CHAPTER 31 Menstruation and menstrual disorders
Introduction
Menstrual abnormality is a common reason why women present at gynaecological outpatient clinics, and heavy menstrual bleeding (HMB) is one of the most common causes of iron-deficiency anaemia in Western women (Cohen and Gibor 1980). The average woman now experiences approximately 400 cycles, in contrast to the 40 or so she would have experienced 50 years ago. This is mainly due to smaller family size, availability of effective contraception, occurrence of an earlier menarche and later onset of menopause (Higham 1994). In addition to having an increased number of cycles, a significant number of women now work outside home, where disruption of work due to episodes of flooding is even less tolerable. Consequently, disorders of menstruation have become a significant public health issue.
Menstruation
At the end of the ovarian cycle, a major portion of the endometrium in primates undergoes periodic necrosis and sloughing associated with blood loss. Hence, the endometrium is a site of recurrent physiological injury and repair (Critchley et al 2001). During menstruation, the gonadal steroids reach their lowest levels. The nature of the supportive effect on the endometrium is unknown, although possible mechanisms will be discussed later.
Anatomy of the uterus
The wall of the uterus consists of three layers: the serous coat, the myometrium and the endometrium. The serous coat is firmly adherent to the myometrium, which consists of smooth muscle fibres, the main branches of the blood vessels, and the nerves of the uterus and connective tissue. The endometrium consists principally of glandular and stromal cells, although its structure does vary spatially within the uterus and temporally with the stage of the menstrual cycle. The human endometrium is a dynamic tissue that, in response to the prevailing steroid environment of sequential oestrogen and progesterone exposure, undergoes well-characterized cycles of proliferation, differentiation and tissue breakdown on a monthly basis (Jabbour et al 2006).
Blood supply of the uterus
The blood supply of the uterus (Figure 31.1) is via the uterine artery, a branch of the anterior division of the internal iliac artery. It passes medially across the pelvic floor above the ureter, reaching the uterus at the supravaginal part of the cervix. Giving a branch to the cervix and vagina, the vessel turns upwards between the leaves of the broad ligament to run alongside the uterus as far as the entrance of the fallopian tube, where it anastomoses with the tubal branch of the ovarian artery. In its course, it gives off branches that penetrate the walls of the uterus. Within the myometrium, the uterine and ovarian arteries form the arcuate arteries. These, in turn, give rise to the radial arteries which, after passing through the endometrial–myometrial junction, branch into the basal arterioles, supplying the basal endometrium, and the spiral arterioles, supplying the superficial layer of the endometrium. Spiral arterioles are end arterioles and are only present in species that menstruate. Each supplies an area of 4–9 mm2. Branching of the spiral arterioles occurs throughout the superficial layer of the endometrium. Just below the surface epithelium, they break up into a prominent subepithelial plexus that drains into venous sinuses.
The endometrial vasculature is unlike any other vascular bed owing to its cyclic remodelling and regression during the menstrual cycle. These vessels are sensitive to changes in gonadal steroid levels, and at menstruation, the capillaries are shed with the glands and stroma (Rogers et al 1998). A recent study confirmed that crucial structural components in the endometrium during the menstrual process are the component blood vessels and the dynamic population of leukocytes that influx at this time (Jabbour et al 2006).
Histology of the uterus
The endometrium
In the uterus, the superficial endometrial layer is characterized by rapid proliferation during the follicular phase of the cycle, followed by secretory transformation of the glandular epithelium, predecidualization of the stromal compartment and influx of uterine natural killer cells in the luteal phase of the cycle (King et al 1998).
During the proliferative phase, the short, straight epithelial glands elongate and become tortuous (Figure 31.2A). Changes occur in the position of the nuclei and the number of mitoses. During the secretory phase, the glands increase in diameter and tortuosity, and vacuoles appear in the cellular cytoplasm (Figure 31.2B). The tissue also becomes markedly oedematous.
