Fig. 8.1
Schematic representation of organization and compaction of sperm chromatin
Nuclear Proteome of the Spermatozoa and Its Role in DNA Stability
The first sign of sperm chromatin packaging is a massive increase in the level of acetylation of core histones as revealed by immunocytochemistry and western blot analysis. This results in the incorporation of noncanonical, replication-independent testis-specific histone variants into the nucleosomes of developing spermatocytes and implies that histones are displaced prior to global replacement [28]. It is therefore, imperative to understand the attributes of the core histones of the nucleosome-bound DNA of the sperm chromatin that assist in the formation of the nucleoprotein make up of the spermatozoa. In early spermatids, DNA is compacted around nucleosomes, the universal organization units of the genome. A nucleosome is comprised of DNA coiled around an octamere of canonical histones (i.e., H2A, H2B, H3, and H4) [16]. These are a subset of histones found in somatic chromatin and are more susceptible to covalent modifications such as methylation, acetylation, ubiquitination, and phosphorylation. Each of these chemical modifications to histones works alone or in concert, under the name of the “histone code” to influence gene repression and/or activation [23]. These subsets of histones include histone H2 that takes the form of two minor variants, called H2A.X and H2A.Z , and the histones H3 and H4 and are extensively acetylated. As a prelude to removal of the histones, the stable nucleosome structure is relaxed by processes linked with acetylation of histone H4 [29]. Within the amino-terminal tail of histone H4, four lysines can be acetylated: lysine 5, lysine 8, lysine 12, and lysine 16 (H4K5, H4K8, H4K12, H4K16). In humans, however, H4K8 and H4K16 acetylations occur in elongating spermatids. In addition, acetylation of histone H3 (H3K9) can be detected in elongating spermatids of humans [1, 15]. It has been reported that these modified histones are responsible for the formation of slightly smaller nucleosomes that apparently lack either H3 or H4 and repackage at least some of the pericentric/chromocentric DNA, providing evidence for novel nucleosome-like complexes in spermnuclei that are delivered to the egg at fertilization (Fig. 8.2). The establishment and removal of acetylation is accomplished by histone acetyl transferases (HATs) and deacetylases (HDACs), respectively. Histone acetylation relaxes chromatin and makes it accessible to transcription factors, whereas deacetylation is associated with gene silencing [23]. It has been hypothesized that H4 hyperacetylation in mammalian spermatids leads to an open chromatin structure that facilitates and induces histone displacement. Further evidences suggest that sperm H4Ac being species-specific is either not lost from the sperm during pericentric condensation or is present as a separate, nonpericentric compartment, possibly located in the posterior of the sperm [30] or peripheral nuclear regions [31]. It is a matter of concern as to whether these paternally derived histones contribute to and persist in zygotic chromatin is currently unresolved. However, both H2AL1/2 rapidly disappear after fertilization in the mouse [32] while H3.1/H3.2 persists in human and mouse zygotes prior to DNA replication which could be an effect of the heterologous system used [26]. Apart from acetylation, histone methylation signals have been observed in elongating spermatids, such as strong H3K4 mono-, di-, and tri-methylation. These modifications in concert with acetylation might assist in achieving a more-open chromatin configuration. It has been seen that H3K4methylation is generally associated with gene expression whereas H3K9 and H3K27 methylation is linked to gene silencing and heterochromatin. Nevertheless, methylation pattern associated with repressed chromatin are observed in elongating spermatids which includes H3K9 mono-, di-, and tri-methylation as well as H3K27 di- and tri-methylation [19, 33]. However, the timing of establishment and removal of methylation markers is critical to spermatogenesis. It has been often observed that the methylation level of H3K4 peaks in spermatogonial stem cells which signals the stem cells to begin differentiation and to go on to become spermatocytes and is removed during meiosis. In contrast, the methylation level of H3K9 and H3K27 increases during meiosis, but the removal of H3K9me at the end of meiosis is essential to the onset of spermiogenesis [23, 34]. Expression of different histone methyltransferases and demethylases has been observed during spermatid elongation. This coexistence of both types of enzymes might be crucial to balance regions of “opened” and “closed” chromatin. However, it has been demonstrated in human spermatozoa that histone enrichment were not randomly distributed but were rather enriched at loci important for embryo development which are transmitted to the oocyte during fertilization. These loci included imprinted gene clusters, miRNA, HOX gene clusters, developmental promoters, and signaling factors [35]. Similarly, Arpanahi et al. found that histone-bound DNA regions of human spermatozoa were associated with regulatory regions of the genome [36]. An epigenetic marking of these retained histones was observed showing key developmental genes bivalently marked with H3K4me3 and H3K27me3, as observed in