Fig. 16.1
Integrated direct digital mammography system. 1 X-ray tube, 2 X-ray beam, 3 compression paddle, 4 breast support, 5 detector, 6 C-arm, 7 monitor for angle, breast thickness and compression force (Image is courtesy of Mário Oliveira)
The stages for production of mammography images are acquisition, processing, display and post processing for interpretation and storage. In digital mammography each step is performed by an individual system that can be independently assessed and optimised. The image acquisition system is composed of an X-ray tube, breast compression plate and image receptor system.
The distance from the X-ray focus to the breast support platform is commonly around 60 cm. A moving anti-scatter grid is normally used which is situated just behind the low-attenuation (often carbon-fibre) table top and in front of the image receptor. Some designs work without an anti-scatter grid and make a software correction for the large-scale effects of scattered radiation in the image.
Due to the requirements for very high resolution, X-ray focal spot sizes must be small. Focal spots of approximately 0.3 × 0.3 mm are used for conventional mammography, with a size of 0.15 × 0.15 mm selectable for magnified views, where the breast is raised away from the image receptor on a special magnification table in order to produce a geometrically magnified view.
The X-ray tube is positioned within the unit so that the anode heel effect is employed to reduce X-ray intensity towards the nipple side of the field where the breast will be thinner. Heavy reliance is placed on the automatic exposure system of modern mammography units. These systems are capable of sensing the thickness and composition of the compressed breast and then automatically selecting the tube potential, target and filter combination and exposure time required to give the optimal imaging exposure within the constraints of patient dose limitations.
The Mammographic X-Ray Spectrum
The X-ray spectrum from a conventional tungsten target, glass encapsulated, aluminium filtered X-ray tube is not optimal for mammography. The best subject contrast for between normal and malignant tissue is considered to be produced at a photon energy of around 20 keV, which is much lower than that used in normal radiography. Increasing photon energy will reduce contrast and reducing photon energy will lead to inadequate penetration of the breast and a large increase in patient dose, so the X-ray spectrum is critical. A range of mammographic spectra are used for digital mammography. The X-ray tube target may well be switchable (depending on the design) between molybdenum and rhodium, or rhodium and tungsten and the tube has a low attenuation beryllium output window. The beam is then filtered with either molybdenum, rhodium, silver or aluminium filters. The X-ray tube is operated at a voltage in the range 25–35 kV.
Figure 16.2 shows the spectrum of a rhodium target, rhodium filtered beam at a tube voltage of 30 kV. Rhodium has characteristic X-ray peaks at 20.2 and 22.7 keV, which contribute strongly to the limited range spectrum. Rhodium is again used as the filter because, due to the K-edge absorption, it strongly attenuates energies just above its own K-characteristic peaks as well as attenuating lower energies. The end result is a spectrum with most photons lying in a narrow band of energies.


Fig. 16.2
X-ray spectrum for a typical rhodium target, rhodium filtered mammographic x-ray beam at 30 kV. The spectrum peaks around 20 keV due to the K-edge filtration produced by the rhodium filter. This spectrum is suitable for imaging moderate-sized breasts
Although the most common spectrum, this is not necessarily optimised for all breast thicknesses. For larger breasts, a more penetrating beam is optimal to avoid very long exposure times which bring the possibility of movement blur, tube overloading and high radiation doses. Figure 16.3 shows the spectrum of a tungsten target, aluminium filtered beam again at a tube voltage of 30 kV, with the X-ray tube again having a beryllium output window. Although the tungsten anode, aluminium filter combination was not used with film-screen mammography, it is well suited to the response of modern digital mammography receptors and is becoming a more common choice.


Fig. 16.3
X-ray spectrum for a typical tungsten target, aluminium filtered mammographic x-ray beam at 30 kV
The shape of the spectrum is quite different from Fig. 16.2 and its peak is now at a higher energy, even though the tube voltage is the same. The tungsten target has no K-characteristic X-ray peaks in this energy range, and the aluminium filter, which similarly does not have a K-absorption edge in this energy range, does not preferentially attenuate the higher energy end of the spectrum.