These morphological events are controlled by highly coordinated activation of certain gene sets essential for the regulation of uterine function. For instance, after ovulation, the sequential expression of progesterone-dependent genes defines a limited period of uterine receptivity or, in the absence of pregnancy, maintains vascular integrity prior to menstruation (Tabibzadeh and Babaknia 1995, Lockwood et al 1998). In addition, recent application of knowledge from the human genome, utilizing microarray technologies, has allowed several groups to contribute to a rapidly expanding literature on gene profiles during the ‘putative window’ of implantation (Carson et al 2002, Kao et al 2002, Riesewijk et al 2003).
The microvascular blood supply of the endometrium undergoes the unique process of benign angiogenesis, and is under the control of ovarian steroids during reproductive life. Following cessation of menstruation, they are simple in form, extending just into the endometrium. The secretory phase is characterized by the growth of arterioles. In the late secretory phase, coiling occurs due to proliferation and extension of the arterioles (Figure 31.2C). With the fall in steroid concentrations, menstrual shedding of the functional layer of the endometrium occurs (Figure 31.2D). Dramatic changes occur in the spiral arterioles at menstruation. These changes were described by Markee (1948) after experiments involving the transplantation of endometrium into the anterior chamber of the eye of the Rhesus monkey. This work was the cornerstone of current concepts of menstruation. On observing the bleeding process, Markee suggested that the arteriolar coiling caused constriction of the vessel lumen with vascular stasis and leukocytic infiltration. Approximately 24 h premenstrually, intense vasoconstriction led to ischaemic damage, followed by vasodilatation with haemorrhage from both arterial and venous vessels: 75% of the loss was arteriolar, 15% was venous and 10% was diapedesis of erythrocytes (Markee 1940).
The myometrium
The human myometrium is structurally and functionally polarized during the reproductive years. Compared with the outer myometrium, the subendometrial layer or junctional zone (JZ) is characterized by higher cell density, lower cellular nuclear:cytoplasmic ratios and the expression of different extracellular matrix (ECM) components (Brosens et al 1998, Campbell et al 1998). Additionally, evidence from 31P nuclear magnetic resonance spectroscopic studies has demonstrated biochemical heterogeneity between both myometrial layers (Xu et al 1997).
The differentiation of the myometrium into two distinct layers is strictly under ovarian hormonal control. In premenarchal girls and postmenopausal women, the zonal anatomy is often indistinct. Ovarian suppression with gonadotrophin-releasing hormone (GnRH) analogues leads to a magnetic resonance imaging (MRI) appearance of the uterus mimicking that of postmenopausal women, whilst hormone replacement therapy in postmenopausal women results in the reappearance of myometrial zonal anatomy (Demas et al 1985).
Further evidence for hormone responsiveness of the JZ is provided by the work of Wiczyk et al (1988), who demonstrated changes in JZ thickness throughout the menstrual cycle in conjunction with endometrial thickness.
Mechanisms of Normal Menstruation and Control of Blood Loss
Withdrawal of progesterone from an oestrogen-primed endometrium results in menstrual shedding. Shedding arises because of the induction of matrix metalloproteinases (MMPs) from the endometrium, in particular MMPs 1, 2 and 9 (Jeziorska et al 1996, Marbaix et al 1996). This arises through a mechanism of upregulation of transforming growth factor-beta (TGF-β) (Bruner et al 1995). The blood vessels are thus denuded of their support. Spiral arterioles and venules are cleaved at the level of the JZ between the endometrial functionalis and basalis, with subsequent bleeding.
Factors involved in the control of menstrual blood loss are:
Derangement of any of these mechanisms is likely to lead to excessive menstrual loss.
Haemostasis
Coagulation occurs in the distal endometrium, and platelets found within the endometrial cavity are deactivated and do not respond to collagen, as they would elsewhere. Once clinical bleeding and tissue shedding have started, haemostatic plug formation occurs, but less rapidly and less completely than is observed in human skin wounds (Christiaens et al 1982). Certain haemorrhagic conditions (e.g. thrombocytopenic purpura) are associated with increased incidence of HMB, suggesting that abnormalities of platelet structure may be important.
