neuroectodermal phenotype typical of Ewing’s tumors, with the acquisition of a small round cell morphology, has been obtained in a fibroblastic cell line [77]. Similarly, in neuroblastoma, Hela, and rhabdomyosarcoma cell lines, the forced expression of EWS-FLI1 resulted in the acquisition of neural phenotypic traits [78]. Consistent with the role of FLI-1 during development, in a separate rhabdomyosarcoma cell line, the expression of neural crest phenotypic markers was induced by EWS/FLI-1 [79].
In addition to repression of normal cell fate, EWS/FLI-1 is thought to induce cell specific oncogenesis in a transformation-permissive cellular background. However, many cell lines, including Rat-1 fibroblasts, Ncm1, CTR, and the NIH 3 T3-derived cell line YAL-7, have been found to be refractory to transformation [80]. Single-step oncogenesis has been reported in murine primary cells [66, 77, 81, 82]. This finding can be attributed to a better transformation potential of rodent cells compared to human cells [83, 84]. In a study by Castillero-Trejo et al., tumorogenicity increased with cell passage in culture and other secondary events, including p53 deletion [81]. Murine bone-derived cells expressing EWS/FLI-1 showed formation of sarcomatous tumors in syngeneic mice. Riggi et al. reported that a single event of transduction with EWS/FLI-1 was sufficient to reconstitute the hallmarks of Ewing sarcoma genesis in a murine model [82]. In contrast, Riggi et al. were unable to reproduce the corresponding findings in primary human cells [85]. Studies of a human model have recently provided strong experimental evidence that the originating cell type is of mesenchymal lineage [86].
Why is it easier to induce tumorigenesis in mouse cells than in human cells? In the mouse, background tumor suppressor pathways, including p16/p19 and p53, may be overcome by unknown factors. In human cells, however, additional mutations may be required to circumvent the strong tumor suppressor program. Indeed, other cytogenetic events [87] and additional mutations have been found in 20–30 % [88–90] of human Ewing’s tumors. An alternative suggestion recently put forward is that the cancer-initiating cells that are able to sustain tumor growth in vivo in humans may actually be deregulated progenitor cells present in adult tissues [91, 92]. It is possible that the target for the transformation event driven by EWS/FLI-1 is an as yet unidentified progenitor cell.
3.2.4 Target Genes
One of the main goals in the study of the genesis of Ewing sarcoma has been to identify downstream target genes regulated by EWS/FLI-1. Global transcriptome analysis in combination with other techniques has elucidated several genes involved in the genetic program driven by the fusion protein. The genes found are involved in neural differentiation, cell proliferation and anti-apoptotic functions. However, because the studies differ significantly in terms of cellular model, it has been difficult to discern which EWS/FLI-1-responsive genes are associated with the initiation and maintenance of tumors.
The most compelling identified target of EWS/FLI-1 is the gene coding for the homeodomain protein NKX2.2, [93] which is transcriptional repressor involved in neural cell differentiation. Induction of NKX2.2 is necessary for oncogenic transformation and represents a potential Ewing diagnostic marker. The protein’s strong repressive function is mediated by a HDAC-dependent mechanism [93, 94].
Id2, a helix–loop–helix transcription factor without the DNA-binding domain, has been found to be upregulated by EWS/FLI-1 in Ewing’s tumors. Through interaction with a variety of cell cycle proteins including p21 and Rb tumor suppressors, [95, 96] Id2 is able to promote cell proliferation. Other potential target genes transcriptionally upregulated by EWS/FLI-1 include PDGF-C, [97] which is expressed in more than 60 % of tumors, CCND1 [98, 99] and c-Myc [100, 101].
hTERT, the catalytic subunit of telomerase and one of the hallmarks of many Ewing’s tumors, has been found to be upregulated in approximately 80 % of Ewing’s samples. The upregulation is an indirect effect of EWS/FLI-1 [102] through the recruitment of an unknown ancillary protein. Similarly, key tumor suppressors such as p57, [100] p21 [103] and TGFBRII [104, 105] were found to be downregulated in Ewing’s tumors. Interestingly, IGFBP-3 is a direct target of the fusion protein: EWS/FLI-1 binds to the IGFBP-3 promoter both in vitro and in vivo, [106] and the consequent repression leads to increased Akt activity and decreased apoptotic activity. Similarly, the IGF-1/IGF-1R axis, which is frequently required for Ewing’s tumor cell growth, promotes cell survival through the Akt pathway [107, 108]. Different pharmacological strategies targeting IGF1R are currently being explored for the treatment of Ewing’s tumors [109, 110].
Recently, the combination of the techniques of transcriptome analysis with high throughput chromatin immunoprecipitation analysis has validated previous research on EWS/FLI-1 by identifying previously reported genes (NKX2.2, ID2 and CCND1) and has also found additional relevant targets, including NROB1 [111–113] and GAS1. The role of these targets in Ewing sarcomagenesis is yet to be determined.
The complexity of the EWS/FLI-1 -induced program is underscored by the disruption of gene regulatory circuits as a direct result of induction or repression of enhancers: oncogenes are activated by a remodeling of the patterns of chromatin. Such oncogenes represent novel potential therapeutic targets, an example being the kinase VRK1 [114]. EWS/FLI-1 has also been shown to regulate alternative splicing [115] and to be itself regulated by a long piece of non-coding RNA: RNA-277 (Ewing sarcoma-associated transcript 1 [EWSAT1]) [116].