embryonic stem cells. Additionally, H3K4me2 was preferentially located at promoters of developmental gene and H3K4me3 at HOX regions, noncoding RNAs, and paternally imprinted loci [35]. This radical change in chromatin configuration is expected to involve mechanism that facilitates the eviction of nucleosomes in favor of incorporation of transition proteins, followed by a subsequent exchange of transition proteins for protamines. The functional activity of each transition protein is still debatable. Some reports suggest that TP1 decreases the melting temperature of DNA, relaxes the DNA in nucleosomal core particles, and stimulates the DNA-relaxing activity of topoisomerase I which indicates that TPs could help chromatin remodeling by making the DNA more flexible. However, others have reported that neither TP1 nor TP2 is able to cause topological changes in supercoiled DNA. Rather, TP1 has been found to stimulate repair of single-strand DNA breaks [37]. Whatever the case might be, data from knockout mouse model suggests that TP1 and TP2 might not be required for histone removal and protamine loading, yet are important for proper regulation of chromatin structure. Subsequently these transition proteins are replaced by the protamines. Protamines are the major class of sperm nuclear proteins. They are of two types, namely, protamine1 (P1) encoded by a single-copy gene and the family of protamine 2 (P2) proteins (P2, P3, and P4), all encoded by a single gene that is transcribed and translated into a precursor protein [38]. The question then arises as to how the DNA binding proteins (such as protamines) of the mammalian spermatozoa fit themselves into the sperm nucleus? Balhorn proposed a model for protamine–DNA binding that accounts for such discrepancy in sperm volume [39]. He stated that the protamines bind to DNA by lying lengthwise inside the minor groove. He opined that positively charged arginine-rich protamines can completely neutralize the negatively charged phosphate groups of DNA and protamine-DNA complex of one strand would fit into the major groove of a neighboring DNA strand so that the DNA strands of sperm nucleus would be packaged side by side in a linear array. Furthermore, the sperm chromatin is stabilized by inter- and intramolecular disulfide bridges between protamines which enable the whole DNA to be tightly packaged in a small volume. An additional process that occurs during chromatin reorganization in elongating spermatids of mammals is the transient appearance of DNA strand breaks [16, 37]. Presumably, the elimination of nucleosomes during spermiogenesis leaves a great number of unconstrained DNA supercoils in the male germ cell that needs to be removed. This elimination is performed in particular by the introduction of single- or double-strand breaks in DNA that relieves the helical tension. Subsequently, upon elimination of strand breaks, effective mechanisms are employed to seal or repair the DNA backbone.


Fig. 8.2
Schematic representation of proposed mechanism(s) for defective packaging of sperm chromatin leading to early pregnancy loss
DNA Damage and Male Infertility
Mammalian spermatogenesis entails a major biochemical and morphological restructuring of the germ cell DNA into the condensed spermatid nucleus. In association with the chromatin restructuring, other well documented nuclear events includes an increase in histone acetylation, an increase in the activity of ubiquitin system, SUMOylation as well as a change in DNA topology resulting from the elimination of the negative supercoiling induced by the removal of DNA-bound nucleosomes. It has been hypothesized that incomplete protamination could render spermatozoa DNA more vulnerable to damage by endogenous or exogenous agents such as nucleases, free radicals, and mutagens. Thus, progressive oxidation of free sulfhydryl or thiol (SH) groups of protamines to disulfides (SS) groups in the epididymis further stabilizes the compacted sperm DNA [40]. Abnormality in the deposition of sperm protamines during spermiogenesis or incomplete oxidation of sperm protamine SH groups during epididymal transit can lead to enhanced susceptibility of sperm DNA to injury. This results in sperm DNA fragmentation and impaired sperm decondensation during fertilization. Spermatozoa with fragmented DNA may initiate apoptosis and interfere with transmission of paternal genetic information to the developing embryo [41]. Several independent investigators have demonstrated the importance of DNA integrity in predicting male reproductive potential. In fact, sperm DNA damage is an objective marker of sperm function, with a lower coefficient of variation than conventional semen parameters. Besides, the several exogenous sources of sperm DNA damage, it is pertinent to focus on the inherent nature of spermatozoa and its maturation process that may cause for sperm DNA damage. In this regard reactive oxygen species (ROS) which are produced by both exogenous and endogenous factors warrants special mention. In general, a mature spermatozoon is accomplished with a small amount of cytoplasm and hence with a limited supply of cytoplasmic antioxidants. Concomitantly, the plasma membrane of the spermatozoa is rich in unsaturated fatty acids that maintain the fluidity of the membrane. Such property leaves the spermatozoa particularly vulnerable to oxidative stress brought upon by increased generation of ROS [42].