The coagulation cascade operates in the uterus and endometrium as in other tissues. Platelet accumulation, platelet degranulation and fibrin deposition occur within hours of the onset of menstruation, sealing endometrial vessels. The platelet count in menstrual discharge is only one-tenth of that seen in peripheral blood. This is probably due to the consumption of platelet aggregates in endometrial blood vessels early in the haemostatic processes of menstruation (de Merre et al 1967). Additionally, these platelets have been found to be devoid of granules, and they fail to aggregate when challenged with aggregating stimuli such as adenosine diphosphate and collagen. Compared with peripheral blood platelets, those in menstrual fluid do not produce appreciable cyclo-oxygenase products from arachidonic acid (Christiaens et al 1981, Sheppard et al 1983, Rees et al 1984).
Since prevention of clot formation is necessary to deter scarring and obliteration of the endometrial cavity, there appears to be an active fibrinolytic system in the endometrium mediated by plasmin through proteolytic cleavage of plasminogen by the activators urokinase-type plasminogen activator (uPA) and tissue-type plasminogen activator (tPA). These activators are regulated by plasminogen activator inhibitor 1 (PAI-1) and PAI-2, both of which are expressed in the endometrium. The presence of these fibrinolytic agents suggests that coagulation occurs but is rapidly reversed. Oestradiol stimulates uPA, whilst progesterone inhibits uPA (Casslen et al 1986). Furthermore, progesterone inhibits production of tPA, and this effect is amplified by production of PAI. Blood coagulation products have also been found to be severely depleted in menstrual fluid, suggesting consumption during menstruation (Hahn 1980).
Fibrin formation occurs during the process of menstruation (Beller 1971, Sheppard et al 1983). The high levels of fibrin degradation products in menstrual fluid are not solely due to direct digestion. Although menstrual blood contains high levels of fibrin and fibrin degradation products, it contains no fibrinogen. Similar levels of protein C1 inactivator, α2-macroglobulin and α2-antitrypsin have been found in peripheral and menstrual blood (Daly et al 1990). However, compared with peripheral plasma, lower levels of activated prothrombin, antithrombin, antiplasmin, plasminogen, protein C and factors V, VII, VIII and X have been reported in menstrual fluid (Rees et al 1985, Daly et al 1990).
Compared with peripheral plasma, menstrual blood has a marked increase in the level of tPA antigen with reduced levels of PAI. Levels of fibrinolytic enzymes in menstrual fluid vary according to cycle stage. Throughout the cycle, higher levels of tPA are found in the myometrium compared with the endometrium in the normal menstrual cycle (Sheppard 1992), whilst during the late secretory phase, several studies have demonstrated a significant increase in endometrial tPA levels.
The above mechanisms ensure that progesterone falls and oestradiol rises during menstruation. There is increased fibrinolytic activity in the endometrium of women with increased menstrual blood loss. The endometrium also generates factors that inhibit platelet aggregation and platelet adhesion. Such factors include prostacyclin, nitric oxide and platelet-activating factor (PAF) (Kelly et al 1984, Alecozay et al 1991).
Vasoconstriction
In the menstruating uterus, haemostatic events are strikingly different from the rest of the body (Christiaens et al 1980). At the onset of menstruation, damaged blood vessels are sealed by intravascular thrombi of platelets and fibrin. However, as menstruation progresses, the functional endometrium is shed and so these haemostatic thrombi are lost. Subsequently, during the first 20 h of menstruation, intense vasoconstriction of the spiral arterioles occurs; this assures haemostasis until regeneration of the endometrial surface is complete.
The role of prostaglandin F2α (PGF2α) in vasoconstriction is well established. The effects of the vasoconstricting PGF2α are balanced by those of the vasodilating prostaglandin E2 (PGE2). Concentrations of both these prostaglandins are increased in the luteal phase. Overproduction of the vasodilatory prostaglandins or reduced production of the vasoconstrictors is likely to lead to excessive blood loss at the time of menstruation. This is confirmed by work showing an elevated PGE2/PGF2α ratio, increased endometrial PGE2 or increased PGE2 receptor concentrations in women with HMB (Smith et al 1981, Adalantado et al 1988). Steroid hormones influence endometrial prostaglandin synthesis, and the highest levels of the latter are found during menstruation. This is particularly true for PGF2α, the synthesis of which rises significantly during the secretory phase of the menstrual cycle under the influence of progesterone. It is also likely that the upregulation of the cyclo-oxygenase pathway impacts on angiogenesis in the endometrium.