Compression Paddle Design
In mammography the breast is compressed using a rigid transparent plastic compression plate which can be motor driven. The use of compression force reduces the thickness of the breast and holds it in place which gives a number of advantages:
Better spatial resolution. The breast is brought closer to the imaging receptor so that magnification and focal spot blurring is reduced.
Reduced movement blur, even at the relatively long exposure times (1 s typical) common in mammography.
Less scattered radiation in the image. The beam path length through the breast is shorter, so there is less material to do the scattering. Reducing the proportion of scattered radiation in the image improves image contrast and reduces image noise.
Improved image uniformity. Compression spreads the breast tissue out more evenly across the image and makes pathology easier to detect.
Reducing the compressed breast thickness diminishes exposure time, decreasing the radiation dose delivered to the breast [8, 9].
The reduced path length makes practicable the use of lower energy (less penetrating) X-ray spectra. This gives greater subject contrast.
Small areas of pathology buried in glandular tissue can be better visualised, as malignant tissues tend to be firmer.
Compression in mammography is one of the few occasions in radiography where a technical advantage is gained without detriment to other aspects of the image; although there is a disadvantage in client discomfort. Modern mammography units can employ a system to measure the increasing amount of force resulting from a given small increase in compression to stop the motorised movement at a given compression. Many units use a motor driven assembly with more or less compression being applied by the practitioner; the practitioner has direct control over the amount of compression applied. It is suggested that the compression paddle must be controlled by hand during the final compression [8].The compression force maximum limit set on mammography systems is 200 Newtons. A range of compression paddles are normally supplied with a digital mammography unit to cover different types of projection. Some typical types are:
Flat rigid paddle:
This is the basic flat paddle that covers the whole of the area of the digital image receptor. The paddle maintains its shape parallel to the plane of the receptor and deforms only slightly when the compression force is applied. This is used for full-field MLO and CC views.
Tilting flat paddle:
This is a flat paddle that allows rotation against a spring resistance so that on compression the chest-wall edge of the plate will be higher than the nipple side. The advantages are claimed to be that the design holds the breast in place more firmly. This type of paddle is also used for full-field MLO and CC views.
Sliding compression plate:
This plate is suitable for imaging smaller breasts where the full area of the image receptor is not required. By sliding the plate to one side or the other, the MLO view can be achieved using the edge of the breast support table to improve positioning.
Spot compression plate:
This plate has a raised cylindrical area that applies extra compression force over a small area (Fig. 16.4). The advantages to spot compression are that better compression over the small area of interest is obtained, with all of the advantages above, but also that the spreading of surrounding parenchyma allows the outline of masses to be better visualised. Whereas features in superimposed tissue will spread out, mechanically harder malignant tissues will tend to retain their shape. Spot views are an additional examination often performed at assessment.
Fig. 16.4
Spot compression plate: this plate has a raised cylindrical area that applies extra compression force over a small area
Magnification compression plate:
For magnification views, a different breast support table is used that raises the breast away from the plane of the image receptor by some 30 cm (depending on the magnification factor and focus-to-receptor distance), so that the image is geometrically magnified. The compression plate for this is smaller, as the X-ray field is smaller close to the focus, and often has a step in the support arm to allow it to fix to the compression system at a point lower than the magnification support table (Fig. 16.5). See also Chap. 25.
Fig. 16.5
Magnification compression plate: the compression plate for this is smaller, as the x-ray field is smaller close to the focus, and it often has a step in the support arm to allow it to fix to the compression system
Biopsy compression plate:
Further Advances
Recently American Mammographic has developed a paddle for screening mammography called S.O.F.T. This new paddle is used as an alternative to the conventional flat compression paddle allowing a tilt for superior compression of the mid and anterior-breast with less patient discomfort.
Another compression paddle was developed also to reduce the pressure and discomfort on the thickest parts of the breasts. The compression paddle bends along the breasts when the paddle touches them. Also, with three slits on the front side and right and left lateral sides of the paddle, the pressure is dispersed.