Role of Reactive Oxygen Species in Sperm DNA Damage
The sperm genome is encoded with information that needs to be accurately transmitted to the oocyte—a feature vital for the pre- and postnatal development of the offspring. Under normal physiological conditions, germ cells produce physiological amounts of ROS that modulate gene and protein activities required for maturation, capacitation, acrosome reaction, and oocyte fusion. The pathogenic effect of ROS occurs when an imbalance between pro- and anti-oxidants is disturbed leading to oxidative stress as onserved during sperm maturation in epididymis or in the seminal plasma [43]. ROS are short-lived, highly reactive, autocatalytic, and nonspecific reactive intermediates of metabolism that oxidize lipids, amino acids, and carbohydrates as well as are responsible for DNA strand breaks and mutations. ROS includes hydroxyl ion, superoxide ion, nitric oxide, peroxyl, lipid peroxyl, and Thiyl and nonradical molecules singlet oxygen, hydrogen peroxide, hypochloric acid, lipid peroxides, and ozone [41]. Spermatozoa are constantly exposed to the interphase between oxidation through high amounts of ROS produced by themselves as during metabolism and by leukocytes present in semen ; and reduction by means of scavengers and antioxidants. Generation of ROS involves two mechanisms which includes the nicotinamide adenine dinucleotide phosphate oxidase system at the level of the sperm plasma membrane and/or the nicotinamide adenine dinucleotide-dependent oxido-reductase reaction at the mitochondrial level. Mitochondrial respiration is the main biological source of ROS under physiological conditions [44]. Normally, oxygen is tetravalent reduced to water by the mitochondrial cytochrome c oxidase while incomplete reduction leads to leakage of these radicals [45]. Spermatozoa are densely populated with mitochondria since a constant supply of energy is required for their motility. The lipid composition of plasma membrane of mammalian spermatozoa are rich in polyunsaturated fatty acids (PUFA) , thus, render them susceptible to ROS attack. These lipids contain unconjugated double bonds separated by methylene groups that, weakens the methyl carbon hydrogen bond making hydrogen extremely susceptible to abstraction and oxidative damage. When the level of ROS escapes the antioxidant defense, they primarily attack PUFA, to initiate chain reactions resulting in lipid peroxidation (LPO). Superoxide (O2 ∙−) is the major ROS generated in human spermatozoa . This electron-deficient product of O2 generates H2O2 via dismutation. These in turn undergo Fenton reaction to form ∙OH that is a potent initiator of chain reaction leading to LPO of membrane lipids. Therefore, may lead to sperm dysfunction due to loss of membrane fluidity [40]. Biopositive effect of ROS is seen at low considerations and is known to act selectively on the metabolism of prostanoids, in gene regulation or in the regulation of cellular growth , intracellular signaling, and in other types of signal transduction while excessive generation leads to cell death [44]. As described in the previous section, sperm chromatin is prone to oxidative damage leading to base modifications and DNA fragmentation. Damaged DNA has been observed in testicular, epididymal, and ejaculated human spermatozoa. Single-strand breaks are a direct result of oxidative damage on sperm DNA, while double-strand breaks may arise from exposure to 4-hydroxyl-2-nonenal a major product of LPO. Two types of DNA adducts, namely, 8-hydroxy-2-deoxyguanosine and two ethenonucleosides (1, N6-ethenoadenosine and 1, N6-ethenoguanosine) are found in human spermatozoa, both of which have been considered key biomarkers of DNA damage caused by oxidative stress [45].
Most of the DNA damages incurred to germ cell during spermatogenesis are taken care of by DNA repair systems. Any insult to such machinery may result in production of spermatozoa with damaged DNA which when fertilizes the ovum may impair embryo development.
DNA Repair Systems in Spermatozoa
An intriguing feature of chromatin remodeling is the introduction of DNA strand breaks concomitant with the general progression for the removal of nucleosomes that leaves the DNA with unconstrained supercoils. To reduce the torsional stress induced by an alteration in DNA topology, there occurs the transient appearance of DNA strand breaks to provide the swivel effect [36, 45]. It has been surmised that the integrity of the DNA condensing process plays a key role in the elimination of DNA strand breaks since the breaks are transient and are no longer detected at later steps of the spermiogenesis process where the nuclear protein transition is completed. Due to haploid nature of post-meiotic spermatids, DNA repair relies mostly on nonhomologous end joining. The basic nuclear proteins, i.e. the transition proteins and protamines would therefore act as “alignment factors” in nonhomologous end joining of the free ends of the DNA [36]. With the absence of DNA ligating activity in the DNA binding proteins it has been proposed that in elongating spermatids, topoisomerase II is responsible for generating as well as ligating DNA strand breaks that overlaps with the appearance of histone variant H2AX foci, a marker of DNA double-strand breaks [46]. H2AX in response to DSBs, acts by recruiting DNA repair factors to sites of DNA damage where it is rapidly phosphorylated resulting in formation of H2AX foci. Also, evidence suggests that ubiquitination and SUMOylation are involved in DNA repair pathways in elongating spermatids thus facilitating appropriate level of histone–protamine exchange [47]. However, a mature spermatozoon being transcriptionally and translationally inactive, the termination of DNA repair process occurs during its transit through the epididymis and post-ejaculation . Ultimately, the breaks in the DNA that may have escaped repair prior to compaction or damage occurring after the completion of chromatin remodeling are delivered to the oocyte. Oxidative stress impairs sperm DNA by introducing adducts such as ethenonucleosides that impair nucleotide excision repair in oocyte [48, 49]. Oocytes and early embryos have been shown to repair sperm DNA damage to some extent, so the biological effect of sperm DNA damage depends cumulatively on the magnitude of sperm chromatin damage and the capacity of the oocyte to repair it after fertilization.