3.2.5 Future Directions
The experimental platforms developed in EFT research hold the potential to resolve many big questions regarding the development of both EFTs and a variety of other sarcomas. Despite the remarkable progress over the last two decades, there are still several areas that have not been rigorously addressed. Amongst these are the clear definition of the permissive cell and the specific time and micro-environment required for transformation. Similarly, the critical molecular events driven by the chimeric EWS/FLI-1 protein to initiate and maintain Ewing’s tumors remain to be systematically dissected in an appropriate model. To this end, an animal model faithfully reproducing the spatiotemporal development of Ewing’s sarcomas would be an invaluable tool. Only with precise knowledge at the cellular and molecular levels can we expect to elucidate the critical target gene or genes of the EWS/FLI-1 protein.
References
1.
Clark JC, Dass CR, Choong PF. A review of clinical and molecular prognostic factors in osteosarcoma. J Cancer Res Clin Oncol. 2008;134:281–97.PubMed
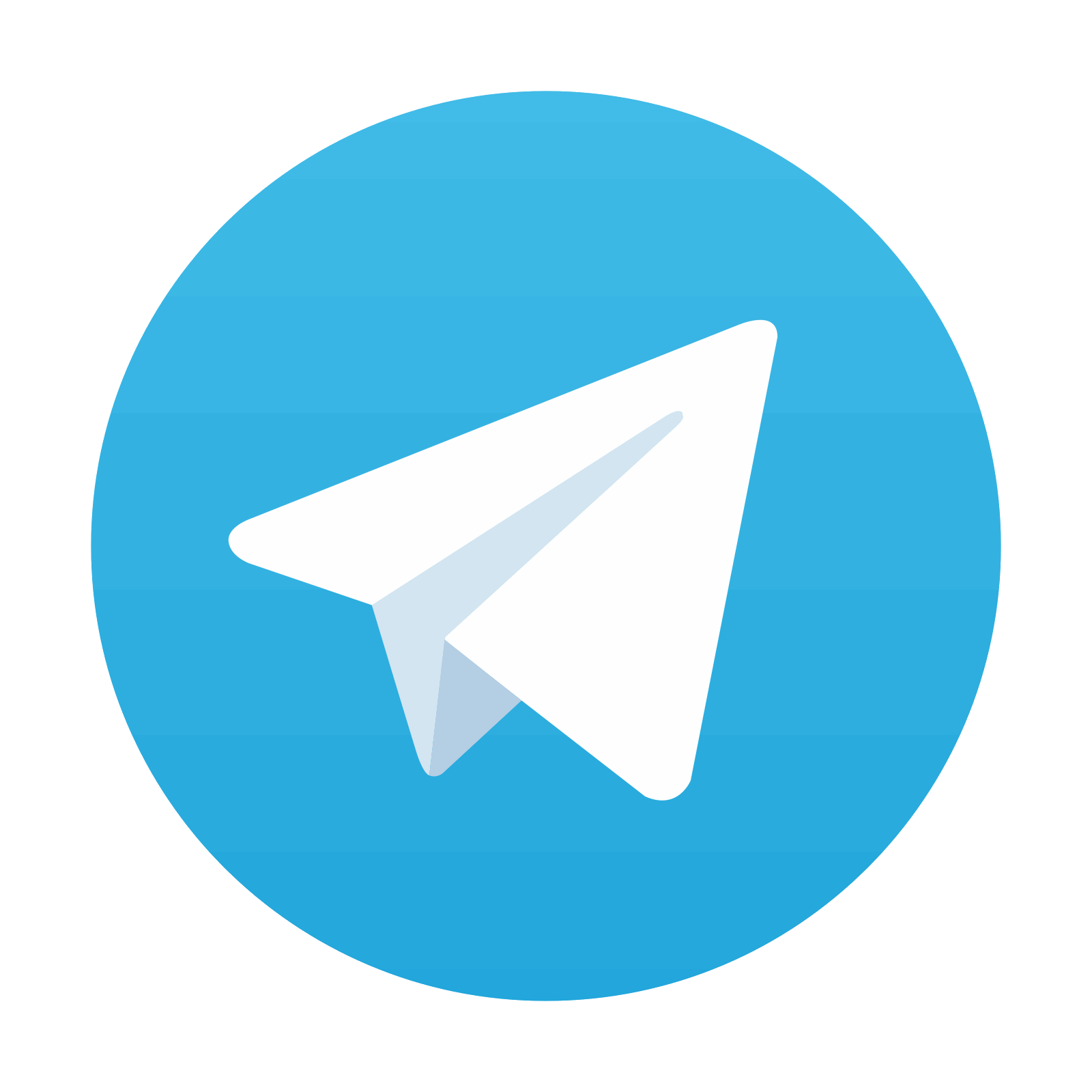
Stay updated, free articles. Join our Telegram channel

Full access? Get Clinical Tree
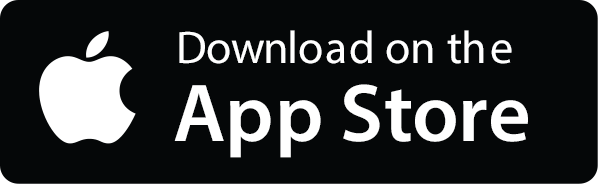
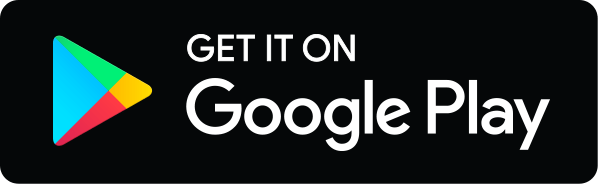