Other vasoconstrictors, such as endothelins and PAF, may also contribute. Endothelins are powerful vasoconstrictors, and are found in human endometrium (Cameron et al 1992). They are thought to be involved in paracrine regulation of a number of endometrial functions, such as the induction of vascular and myometrial smooth muscle contractions, fibroblast mitogenesis and the release of other paracrine agents (Ohbuchi et al 1995). Endothelins can also induce PGF2α. It has been proposed that as endometrial glandular epithelium breaks down during menstruation, stored endothelin gains access to the spiral arterioles, causing long-lasting vasoconstriction (Cameron and Davenport 1992).
PAF is present in the endometrium in the luteal phase and has an ambiguous effect on spiral arteriolar tone. PAF itself is a vasoconstrictor but it stimulates production of the vasodilator PGE2 (Björk and Smedegård 1983, Smith and Kelly 1988).
Endometrial repair
Vasculature
Seventy percent of menstrual loss arises from the spiral arterioles, and most of this bleeding occurs within the first 3 days of menses (Haynes et al 1977). Concordantly, vessel repair begins within the first 2–3 days of the onset of bleeding. As might be expected, the endometrium is a rich source of angiogenic growth factors (Smith 1998). The fibroblast growth factor (FGF) and the vascular endothelial growth factor (VEGF) families are the best known.
Vascular endothelial growth factor
VEGF is a heterodimeric angiogenic growth factor which is expressed and secreted by a variety of endometrial cells, including macrophages and large decidualized cells. They cluster around the spiral arterioles during the late luteal phase (Gospodarowicz et al 1989, Charnock-Jones et al 1993). The variants of VEGF differ in their cellular localization.
Steroids and hypoxia regulate VEGF, with steroid regulation being around two orders of magnitude lower than that of hypoxia. VEGF mRNA is abundant in the endometrium at the time of menstruation (Charnock-Jones et al 1993). This upregulation probably follows the hypoxia induced by spiral arteriole vasoconstriction (Sharkey et al 2000). Its expression is followed by rapid angiogenesis when the functional endometrium is lost.
Vascular smooth muscle cells (VSMCs) in endometrium also express certain VEGF receptors. This provides a direct link between the development of spiral arterioles and VEGF-A expression. The finding of reduced proliferation of VSMCs in patients with HMB could be explained by altered VEGF-A expression. A novel angiogenic factor, endocrine gland VFGF, and its receptors have recently been reported to be expressed in the uterus (Battersby et al 2004).
Fibroblast growth factor
The FGF family consists of at least eight members, some of which are expressed in the endometrium and are hormone dependent with expression during the proliferative phase and reduction during the secretory phase (Basilico and Moscatelli 1992, Ferriani et al 1993). FGF synergizes with VEGFs in inducing angiogenesis (Pepper et al 1992). Inhibition of FGF action does not fully inhibit angiogenesis, but this is possible when anti-VEGF agents are used. VEGFs promote the release of FGF from the ECM. FGF and platelet-derived growth factor are also known to stimulate angiogenesis, and have been demonstrated in the endometrium of a number of a species (Weston and Rogers 2000).
Angiopoietins
The angiopoietins are another family of molecules which influence vascular development and maintenance by stabilizing the endothelial cell and vascular smooth muscle structure (Suri et al 1998). Recently, expression patterns of the angiopoetin family in the human endometrium have been examined, although results have been conflicting, perhaps reflecting relatively low expression of these factors (Rogers and Abberton 2003). It is possible that this will alter the function of the VSMCs that are essential for controlling the blood loss at menstruation, and that HMB is due to aberrant build-up of VSMCs. Angiopoietin expression and signalling are regulated by the cyclo-oxygenase enzymes.
Epithelium
Once the functional layer of the endometrium is shed, the regeneration of all cell types (epithelial, endothelial and stromal) occurs rapidly. This regeneration occurs from the cells of the remaining basal layer, which acts as the germinal compartment of the functional endometrium (Chan et al 2004).