From the above-mentioned facts, it is important to understand that proteins involved in chromatin remodeling and condensation are important for the repair of DNA in vivo. Under such circumstances, it is expected that targeted deletion of these respective genes or any alteration in their sequences would lead to the persistence of strand breaks up to much later stages of spermiogenesis or even in a matured spermatozoa. In this context, targeted deletion of mouse TP1 or TP2 gene have been compensated with a marked increase in the expression of other genes so that their function is apparently reduced. As a consequence of these mutations, it did not result in major sperm head abnormalities although alterations in the condensation state of the nuclei were nevertheless observed in both cases [50].
Gene Deletion and Sperm DNA Integrity
Y-Chromosome harbors several genes critical for spermatogenesis and development of gonads. Extensive research has been performed on the association of Y-chromosome deletions with male infertility. The Y-chromosome locus is divided into three regions; the proximal, middle, and distal of Yq11 and is labeled as AZF-a, b, and c after the azoospermia factor AZF . Patients with microdeletions in the AZFa region have been reported with congenital oligozoospermia or partial spermatogenic arrest, whereas patients with AZFb and AZFc are usually reported with azoospermia or oligozoospermia [51]. However, microdeletions in the overlapping area between the latter two regions may present with range of sperm counts (azoospermia to normal sperm count) [52].
Apoptosis
As the male germ cell development progress, apoptosis orchestrates the production and function of these cells from the early stages of gonadal differentiation to the moment of fertilization. It has been suggested that an early apoptotic pathway is initiated in spermatogonia and spermatocytes which express Fas. On the other hand, Sertoli cells express Fas ligand which upon binding to Fas on germ cells leads to death of the later. This mechanism enables Sertoli cells to limit the population of germ cells that adhere to it for support. Infact, apoptosis plays a significant role in the regulation of germ cell development by removing damaged cell in the seminiferous tubules and thus, safeguarding the genome integrity [42]. However, absence of timely repair or DNA damage if tolerated, the cells that harbor the damage are removed by the apoptosis pathway. Sperm DNA damage and impaired fertilization has often been correlated with unsuccessful apoptosis in the germ cell. The presence of apoptosis in ejaculated spermatozoa could be the result of various types of injuries . Testicular causes including hormonal depletion, irradiation, toxic agents, chemicals, and heat have been shown to induce apoptosis, while those in the epididymis are signals released by abnormal or defective spermatozoa or leukocytes, such as ROS and other mediators of inflammation/infection [53]. Irrespective of source or origin, the fertilization capacity of apoptotic sperm has been observed at the same rate as intact spermatozoa. However, the in vitro embryo development to the blastocyst stage is closely related to the integrity of the DNA. As a result of oxidative stress, the human ejaculate expresses various apoptotic markers that initiate apoptosis, some of which include Fas, phosphatidylserine (PS), Bcl-Xl, and p53. This apoptotic pathway in turn induces release of cytochrome c from mitochondrial membranes that triggers caspases, such as caspases 3 and 9, and annexin-V binding (Annexins are calcium-dependent phospholipid-binding proteins, which bind to PS). This pathway eventually leads to sperm apoptosis [54, 55]. It has been observed that mature spermatozoa from infertile patients with increased ROS levels had significantly higher levels of apoptosis than mature spermatozoa from the control group [40]. More dramatically, they may pose the risk of carrying a damaged genome into the egg, resulting in poor embryo development, miscarriage, or birth defects.