The discomfort to the patient is the negative aspect of the compression although the tolerance to compression is variable among women (see Chap. 14). No recommendation is provided regarding the suitable compression force to take into account the characteristics of the breast, namely compressibility, composition and thickness. Several studies [10–15] investigated the best compression force in terms of dose, IQ and patient tolerance. One of these studies concluded that the amount of compression force has noticeable effects on IQ. Moreover, higher IQ rates were consistently associated with higher compression forces. The mean compression force required to produce a “perfect” image in digital systems was 121.3 N for CC and 134.2 N for MLO, whereas for analogue systems the compression force was 112.2 N and 129.7 N for CC and MLO, respectively. In this group of 1,200 patients, 2 % expressed dissatisfaction with the endured compression force [10].
Chapter 22 gives details on a new approach to breast compression, employing a system that uses pressure instead of force.
Digital Mammographic Image Receptors
Digital image capture was first introduced into mammography as ‘small-field digital mammography’ for needle and core biopsy guidance using detectors typically approximately 15 cm in size. Full-field digital mammography, with detector sizes up to the equivalent of the 24 × 30 cm was developed later.
The imaging advantages of digital mammography include a wide dynamic range and the separation of the image capture and image display functions, so that the image display can be varied to optimally show the full range of recorded X-ray intensities. This provides good visualisation of the skin line and nipple and has advantages when imaging dense breasts and younger women. There are a range of competing image capture technologies for digital mammography, and the choice of technology depends to some extent on the imaging task, be it screening or symptomatic, and the size and format of the hospital or clinic environment in which it will be operated. The range of technologies described below fit the state of the market at the time of writing, but this is an area where progress is still rapid.
General Features of Digital Mammographic Images
A digital image is not a continuous distribution of bright and dark, but is composed of a finite number of points (or ‘pixels’), where each pixel has a value of brightness dictated by a stored numerical value. Digital images have the advantage that they can be enhanced and manipulated by computer to extract the maximum amount of diagnostic information. Digital images can be stored, transferred, copied without detriment and retrieved in a very efficient manner using computer mass data storage techniques. They have the disadvantage of a limit to spatial resolution caused by the finite pixel size. Digital mammography receptors usually have a linear response between pixel value and the radiation dose incident on the pixel over a very wide dynamic range, typically a factor of some 10,000:1. The choice of what patient dose is required for digital mammography is therefore driven by the signal-to-noise ratio required for a diagnostic image rather than a specific radiation dose to the receptor.
The Direct Digital Detector: Amorphous Selenium
In direct conversion detectors the X-ray interaction is converted directly to an electrical signal using an amorphous selenium (a-Se) layer, behind which lies an amorphous silicon micro-circuit layer which in turn is supported by a rigid substrate (Fig. 16.7). Selenium is a photoconductor, so is an electrical insulator in the dark, and a conductor when exposed to light or X-rays. The amorphous selenium is employed as a mammographic image receptor in the form of a thin layer (0.5 mm) with a voltage applied between a large area electrode across the front surface, and an array of charge collection electrodes, one per pixel, on the back surface. These are linked to capacitors to accumulate the charge released during the exposure. These are linked in turn to thin-film-transistor switches to provide a line-by-line read out arrangement in which the charge stored for an individual pixel is passed pixel-by-pixel along the line until it can be measured by electronics external to the imaging sensor. Incoming X-ray photons interact photo-electrically in the a-Se layer producing electrons and ‘holes’ (the vacancy where an electron should be). Because of the high voltage gradient across the thin a-Se layer, the electrons move towards the positive surface electrode and the holes towards the negative charge collection electrodes. The electrons and holes do not move sideways as they have to follow the direction of the electric field gradient, so image blurring from this source is minimal and the spatial resolution of the detector is good. At the end of the exposure, the charge signals (proportional to the radiation detected) from each pixel are read out via the thin-film-transistor switches and data lines. The charge signals are converted to digital values via charge amplifiers and with a digital-to-analogue converter and sent to the computer for assembly into an image.