Given its dynamic nature, growth factors and cytokines are especially important in the development of the endometrium. In addition, tissue remodelling is dependent on the integrity of the ECM. Profound hormone-dependent tissue remodelling is seen in the endometrium, although currently there is no direct evidence linking disorders of tissue regeneration with HMB. Proliferation of the endometrium is most active during menstruation. The proliferation starts on day 2 in the basal glands and is complete by day 5, leaving a completely re-epithelialized endometrial surface. Various agents regulate this process, the best-known being epidermal growth factor (EGF). EGF is expressed in human epithelial cells throughout the cycle and stimulates endometrial epithelial proliferation (Haining et al 1991, Zhang et al 1995). Oestradiol induces the expression of EGF and its receptor. However, during menstruation, when the concentration of oestradiol is at its lowest, there are sufficient amounts of residual EGF remaining in the epithelial cells as growth continues before oestradiol levels increase during the proliferative phase. Additionally, EGF has been shown to mimic the proliferative effect of oestradiol in the endometrium of transgenic mice lacking the oestradiol receptor (Ignar Trowbridge et al 1993). Numerous other growth factors (insulin-like growth factor, FGF) and their binding proteins are expressed in the endometrium, and all promote cell proliferation. The complexities of tissue remodelling suggest that altered wound healing may be a factor in the aetiology of HMB, and its role warrants further investigation.
Matrix
Integrins are the agents which link the ECM with epithelial cells of the endometrium (Hynes 1987, Lessey et al 1992). The MMPs are a highly regulated family of calcium and zinc endopeptidases, which are able to degrade most components of the ECM in the activated state. These enzymes are active in normal and pathological processes involving tissue remodelling. MMP activity is greatest during days 1–4 of the cycle. Progesterone withdrawal and migratory leukocytes activate some MMPs, and this may be a significant step in the initiation of menstruation. Local release of agents such as MMP-1 and MMP-3 from stromal cells may activate other proteases released from the invading neutrophils (Schatz et al 1999). This is supported by the presence of substantially more latent MMP-9 than active MMP-9 before menstruation (Rigot et al 2001). Abnormalities in the dynamic turnover of collagen production are likely to be important in menstrual upset given their importance in other vascular and fibrotic processes.
Normal Menstrual Cycle
The majority of cycles lie between 24 and 32 days, and a normal cycle is considered to last for 28 days. Menstrual cycle length varies during reproductive life, being most regular between the ages of 20 and 40 years. It tends to be longer after the menarche and shorter as the menopause approaches. The mean menstrual blood loss per menstruation in a healthy Western European population ranges between 37 and 43 ml; 70% of the loss occurs in the first 48 h. Despite large interpatient variability, loss between consecutive menses in the same woman does not vary largely (Figure 31.3). Only 9–14% of women lose more than 80 ml per menses, and 60% of these women are actually anaemic. The upper limit of normal menstruation is thus taken as 80 ml per menses (Rybo 1966). However, total fluid loss (mucus, tissue, etc.) may be considerably more than the blood loss alone and amounts vary. These parameters have been reviewed recently (Fraser and Inceboz 2000).
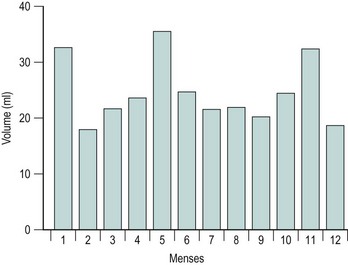
Figure 31.3 The variability in menstrual blood loss in a single individual: values for 12 consecutive periods.
From Hallberg L, Nilsson L 1964 Consistency of individual menstrual blood loss. Acta Obstetrica et Gynecologica Scandinavica 43:352–359, Blackwell Science.
The pattern of spontaneous myometrial contractions varies during the menstrual cycle (Lumsden et al 1983). Time-lapse ultrasound studies have shown that propagated myometrial contractions in the non-pregnant uterus emanate from the JZ. The frequency and direction of these contractions depend on the phase of the cycle. In the follicular and periovulatory phases, cervicofundal subendometrial contractions can be seen, the amplitude and frequency of which increase notably towards ovulation. Short, asymmetrical myometrial waves are present during the luteal phase, but propagated fundocervical subendometrial contraction waves are noted during menstruation (de Vries et al 1990, Chalubinski et al 1993).