Ubiquitination
In response to DNA damage, cells activate a highly conserved signaling network, commonly referred to as the DNA damage response (DDR) , to safeguard genomic integrity. It is well understood that chromatin reorganization not only facilitates the compaction of the paternal genome into the sperm head but also protect the DNA from damaging agents. The DDR consists of a set of tightly regulated events, including detection of DNA damage, accumulation of DNA repair factors at the site of damage, and finally physical repair of the lesion. One of the process by which DNA damage is detected is histone ubiquitination. H2A and H2B ubiquitination are known to be enriched at sites of DNA damage albeit the primary function of histone ubiquitination is suggested to be sex chromosome inactivation during meiotic prophase and nucleosome removal at post meiotic stages [56–58]. RNF8 is a 485-residue nuclear polypeptide that is known to have ubiquitin E3 ligase activity. Upon DNA damage, RNF8 has been shown to be rapidly recruited to sites of DNA damage via its interaction with ϒH2AX. At sites of DNA damage, RNF8 ubiquitinates histones—H2A and H2B, promoting the recruitment of downstream DNA damage response factors such as 53BP1, BRCA1, and Rad51 [59]. However, more recently it has been reported that H4K8 acetylation and H4K16 acetylation are not affected in elongating spermatids of RNF8-deficient mice leaving this issue unresolved [60]. Ubiquitin is one of the 200 major proteins secreted in apocrine fashion by the epididymal epithelium which has the property of binding covalently to other proteins, via an isopeptide bond between the C-terminal glycine of ubiquitin and the E-amino group of a lysine, in substrate proteins [61]. Upon overwhelming damage, the DDR provokes detrimental cellular actions by involving the apoptotic machinery and inducing a coordinated demise of the damaged cells. Moreover, recent observations highlighted the role of ubiquitination in orchestrating the DDR, providing a dynamic cellular regulatory circuit helping to guarantee genomic stability and cellular homeostasis. Abnormal spermatozoa produced as a result of endogenous or exogenous insult become ubiquitinated and subsequently phagocytised by epididymal epithelial cells [62]. Despite this safety mechanism available during the epidiymal transit of the spermatozoa, some of the ubiquitinated spermatozoa are believed to escape phagocytosis and find their way into ejaculate [62, 63] and cause defects in head and axoneme. Protein ubiquitination typically occurs in the cell cytosol or nucleus and it has been postulated that sperm-acrosomal ubiquitin C-terminal hydrolases are involved in sperm-ZP interactions and antipolyspermy defense. In the studies of Sutovsky et al. in bovine semen, ubiquitination have been associated with DNA fragmentation. It is suggested that sperm ubiquitination is associated with poor-quality sperm parameters in men. In contradiction to the above, studies have revealed that ubiquitination is involved in the fertilization process, and once the process of ubiquitin–proteasome is inhibited, the percentage of fertilization is reduced [64]. Apart from DDR, protein ubiquitination has also been detected in several regions of human sperm and is initially inversely related to semen quality [63] while other studies have suggested that ubiquitination also plays a role in normal sperm function [63, 65–67]. During spermatogenesis, ubiquitination is a crucial process that is responsible for the replacement of the spermatid’s nuclear histones by transition proteins, followed by permanent substitution with protamines [53]. Ubiquitination also has a principal role in the dramatic reduction of human sperm centrosome that occurs during spermatid elongation. Thus, ubiquitination, principally a death signal for proteins is involved in maintaining the protein homeostasis in the spermatozoa, however, beyond threshold level is associated with anomalies is sperm structure and function.
SUMOylation
One of the critical phenomena for preserving genome integrity is the sheltering of chromosomal ends from unwanted DNA repair reactions and maintenance of telomere length homeostasis. A growing body of evidence suggests that covalent protein modification (SUMOylation) by SUMO (small ubiquitin-like modifier) to be critical in the regulation of numerous DNA transactions , including DNA repair and transcription, as well as heterochromatin formation and maintenance. These SUMO proteins exist in three isoforms: 1, 2, and 3. Of the three isoforms, SUMO2 and 3 are 95 % identical and are often referred to as SUMO2/3 [68–70]. Amongst the many targeted PTMs, SUMOylation have not been identified as a potential target although such targets are critical for understanding the role of SUMO in normal and impaired sperm function. SUMO proteins have been localized to different subcompartments of mouse and human testicular cells [71–73] while SUMO1 is localized mostly to the heads of human sperm [74]. A recent study by Vigodner et al. demonstrated the localization and identification of SUMOylated proteins in the defective spermatozoa by immunofluorescence and electron microscopy. They revealed that SUMO proteins were highly expressed in the neck area of human sperm and were also detectable in the flagella and head regions . High levels of SUMOylation were detected in defective spermatozoa in the neck and tail region relative to normal spermatozoa [75]. The study concluded that numerous proteins are modified by SUMOylation in human spermatozoa; wherein excessive SUMOylation is a marker of defective spermatozoa.
Sperm DNA and Recurrent Pregnancy Loss
Past research have focused on the contributions of the fertilizing spermatozoon to the oocyte and have limited their observation to spermatozoon by being a carrier or vector that transfers the DNA to the egg. DNA in the male germ cell is tightly compacted and is considered evolutionarily as a highly conserved process. Owing, to this silent state of the compacted nucleus, it was long thought spermatozoon transcripts did not play a role in embryo development and that only maternal transcripts were involved. It is now well established that apart from being a mere cargo for the delivery of DNA, spermatozoa transmit information to the next generation via the genome as well as the epigenome . Several recent studies however suggest that this tight packaging of the sperm chromatin conveys important epigenetic message to the embryo . The possible mechanism underlying this phenomenon is the extensive cross-talk between the fertilizing spermatozoa and the oocyte which leads to activation of the egg on one hand and sperm head decondensation on the other. This is extremely important to understand in the light of RPL, as here the problem is not the inability to impregnate or conceive but rather the limitation in carrying the conceptus to a live birth. The male gamete confers 50 % of the genomic material on the embryo, and also contributes to placental and embryonic development [76]. Genetic and epigenetic alterations of sperm may therefore have dire consequences in early pregnancy loss. In this context, it is noteworthy to state that while genetic inheritance is based on the DNA code, epigenetic information comprises modifications occurring directly on DNA or on the chromatin. The major type of DNA modification is methylation, whereas on the chromatin various modifications occur on specific residues of histones, including methylation, phosphorylation, acetylation, and ubiquitination. The mammalian genome undergoes two major phases of epigenetic reprogramming, once in the primordial germ cells and once in the preimplantation embryos. After the crucial process of fertilization, protamines in sperm chromatin are rapidly replaced with histones which are hyperacetylated, while the male pronucleus DNA undergoes demethylation in the absence of DNA replication. Therefore, it is speculated that any alteration of chromatin structure is an important mechanism for regulating DNA transcription.