Fig. 16.7
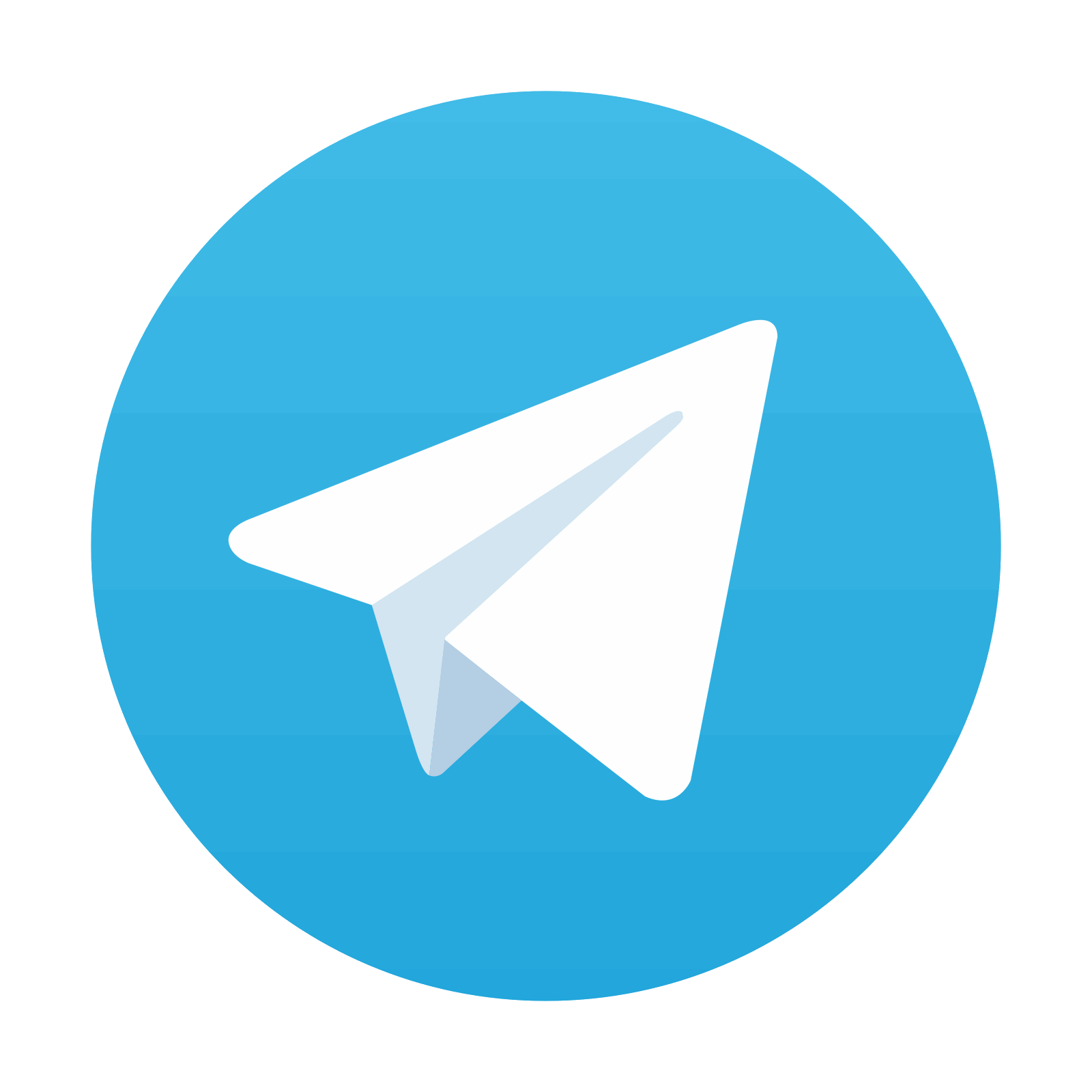
Cross-section through a direct digital mammography image receptor. The photoconductor is a layer of amorphous selenium that allows electrons to flow across it when exposed to x-ray photons. The capacitor builds up a charge, proportional to the x-ray exposure for that pixel. The