Kunz et al (1996) demonstrated the role of preovulatory cervicofundal contractions in assisting the rapid transport of sperm through the female reproductive tract. Others have postulated that the asymmetrical myometrial peristalsis during the luteal phase serves to maintain the developing blastocyst within the uterine fundus. The role of fundocervical contractions during menstruation is likely to be important in controlling menstrual flow and limiting retrograde menstruation (Chalubinski et al 1993).
Abnormal Menstruation
Heavy menstrual bleeding (HMB)
The causes of HMB fall into four categories:
Dysfunctional uterine bleeding
Anovulatory dysfunctional uterine bleeding
Occasionally, anovulatory cycles occur in all women. Chronic anovulation, however, is associated with an irregular and unpredictable pattern of bleeding ranging from short cycles with scanty bleeding to prolonged periods of irregular heavy loss. Normal bleeding occurs in response to withdrawal of both progesterone and oestradiol. If ovulation does not occur, the absence of progesterone results in an absence of secretory changes in the endometrium, accompanied by abnormalities in the production of steroid receptors, prostaglandins and other locally active endometrial products. Unopposed oestrogen gives rise to persistent proliferative or hyperplastic endometrium, and oestrogen withdrawal bleeding is characteristically painless and irregular. It tends to occur at the extremes of reproductive life but is rare at other times. Only 20% of those cycles with excessive menstrual blood loss are anovulatory (Haynes et al 1979); this same study failed to demonstrate any abnormalities in gonadotrophin or circulating steroid concentrations. In anovulatory cycles, the endometrium is unable to produce factors whose synthesis is controlled by progesterone, such as PGF2α (Smith et al 1982). This may account for the painless nature of the bleeds. Ovulation occurs in response to the mid-cycle surge of luteinizing hormone (LH). If this fails to occur due to insufficient oestradiol secretion or impaired positive feedback, ovulation will not occur.
Failure of follicular development
Follicular development which is insufficient to produce an oestrogen signal strong enough to induce an LH surge is one of the common reasons for the irregularities in menstrual cycle pattern in premenopausal women. This occurs perimenopausally and in PCOS (see Chapter 18 for information regarding the aetiology and treatment of PCOS).
Anovulatory bleeding may be associated with cystic glandular hyperplasia of the endometrium (see Figure 31.2E). This occurs in some older women and also in peripubertal girls where unopposed oestrogen secretion occurs. The first few cycles after the menarche are commonly anovulatory. However, if anovulation persists, a long period of amenorrhoea is accompanied by endometrial hyperplasia. This is probably a result of multiple follicular development (multicystic ovaries) with failure of antral follicle formation. Endometrial hyperplasia may cause excessive bleeding, anaemia, infertility and even cancer of the endometrium.
Ovulatory dysfunctional uterine bleeding: idiopathic bleeding
As described above, an important factor in the control of menstrual blood loss is vasoconstriction. It appears that there are a number of endometrial products which alter the degree of vasoconstriction, and thus may affect the volume of menstrual blood lost. In the mid-1970s, a relationship between prostaglandin production and HMB was suggested by work showing that total endometrial prostaglandin content was proportional to menstrual loss. It appears that a shift in endometrial conversion of endoperoxide from the vasoconstrictor prostaglandins (PGF2α) to the vasodilator prostaglandins (PGE2 or prostacyclin) occurs. However, it is likely that it is not only prostaglandins that are of importance, and work is now being performed on the role of endothelin in heavy menstrual loss. Endothelins are very potent vasoconstrictors that are produced within the endometrial vessels; their receptors are also present, although it is not yet clear whether either of these two factors differ in women with heavy menstrual loss. Although studies to date are limited, Marsh (1996) showed reduced immunostaining for endothelin in the endometrium of women with HMB, implicating this peptide in the pathophysiology of increased menstrual blood loss. This is a rapidly changing area of knowledge, and it is likely that other elements will come to light which will be significant. According to Jabour et al (2006): ‘Further challenge in the future will be the development of experimental strategies that will allow us to assess the exact role of the various factors deduced from gene mining studies in menstrual function/dysfunction and which of these gene pathways constitute a sensible target for novel therapeutic application in the clinic.’