DNA Damage and Sperm Function
As the paternal genome is inactive transcriptionally till 2 days after fertilization, a damaged sperm DNA does not impair fertilization or cleavage [77]. However, activation of paternal genome with modifications at the level of the DNA nucleotides and/or DNA strand breaks that are beyond the oocyte repair capacity after fertilization are not compatible with normal embryo and fetal development. This raises the question as to whether the retention of paternal gene sequences having some potentially important embryological function in a more relaxed chromatin configuration is more susceptible to DNA damage than the bulk, protamine-packaged DNA [53]. Since, abnormal paternal genome modifications lead to poor blastocyst development, unequal cleavage, implantation failure, or early fetal loss. As mentioned earlier, small DNA damages in spermatozoa as result of endo-or exogenous insults are repaired by pre- and postreplication repair mechanisms, but large DNA damages cannot be repaired. Decreased elimination and subsequent accumulation of the DNA-damaged spermatozoa results in poor-quality sperm. This is due largely to inefficient apoptotic machinery and poor DNA integrity and abnormal chromatin packaging [78]. Sperm chromatin packaging anomalies are closely associated with poor fertility outcomes and higher levels of DNA damage are an accompanying feature of dysfunctional sperm [37]. Thus, men who are partners of couples with recurrent pregnancy loss may have sperms with normal morphology, whose, germ cells may harbor damaged DNA. This causes an alteration in sperm quality and function, sperm-oocyte interaction, implantation, and early embryo development all of which are good indicators of successful pregnancy.
Aneuploidy
As a subpopulation, men with normal semen parameters who are partners in a couple with RPL or unexplained recurrent IVF failure are commonly overlooked [79]. Elevated levels of sperm aneuploidies are known to be associated with RPL. Sperm aneuploidy has been defined as the abnormality in the number of chromosome resulting from defective meiosis during spermatogenesis. As a result, a spermatozoon that is disomic or nullisomic for a particular chromosome will develop. Arguably, delivery of an intact chromosome by the spermatozoon to the ovum is the most important function of sperm. Fertilization with such type of abnormal sperm cell results in monosomic or trisomic embryos, the majority of which are incompatible with a viable birth. It is unfortunate, that, with the available technologies we can have a gross identification of sperm with abnormal morphology than detecting the underlying genetic abnormality such as aneuploidy [79]. Carrell and his colleagues reported a significant increase in sperm chromosome aneuploidy, apoptosis, and abnormal sperm morphology in some patients with recurrent loss of pregnancy [80]. The incidence of aneuploidy increases as the standard semen parameters worsen. Even though men within fertility exhibit high rates of sperm aneuploidy genetic testing in clinics for men with infertility is restricted to detection of chromosomal abnormalities using a karyotype and Y-chromosome microdeletion analysis. It is important to realize that sperm aneuploidy rates can be high even in men with normal sperm morphology. Cytogenetic analysis of sperm using fluorescence in situ hybridization (FISH) provides a method to test for sperm chromosomal aneuploidy and can help evaluate potential causes of RPL or recurrent IVF failure [79] which has been detailed below. Recently, a study was undertaken in order to bring about an association between sperm aneuploidies and normal semen parameters in men who are partners of RPL using the cytogenetic technique FISH. The study showed that nearly was 40 % of men with RPL and normal sperm density/motility had abnormal sperm aneuploidy while no association was found between sperm DNA fragm entation and sperm aneuploidy [79].