charge is transferred out of the device via the switch at the end of the exposure and converted to a numerical pixel value
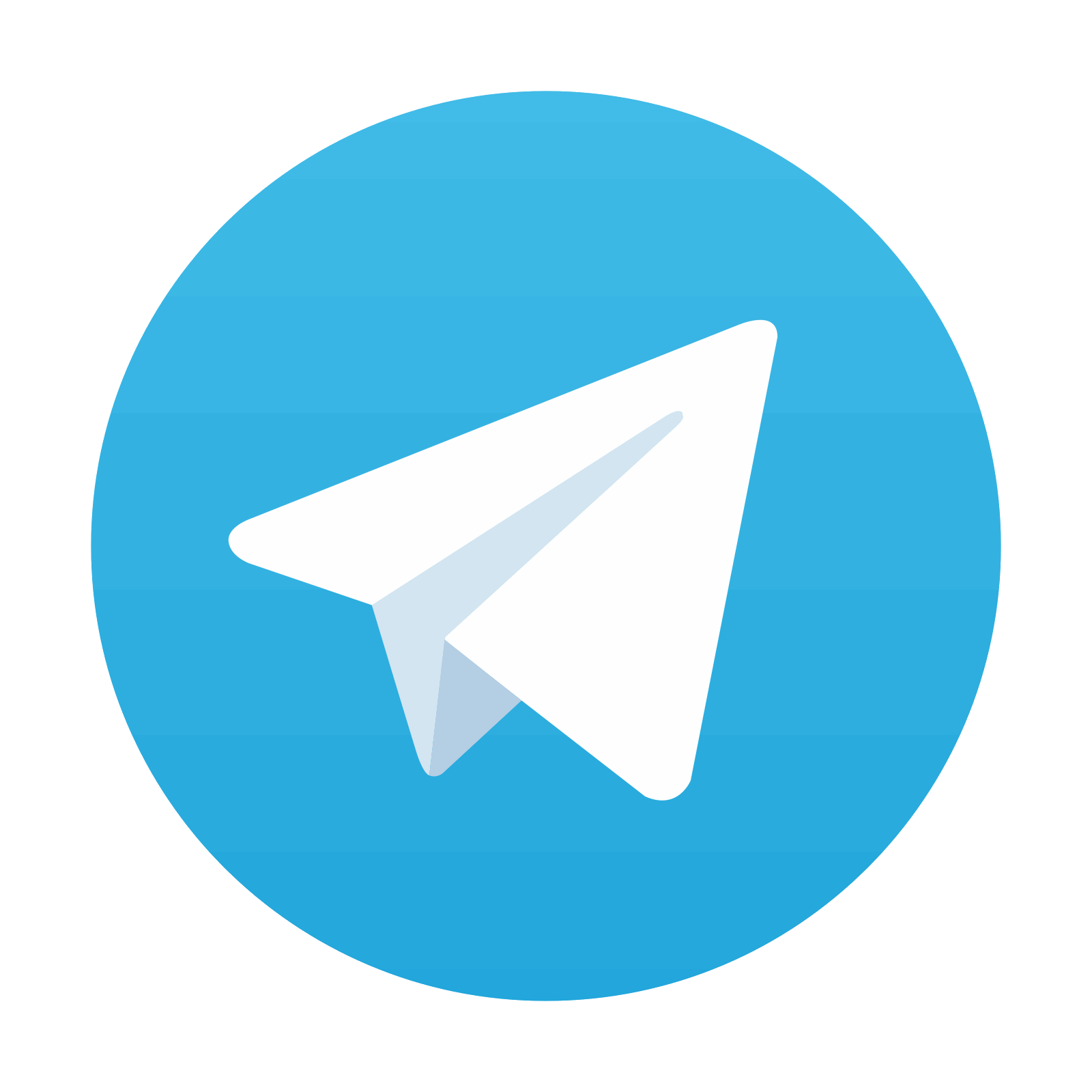
Stay updated, free articles. Join our Telegram channel

Full access? Get Clinical Tree
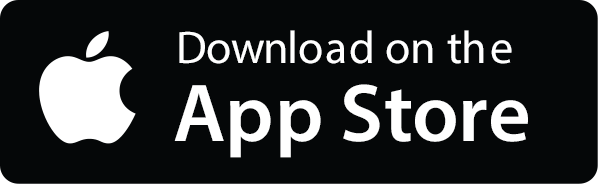
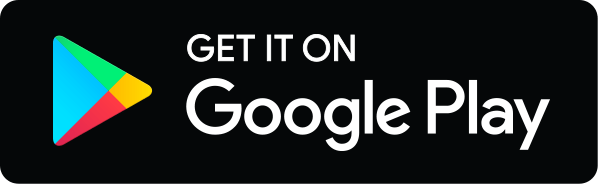