It is still uncertain why there is a difference in production of local factors in those with heavy menstrual loss compared with those with normal loss. Interest has centered on the role of steroid hormones, but it has been impossible to demonstrate either a difference in the circulating levels of oestradiol and progesterone or in the receptor concentration within the endometrium (Critchley et al 1994). It is possible that there is a genetic difference altering the production of local hormones and growth factors, or that there is a multifactorial aetiology. Reference has been made above, in discussion of the control of menstrual blood loss, to areas of research which may throw new light on the mechanism of HMB.
Coagulation disorders
Although certain haemorrhagic conditions, e.g. thrombocytopenic purpura and von Willebrand’s disease, are associated with an increased incidence of HMB, coagulation disorders have a variable effect overall. There is no impairment of systemic coagulation in those with excess menstrual loss, nor are fibrin degradation products elevated in the menstrual fluid of those with heavy menstrual loss (Bonnar et al 1983). In women with thrombocytopenia, menstrual blood loss correlates broadly with platelet count at the time of the menses. Splenectomy has been known to reduce menstrual blood loss dramatically in these patients.
Women with factor VII deficiency exhibit a spectrum of bleeding symptoms, with HMB being one of the most common (Kulkarni et al 2006). There is no need to test for these routinely unless HMB has been present from adolescence or the woman encountered bleeding problems with dental extraction.
Dysmenorrhoea
Idiopathic (primary) dysmenorrhoea
Uterine hyperactivity
The significance of uterine hyperactivity in women with dysmenorrhoea was first proposed in 1932. Since then, there has been much research which suggests that women with dysmenorrhoea have increased uterine activity during menstruation (Novak and Reynolds 1932, Filler and Hall 1970). Patients often describe the pain as ‘labour-like’, and an increase in uterine contractility can be demonstrated by measuring intrauterine pressure in those with dysmenorrhoea compared with women without dysmenorrhoea (Lumsden and Baird 1985). The increased uterine contractility also appears to be related to uterine blood flow and the presence of pain (Åkerlund and Anderssen 1976).
During the reproductive years, the myometrium is structurally and functionally polarized (see above). Using transvaginal ultrasound scanning or MRI, it is possible to delineate between the myometrial zones (Brosens et al 1998, Lesny et al 1999).
Patients with endometriosis and adenomyosis have been found to have structural and functional abnormalities of the JZ (Brosens et al 2000). Leyendecker et al (1996) demonstrated marked hyperperistalsis of the JZ during the early- and mid-follicular phase in women with endometriosis, associated with a marked increase in the transport of inert particles from the vaginal depot to the peritoneal cavity.
Dysmenorrhoeic patients have been found to exhibit profound structural changes in the JZ, including irregular thickening, and smooth muscle hyperplasia characterized by closely packed smooth muscle fibres which are poorly oriented and less vascular than the smooth muscle of normal inner myometrium (Togashi et al 1989, Brosens et al 1998). Consequently, the term ‘junctional zone hyperplasia’ was coined for this disorder (Brosens et al 1998).
Dysperistalsis and hyperactivity of the uterine JZ are important mechanisms of primary dysmenorrhoea, and possibly menstrual pain associated with adenomyosis and endometriosis (Brosens et al 2000).
Endothelins
Endothelins are potent uterotonins in the non-pregnant uterus. They are thought to be involved in the induction of myometrial smooth muscle contraction in a juxtacrine fashion. The greatest density of endothelin-binding sites is found on glandular epithelium in the endomyometrial junction, and recent evidence suggests that endothelins in the endometrium can induce PGF2α and further endothelin release in a paracrine and autocrine fashion (Bacon et al 1995). Local ischaemia could further increase the expression of endothelins and prostaglandins, which could further aggravate uterine dysperistalsis.