DNA Fragmentation
Recent advances in the understanding of mammalian sperm chromatin and function have changed our perception of spermatozoa as a silent carrier of paternal DNA. However, there are several theories with relation to DNA strand breakage and subsequent DNA fragmentation that needs to be resolved. The question of whether DNA strand break occurs exclusively during spermiogenic compaction of chromatin and is not repaired in the seminiferous tubules , or rather is a result of aggressors acting on the male genital tract , is still unresolved [78]. To explain the origin of sperm DNA damage, several hypotheses have been proposed. DNA strand separation is a physiological process that accompanies the recombination step that occurs at the time of protamine-ordained compaction. DNA fragmentation is often a result of double or single-strand breaks which have been induced in the DNA prior to, or post, ejaculation of the spermatozoa. The causes of sperm DNA fragmentation are still unclear, even if apoptosis, oxidative assault, and defects in chromatin maturation are hypothesized. Several studies have reported a significant negative association between the percentage of sperm with DNA fragmentation and fertilization rate in the context of RPL. A recent meta-analysis including 16 studies found a highly significant increase in miscarriage rate in couples where the male partner had elevated levels of sperm DNA damage compared to those where the male partner had low levels of sperm DNA damage (risk ratio = 2.16, P < 0.00001) [81]. In another study comparing fertile sperm donors with couples who have unexplained recurrent miscarriage, showed that 85 % of the couples affected with recurrent miscarriage had a profile with high values of double-stranded DNA damage compared to only 33 % among fertile sperm donors , suggesting a specific paternal explanation in these otherwise unexplained cases [82]. Several mechanisms have been proposed in explanation to the genesis of sperm DNA fragmentation but none have completely clarified the causes and site of origin of sperm DNA fragmentation and our knowledge is still limited to hypothesis and theories. Amongst the many proposed mechanism, one theory states that DNA nicks, as a part of the remodeling process of sperm chromatin are produced which are not completely repaired due to an impairment of sperm maturation process. Abortive apoptosis can be associated with DNA cleavage and can also be provoked by the attack of free radicals including ROS, acting both in testis and in post-testicular sites. The major DNA adducts found 8-hydroxy-2-deoxyguanosine and two ethenonucleosides (1, N6-ethenoadenosine and 1, N6-ethenoguanosine) are found in human sperm DNA which have been considered key biomarkers of DNA damage caused by OS [40]. Many direct and indirect studies prove the aforementioned hypothesis by stating that increased levels of sperm DNA fragmentation show high degree of cell immaturity , apoptosis , or oxidative stress. Whatever the case may be, it is important to understand that a damaged DNA does not inhibit fertilization or prevent the formation of a zygote rather prohibits further development of the embryo beyond the two-celled stage. It is therefore, pertinent to understand other underlying causes such as alterations in the epigenetic programming of the sperm chromatin that may have a profound effect on sperm DNA.
DNA Methylation
DNA compaction in male germ cells is a fundamental biologic process and a prerequisite for transmission of the male genome to the next generation. Several studies suggest that this sperm-specific genome packaging structure conveys an important epigenetic message to the embryo [22]. Epigenetic programming in the spermatozoa is unique with as erasure of epigenetic marks occurs in primordial germ followed by its establishment during post-meiotic maturation. Therefore, understanding the epigenetic processes in the male germ cells may contribute to understanding paternal effects on early embryonic development. DNA methylation as one of the most studied epigenetic markers of male germ cells refers to the addition of a methyl group from S-adenosyl-methionine to the fifth position of the cytosine ring (5meC) in CpG dinucleotides. Nearly, 3–5 % of the cytosine residues in mammalian genomic DNA appear in the form of 5meC [83]. The process of methylation in germ cells is unique and necessary for proper spermatogenesis and sperm production. Three structurally distinct chromatin configurations may occur as follows: histone-packaged hypomethylated DNA, histone-packaged methylated DNA, and protamine-packaged hypomethylated DNA [27, 84]. It is observed that only four imprinted loci are methylated in the male germ line which includes Igf2/H19, Rasgrf1, Dlk1-Gtl2, and Zdbf2. A specific family of methyltansferases (DNMTs) is also involved in DNA methylation. DNMT1 ensures methylation maintenance while DNMT3A, 3B, and 3L specifically allow the methylation process in germ cells. DNMT3A and 3B have a catalytic activity, whereas DNMT3L lacks this catalytic activity and acts as a cofactor to DNMT3A [85, 86]. After fertilization, the decondensation of the male pronucleus follows an epigenetic reprogramming with DNA demethylation along with protamine-to-histone transition, and histone modifications that contributes to genome activation in the embryo and subsequent embryonic development. But, there still remain many facts unclear with regard to mechanism and function of paternal genome. Post-fertilization, histones incorporated into the male pronucleus are highly acetylated, however, immediately upon histone incorporation; H3K4me1, H3K9me1, and H3K27me1 are detectable which is at a time when DNA methylation is still present in the male pronucleus. Although these findings raise the question that whether any of these epigenetic marks in the paternal pronucleus protect specific regions such as the centromeres from demethylation remains known. These suggest that the sperm genome may be packaged and poised for two programs: first is a reminiscent gametogenesis program (active chromatin marks), and second is a future embryonic program (bivalent domains) [34]. Nevertheless, the progressive histone modification and concomitant demethylation in the paternal pronucleus presumably leads to a chromatin state easily accessible by the maternal genome. However, this does not devoid the paternal genome from acquiring unique epigenetic marks early on in development which are important for imprinting and X chromosome inactivation [87]. Furthermore, the dynamics of DNA methylation and histone modifications during epigenetic reprogramming raise several questions about additional mechanistic links. For example, specific sequences in the male pronucleus escape demethylation which generates special interest. Secondly, is there sequence preference for demethylation or are there regions in the sperm genome that contain histones rather than protamines, perhaps carrying particular modifications? Are the stepwise histone modifications of the paternal genome marks for later de novo methylation events? In an answer to these mechanistic links, it is possible that during reprogramming these appear to be developmentally regulated and may depend on the precise developmental stage, cell type, and genomic region. This may account for developmental plasticity and regulation, which is typical of pluripotent cell types. The fact that there are so many open questions means that this is an area with exciting discoveries ahead of us.
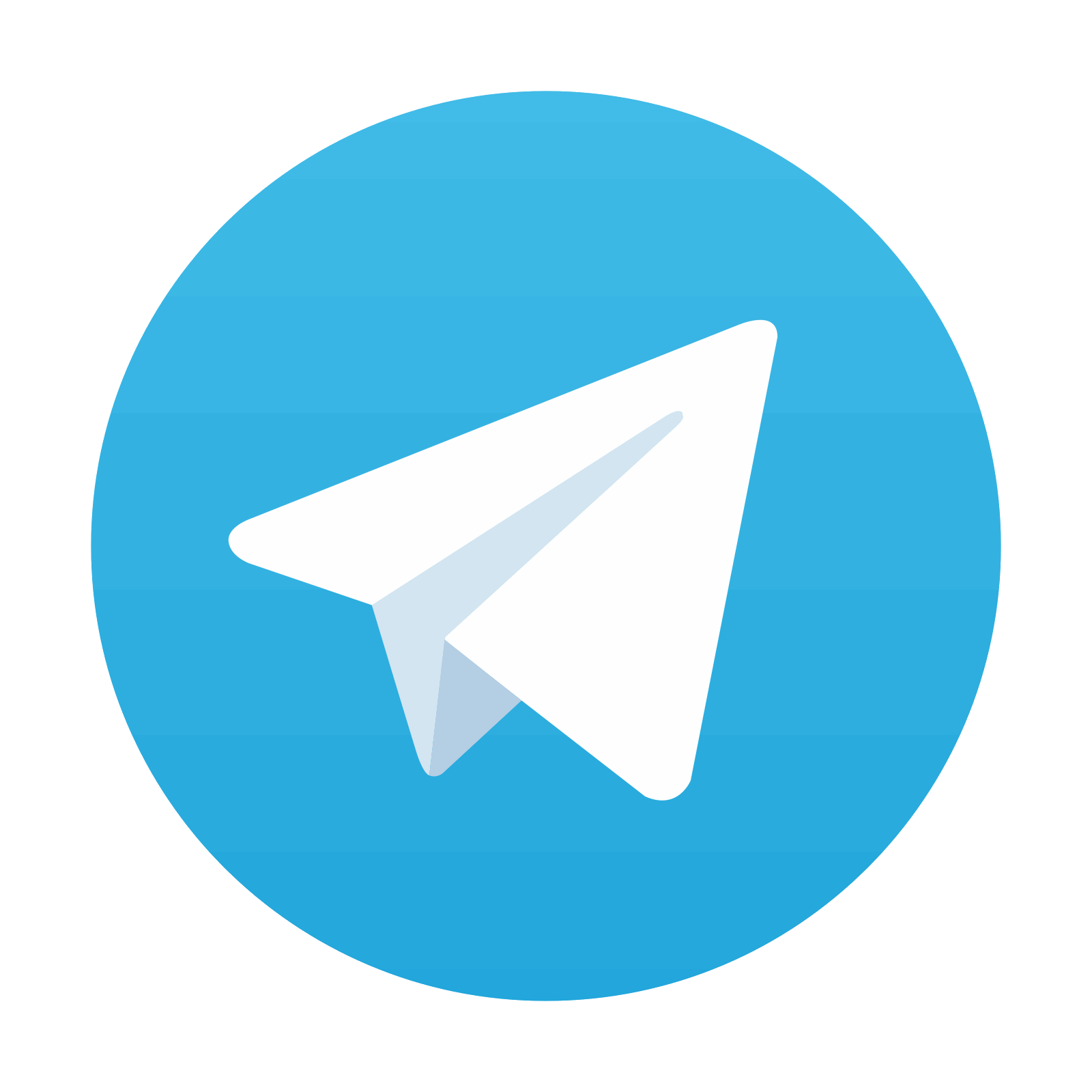
Stay updated, free articles. Join our Telegram channel

Full access? Get Clinical Tree
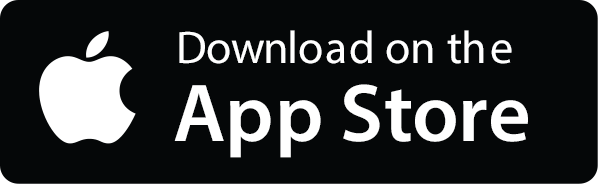
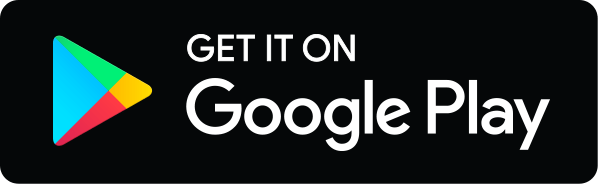